Diagnosis of Hypertensive Disorders
Hypertension is diagnosed empirically when appropriately taken blood pressure exceeds 140 mm Hg systolic or 90 mm Hg diastolic. Korotkoff phase V is used to define diastolic pressure. Previously, incremental increases of 30 mm Hg systolic or 15 mm Hg diastolic from midpregnancy blood pressure values had also been used as diagnostic criteria, even when absolute values were < 140/90 mm Hg. These incremental changes are no longer recommended criteria because evidence shows that such women are not likely to experience increased adverse pregnancy outcomes (Levine, 2000; North, 1999). That said, women who have a rise in pressure of 30 mm Hg systolic or 15 mm Hg diastolic should be observed more closely because eclamptic seizures develop in some of these women whose blood pressures have stayed < 140/90 mm Hg (Alexander, 2006). A sudden rise in mean arterial pressure later in pregnancy—also known as “delta hypertension”—may also signify preeclampsia even if blood pressure is < 140/90 mm Hg (Macdonald-Wallis, 2012; Vollaard, 2007).
Concept of “Delta Hypertension”
The systolic and diastolic blood pressure levels of 140/90 mm Hg have been arbitrarily used since the 1950s to define “hypertension” in nonpregnant individuals. As discussed in detail in Chapter 50 (p. 1000), these levels were selected by insurance companies for a group of middle-aged men. It seems more realistic to define normal-range blood pressures that fall between an upper and lower limit for blood pressure measurements for a particular population—such as young, healthy, pregnant women. A schematic example is shown in Figure 40-1 using arbitrary blood pressure readings. Data curves for both women show blood pressure measurements near the 25th percentile until 32 weeks. These begin to rise in patient B, who by term has substantively increased blood pressures. However, her pressures are still < 140/90 mm Hg, and thus she is considered to be “normotensive.” We termed this rather acute increase in blood pressure as “delta hypertension.” As discussed later, some of these women will develop eclamptic seizures or HELLP (hemolysis, elevated liver enzyme levels, low platelet count) syndrome while still normotensive.
FIGURE 40-1 Schematic shows normal reference ranges for blood pressure changes across pregnancy. Patient A (blue) has blood pressures near the 20th percentile throughout pregnancy. Patient B (red) has a similar pattern with pressures at about the 25th percentile until about 36 weeks when blood pressure begins to increase. By term, it is substantively higher and in the 75th percentile, but she is still considered “normotensive.”
Gestational Hypertension
This diagnosis is made in women whose blood pressures reach 140/90 mm Hg or greater for the first time after midpregnancy, but in whom proteinuria is not identified (Table 40-1). Almost half of these women subsequently develop preeclampsia syndrome, which includes findings such as headaches or epigastric pain, proteinuria, and thrombocytopenia. Even so, when blood pressure increases appreciably, it is dangerous to both mother and fetus to ignore this rise only because proteinuria has not yet developed. As Chesley (1985) emphasized, 10 percent of eclamptic seizures develop before overt proteinuria can be detected. Finally, gestational hypertension is reclassified by some as transient hypertension if evidence for preeclampsia does not develop and the blood pressure returns to normal by 12 weeks postpartum.
TABLE 40-1. Diagnostic Criteria for Pregnancy-Associated Hypertension
Preeclampsia Syndrome
As emphasized throughout this chapter, preeclampsia is best described as a pregnancy-specific syndrome that can affect virtually every organ system. And, although preeclampsia is much more than simply gestational hypertension with proteinuria, appearance of proteinuria remains an important diagnostic criterion. Thus, proteinuria is an objective marker and reflects the system-wide endothelial leak, which characterizes the preeclampsia syndrome.
Abnormal protein excretion is arbitrarily defined by 24-hour urinary excretion exceeding 300 mg; a urine protein:creatinine ratio ≥ 0.3; or persistent 30 mg/dL (1+ dipstick) protein in random urine samples (Lindheimer, 2008a). None of these values is sacrosanct, and urine concentrations vary widely during the day, as do dipstick readings. Thus, assessment may show a dipstick value of 1+ to 2+ from concentrated urine specimens from women who excrete < 300 mg/day. As discussed on page 740, it is likely that determination of a spot urine:creatinine ratio is a suitable replacement for a 24-hour urine measurement (Chap. 4, p. 65).
It is now appreciated that overt proteinuria may not be a feature in some women with the preeclampsia syndrome (Sibai, 2009). Because of this, the Task Force (2013) suggested other diagnostic criteria, which are shown in Table 40-1. Evidence of multiorgan involvement may include thrombocytopenia, renal dysfunction, hepatocellular necrosis (“liver dysfunction”), central nervous system perturbations, or pulmonary edema.
Indicators of Preeclampsia Severity
The markers listed in Table 40-1 are also used to classify preeclampsia syndrome severity. Although many use a dichotomous “mild” and “severe” classification, the Task Force (2013) discourages the use of “mild preeclampsia.” It is problematic that there are criteria for the diagnosis of “severe” preeclampsia, but the default classification is either implied or specifically termed as “mild,” “less severe,” or “nonsevere” (Alexander, 2003; Lindheimer, 2008b). There are no generally agreed-on criteria for “moderate” preeclampsia—an elusive third category. We use the criteria listed in Table 40-2, which are categorized as “severe” versus “nonsevere.” Importantly, while it is pragmatic that nonsevere classifications include “moderate” and “mild,” these have not been specifically defined.
TABLE 40-2. Indicators of Severity of Gestational Hypertensive Disordersa
Some symptoms are considered to be ominous. Headaches or visual disturbances such as scotomata can be premonitory symptoms of eclampsia. Epigastric or right upper quadrant pain frequently accompanies hepatocellular necrosis, ischemia, and edema that ostensibly stretches Glisson capsule. This characteristic pain is frequently accompanied by elevated serum hepatic transaminase levels. Finally, thrombocytopenia is also characteristic of worsening preeclampsia as it signifies platelet activation and aggregation as well as microangiopathic hemolysis. Other factors indicative of severe preeclampsia include renal or cardiac involvement and obvious fetal-growth restriction, which also attests to its duration.
As will be discussed, the more profound these signs and symptoms, the less likely they can be temporized, and the more likely delivery will be required. A caveat is that differentiation between nonsevere and severe gestational hypertension or preeclampsia can be misleading because what might be apparently mild disease may progress rapidly to severe disease.
Eclampsia
In a woman with preeclampsia, a convulsion that cannot be attributed to another cause is termed eclampsia. The seizures are generalized and may appear before, during, or after labor. The proportion who do not develop seizures until after 48 hours postpartum approximates 10 percent (Sibai, 2005). In some reports, up to a fourth of eclamptic seizures develop beyond 48 hours postpartum (Chames, 2002). Our experiences from Parkland Hospital, however, are that delayed postpartum eclampsia continues to occur in about 10 percent of cases—similar to what we first reported more than 20 years ago (Alexander, 2006; Brown, 1987). This lower percentage was also found in 222 women with eclampsia from The Netherlands (Zwart, 2008).
Preeclampsia Superimposed on Chronic Hypertension
Regardless of its cause, any chronic hypertensive disorder predisposes a woman to develop superimposed preeclampsia syndrome. Chronic underlying hypertension is diagnosed in women with documented blood pressures ≥ 140/90 mm Hg before pregnancy or before 20 weeks’ gestation, or both. Hypertensive disorders can create difficult problems with diagnosis and management in women who are not first seen until after midpregnancy. This is because blood pressure normally decreases during the second and early third trimesters in both normotensive and chronically hypertensive women (Chap. 50, p. 1003). Thus, a woman with previously undiagnosed chronic vascular disease who is seen before 20 weeks frequently has blood pressures within the normal range. During the third trimester, however, as blood pressures return to their originally hypertensive levels, it may be difficult to determine whether hypertension is chronic or induced by pregnancy. Even a careful search for evidence of preexisting end-organ damage may be futile, as many of these women have mild disease and no evidence of ventricular hypertrophy, retinal vascular changes, or renal dysfunction.
In some women with chronic hypertension, their blood pressure increases to obviously abnormal levels, and this is typically after 24 weeks. If new-onset or worsening baseline hypertension is accompanied by new-onset proteinuria or other findings listed in Table 40-1, then superimposed preeclampsia is diagnosed. Compared with “pure” preeclampsia, superimposed preeclampsia commonly develops earlier in pregnancy. It also tends to be more severe and often is accompanied by fetal-growth restriction. The same criteria shown in Table 40-2 are also used to further characterize severity of superimposed preeclampsia.
INCIDENCE AND RISK FACTORS
Preeclampsia Syndrome
Young and nulliparous women are particularly vulnerable to developing preeclampsia, whereas older women are at greater risk for chronic hypertension with superimposed preeclampsia. The incidence is markedly influenced by race and ethnicity—and thus by genetic predisposition. By way of example, in nearly 2400 nulliparas enrolled in a Maternal-Fetal Medicine Units (MFMU) Network study, the incidence of preeclampsia was 5 percent in white, 9 percent in Hispanic, and 11 percent in African-American women (Myatt, 2012a,b).
Other factors include environmental, socioeconomic, and even seasonal influences (Lawlor, 2005; Palmer, 1999; Spencer, 2009). With consideration for these vicissitudes, in several worldwide studies reviewed by Staff and coworkers (2014), the incidence of preeclampsia in nulliparous populations ranged from 3 to 10 percent. The incidence of preeclampsia in multiparas is also variable but is less than that for nulliparas. Specifically, population studies from Australia, Canada, Denmark, Norway, Scotland, Sweden, and Massachusetts indicate an incidence of 1.4 to 4 percent (Roberts, 2011).
There are several other risk factors associated with preeclampsia. These include obesity, multifetal gestation, maternal age, hyperhomocysteinemia, and metabolic syndrome (Conde-Agudelo, 2000; Scholten, 2013; Walker, 2000). The relationship between maternal weight and the risk of preeclampsia is progressive. It increases from 4.3 percent for women with a body mass index (BMI) < 20 kg/m2 to 13.3 percent in those with a BMI > 35 kg/m2 (Fig. 48-5, p. 966). In women with a twin gestation compared with those with singletons, the incidence of gestational hypertension—13 versus 6 percent, and the incidence of preeclampsia—13 versus 5 percent, are both significantly increased (Sibai, 2000). It is interesting that this latter incidence is unrelated to zygosity (Maxwell, 2001).
Although smoking during pregnancy causes various adverse pregnancy outcomes, ironically, it has consistently been associated with a reduced risk for hypertension during pregnancy (Bainbridge, 2005; Zhang, 1999). Kraus and associates (2013) posit that this is because smoking upregulates placental adrenomedullin expression, which regulates volume homeostasis.
Women with preeclampsia in the first pregnancy are at greater risk in a second pregnancy compared with women normotensive during their first pregnancy (McDonald, 2009). And conversely, in the woman who was normotensive during her first pregnancy, the incidence of preeclampsia in a subsequent pregnancy is much lower than for a first pregnancy. In a population-based retrospective cohort analysis, Getahun and colleagues (2007) studied almost 137,000 second pregnancies in such women. The incidence for preeclampsia in secundigravida white women was 1.8 percent compared with 3 percent in African-American women.
Eclampsia
Presumably because it is somewhat preventable by adequate prenatal care, the incidence of eclampsia has decreased over the years in areas where health care is more readily available. In countries with adequate resources, its incidence averages 1 in 2000 deliveries. Ventura and associates (2000) estimated that the incidence in the United States in 1998 was 1 in 3250 births. According to the Royal College of Obstetricians and Gynaecologists (2006), it approximates 1 in 2000 in the United Kingdom. Akkawi and coworkers (2009) reported it to be 1 in 2500 in Dublin, Andersgaard and associates (2006) as 1 per 2000 for Scandinavia, and Zwart and colleagues (2008) as 1 per 1600 for The Netherlands.
ETIOPATHOGENESIS
Any satisfactory theory concerning the etiology and pathogenesis of preeclampsia must account for the observation that gestational hypertensive disorders are more likely to develop in women with the following characteristics:
• Are exposed to chorionic villi for the first time
• Are exposed to a superabundance of chorionic villi, as with twins or hydatidiform mole
• Have preexisting conditions of endothelial cell activation or inflammation such as diabetes or renal or cardiovascular disease
• Are genetically predisposed to hypertension developing during pregnancy.
A fetus is not a requisite for preeclampsia to develop. And, although chorionic villi are essential, they need not be intrauterine. For example, Worley and associates (2008) reported a 30-percent incidence in women with an extrauterine pregnancy exceeding 18 weeks’ gestation. Regardless of precipitating etiology, the cascade of events leading to the preeclampsia syndrome is characterized by abnormalities that result in vascular endothelial damage with resultant vasospasm, transudation of plasma, and ischemic and thrombotic sequelae.
Phenotypic Expression of Preeclampsia Syndrome
The preeclampsia syndrome is widely variable in its clinical phenotypic expression. There are at least two major subtypes differentiated by whether or not remodeling of uterine spiral arterioles by endovascular trophoblastic invasion is defective. This concept has given rise to the “two-stage disorder” theory of preeclampsia etiopathogenesis. Ness and Roberts (1996) consider that the two-stage disorder includes “maternal and placental preeclampsia.” According to Redman and coworkers (2014), stage 1 is caused by faulty endovascular trophoblastic remodeling that downstream causes the stage 2 clinical syndrome. Importantly, stage 2 is susceptible to modification by preexisting maternal conditions that are manifest by endothelial cell activation or inflammation. Such conditions include cardiovascular or renal disease, diabetes, obesity, immunological disorders, or hereditary influences.
Such compartmentalization is artificial, and it seems logical to us that preeclampsia syndrome presents clinically as a spectrum of worsening disease. Moreover, evidence is accruing that many “isoforms” exist as discussed below. Examples include differences in maternal and fetal characteristics, placental findings, and hemodynamic findings (Phillips, 2010; Valensise, 2008; van der Merwe, 2010).
Etiology
Writings describing eclampsia have been traced as far back as 2200 BC (Lindheimer, 2014). And, an imposing number of mechanisms have been proposed to explain its cause. Those currently considered important include:
1. Placental implantation with abnormal trophoblastic invasion of uterine vessels
2. Immunological maladaptive tolerance between maternal, paternal (placental), and fetal tissues
3. Maternal maladaptation to cardiovascular or inflammatory changes of normal pregnancy
4. Genetic factors including inherited predisposing genes and epigenetic influences.
Abnormal Trophoblastic Invasion
Normal implantation is characterized by extensive remodeling of the spiral arterioles within the decidua basalis as shown schematically in Figure 40-2 (Chap. 5, p. 93). Endovascular trophoblasts replace the vascular endothelial and muscular linings to enlarge the vessel diameter. The veins are invaded only superficially. In some cases of preeclampsia, however, there may be incomplete trophoblastic invasion. With this, decidual vessels, but not myometrial vessels, become lined with endovascular trophoblasts. The deeper myometrial arterioles do not lose their endothelial lining and musculoelastic tissue, and their mean external diameter is only half that of corresponding vessels in normal placentas (Fisher, 2014). In general, the magnitude of defective trophoblastic invasion is thought to correlate with severity of the hypertensive disorder (Madazli, 2000).
FIGURE 40-2 Schematic representation of normal placental implantation shows proliferation of extravillous trophoblasts from an anchoring villus. These trophoblasts invade the decidua and extend into the walls of the spiral arteriole to replace the endothelium and muscular wall to create a dilated low-resistance vessel. With preeclampsia, there is defective implantation characterized by incomplete invasion of the spiral arteriolar wall by extravillous trophoblasts. This results in a small-caliber vessel with high resistance to flow.
Using electron microscopy, De Wolf and coworkers (1980) examined arteries taken from the implantation site. They reported that early preeclamptic changes included endothelial damage, insudation of plasma constituents into vessel walls, proliferation of myointimal cells, and medial necrosis. Lipid accumulated first in myointimal cells and then within macrophages. These lipid-laden cell changes, shown in Figure 40-3, were referred to as atherosis by Hertig (1945). Nelson and colleagues (2014) completed placental examination in more than 1200 women with preeclampsia. These investigators reported that vascular lesions including spiral arteriole narrowing, atherosis, and infarcts were more common in placentas from women diagnosed with preeclampsia before 34 weeks.
FIGURE 40-3 Atherosis in a blood vessel from a placental bed. A. Photomicrograph shows disruption of the endothelium that results in a narrowed lumen because of subendothelial accumulation of plasma proteins and foamy macrophages. Some of the foamy macrophages are shown by curved arrows, and straight arrows highlight areas of endothelial disruption. B. Schematic diagram of the photomicrograph. (Modified from Rogers, 1999, with permission.)
Thus, the abnormally narrow spiral arteriolar lumen likely impairs placental blood flow. McMahon and colleagues (2014) have provided evidence that decreased soluble antiangiogenic growth factors may be involved in faulty endovascular remodeling. Diminished perfusion and a hypoxic environment eventually lead to release of placental debris or microparticles that incite a systemic inflammatory response (Lee, 2012; Redman, 2012). Fisher and Roberts (2014) have provided an elegant review of the molecular mechanisms involved in these interactions.
Defective placentation is posited to further cause the susceptible (pregnant) woman to develop gestational hypertension, the preeclampsia syndrome, preterm delivery, a growth-restricted fetus, and/or placental abruption (Brosens, 2011; Kovo, 2010; McElrath, 2008; Nelson, 2014). In addition, Staff and coworkers (2013) have hypothesized that acute atherosis identifies a group of women at increased risk for later atherosclerosis and cardiovascular disease.
Immunological Factors
Maternal immune tolerance to paternally derived placental and fetal antigens is discussed in Chapter 5 (p. 97). Loss of this tolerance, or perhaps its dysregulation, is another theory cited to account for preeclampsia syndrome (Erlebacher, 2013). Certainly, the histological changes at the maternal-placental interface are suggestive of acute graft rejection. Some of the factors possibly associated with dysregulation include “immunization” from a previous pregnancy, some inherited human leukocyte antigen (HLA) and natural killer (NK)-cell receptor haplotypes, and possibly shared susceptibility genes with diabetes and hypertension (Fukui, 2012; Ward, 2014).
Inferential data also suggest preeclampsia to be an immune-mediated disorder. For example, the risk of preeclampsia is appreciably enhanced in circumstances in which formation of blocking antibodies to placental antigenic sites might be impaired. In this scenario, the first pregnancy would carry a higher risk. Tolerance dysregulation might also explain an increased risk when the paternal antigenic load is increased, that is, with two sets of paternal chromosomes—a “double dose.” Namely, women with molar pregnancies have a high incidence of early-onset preeclampsia. Women with a trisomy 13 fetus also have a 30- to 40-percent incidence of preeclampsia. These women have elevated serum levels of antiangiogenic factors, and the gene for one of these factors, sFlt-1, is on chromosome 13 (Bdolah, 2006). Conversely, women previously exposed to paternal antigens, such as a prior pregnancy with the same partner, are “immunized” against preeclampsia. This phenomenon is not as apparent in women with a prior abortion. Strickland and associates (1986) studied more than 29,000 pregnancies at Parkland Hospital. These investigators reported that hypertensive disorder rates were decreased in women who previously had miscarried compared with nulligravidas. However, the difference, although statistically significant, was not great—22 versus 25 percent. Other studies have shown that multiparas impregnated by a new consort have an increased risk of preeclampsia (Mostello, 2002).
Redman and colleagues (2014) reviewed the possible role of immune maladaptation in preeclampsia pathophysiology. In women destined to be preeclamptic, extravillous trophoblasts early in pregnancy express reduced amounts of immunosuppressive nonclassic HLA G. Black women more commonly have the 1597ΔC allele that further predisposes to preeclampsia (Loisel, 2013). Zhou and coworkers (2012b) have shown this to be associated with high levels of placental oxidative products. These changes may contribute to defective placental vascularization in stage 1 of the preeclampsia syndrome (p. 732). Recall that as discussed in Chapter 4 (p. 56), during normal pregnancy, T-helper (Th) lymphocytes are produced so that type 2 activity is increased in relation to type 1—termed type 2 bias (Redman, 2012, 2014). Th2 cells promote humoral immunity, whereas Th1 cells stimulate inflammatory cytokine secretion. Beginning in the early second trimester in women who develop preeclampsia, Th1 action is increased and the Th1/Th2 ratio changes. Contributors to an enhanced immunologically mediated inflammatory reaction are stimulated by placental microparticles and by adipocytes (Redman, 2012, 2014).
Endothelial Cell Activation
In many ways, inflammatory changes are believed to be a continuation of the stage 1 changes caused by defective placentation as discussed above. In response to placental factors released by ischemic changes or by any other inciting cause, a cascade of events begins (Davidge, 2014). Thus, antiangiogenic and metabolic factors and other inflammatory mediators are thought to provoke endothelial cell injury.
Endothelial cell dysfunction may result from an extreme activated state of leukocytes in the maternal circulation (Faas, 2000; Gervasi, 2001). Briefly, cytokines such as tumor necrosis factor-α (TNF-α) and the interleukins (IL) may contribute to the oxidative stress associated with preeclampsia. This is characterized by reactive oxygen species and free radicals that lead to formation of self-propagating lipid peroxides (Manten, 2005). These in turn generate highly toxic radicals that injure endothelial cells, modify their nitric oxide production, and interfere with prostaglandin balance. Other consequences of oxidative stress include production of the lipid-laden macrophage foam cells seen in atherosis and shown in Figure 40-3; activation of microvascular coagulation manifest by thrombocytopenia; and increased capillary permeability manifest by edema and proteinuria.
These observations on the effects of oxidative stress in preeclampsia have given rise to increased interest in the potential benefit of antioxidants to prevent preeclampsia. Unfortunately, dietary supplementation with vitamins E (α-tocopherol) and C (ascorbic acid) to prevent preeclampsia has thus far proven unsuccessful (Task Force, 2013). This is discussed subsequently.
Nutritional Factors
John and colleagues (2002) showed that in the general population, a diet high in fruits and vegetables with antioxidant activity is associated with decreased blood pressure. Zhang and associates (2002) reported that the incidence of preeclampsia was doubled in women whose daily intake of ascorbic acid was less than 85 mg. These studies were followed by randomized trials to study dietary supplementation. Villar and coworkers (2006) showed that calcium supplementation in populations with a low dietary calcium intake had a small effect to lower perinatal mortality rates but no effect on preeclampsia incidence (p. 748). According to the 2013 Task Force, in several trials, supplementation with the antioxidant vitamins C or E showed no benefits.
Genetic Factors
From a hereditary viewpoint, preeclampsia is a multifactorial, polygenic disorder. In their comprehensive review, Ward and Taylor (2014) cite an incident risk for preeclampsia of 20 to 40 percent for daughters of preeclamptic mothers; 11 to 37 percent for sisters of preeclamptic women; and 22 to 47 percent for twins. In a study by Nilsson and colleagues (2004) that included almost 1.2 million Swedish births, there was a genetic component for gestational hypertension and for preeclampsia. They also reported 60-percent concordance in monozygotic female twin pairs.
The hereditary predisposition for preeclampsia likely is the result of interactions of literally hundreds of inherited genes—both maternal and paternal—that control myriad enzymatic and metabolic functions throughout every organ system. Plasma-derived factors may induce some of these genes in preeclampsia (Mackenzie, 2012). Thus, the clinical manifestation in any given woman with the preeclampsia syndrome will occupy a spectrum as previously discussed. In this regard, phenotypic expression will differ among similar genotypes depending on interactions with environmental factors (Yang, 2013).
Candidate Genes
Hundreds of genes have been studied for their possible association with preeclampsia (Buurma, 2013; Ward, 2014). Several of those that may have positive significant association with preeclampsia are listed in Table 40-3. Polymorphisms of the genes for Fas receptor, hypoxia-inducible factor-1α protein (HIF-1α), IL-1β, lymphotoxin-α, transforming growth factor beta 3 (TGF-β3), apolipoprotein E (ApoE), and TNF-α have also been studied with varying results (Borowski, 2009; Hefler, 2001; Jamalzei, 2013; Lachmeijer, 2001; Livingston, 2001; Wilson, 2009). Because of the heterogeneity of the preeclampsia syndrome and especially of the other genetic and environmental factors that interact with its complex phenotypic expression, it is doubtful that any one candidate gene will be found responsible. Indeed, Majander and associates (2013) have linked preeclampsia predisposition to fetal genes on chromosome 18.
TABLE 40-3. Genes with Possible Associations with Preeclampsia Syndrome
Other Genetic Variables
An extensive list of other variables affect genotypic and phenotypic expression of the preeclampsia syndrome. Some include maternal and paternal genotypes, associated disorders, genomic ethnicity, gene-gene interactions, epigenetic phenomena, and gene-environmental interactions. Combinations of these are infinite. Ethnoracial factors are important as discussed regarding the high incidence of preeclampsia in African-American women. It may be that Latina women have a lower prevalence because of interactions of American Indian and white race genes (Shahabi, 2013).
Pathogenesis
Vasospasm
The concept of vasospasm with preeclampsia was advanced by Volhard (1918) based on his direct observations of small blood vessels in the nail beds, ocular fundi, and bulbar conjunctivae. It was also surmised from histological changes seen in various affected organs (Hinselmann, 1924; Landesman, 1954). Endothelial activation causes vascular constriction with increased resistance and subsequent hypertension. At the same time, endothelial cell damage causes interstitial leakage through which blood constituents, including platelets and fibrinogen, are deposited subendothelially. Wang and associates (2002) have also demonstrated disruption of endothelial junctional proteins. Suzuki and coworkers (2003) described ultrastructural changes in the subendothelial region of resistance arteries in preeclamptic women. The much larger venous circuit is similarly involved, and with diminished blood flow because of maldistribution, ischemia of the surrounding tissues can lead to necrosis, hemorrhage, and other end-organ disturbances characteristic of the syndrome. One important clinical correlate is the markedly attenuated blood volume seen in women with severe preeclampsia (Zeeman, 2009).
Endothelial Cell Injury
As discussed on page 733, during the past two decades, endothelial cell injury has become the centerpiece in the contemporary understanding of preeclampsia pathogenesis (Davidge, 2014). In this scheme, protein factor(s)—likely placental—are secreted into the maternal circulation and provoke activation and dysfunction of the vascular endothelium. Many of the facets of the clinical syndrome of preeclampsia are thought to result from these widespread endothelial cell changes. Grundmann and associates (2008) have reported that circulating endothelial cell—CEC—levels are elevated fourfold in the peripheral blood of preeclamptic women. Similarly, Petrozella and colleagues (2012) demonstrated increased levels of circulating endothelial microparticles—EMPs—in preeclamptic women.
Intact endothelium has anticoagulant properties, and endothelial cells blunt the response of vascular smooth muscle to agonists by releasing nitric oxide. Damaged or activated endothelial cells may produce less nitric oxide and secrete substances that promote coagulation and increase sensitivity to vasopressors (Gant, 1974). Further evidence of endothelial activation includes the characteristic changes in glomerular capillary endothelial morphology, increased capillary permeability, and elevated blood concentrations of substances associated with endothelial activation. These latter substances are transferable, and serum from women with preeclampsia stimulates some of these substances in greater amounts. It seems likely that multiple factors in plasma of preeclamptic women combine to have these vasoactive effects (Myers, 2007; Walsh, 2009).
Increased Pressor Responses
As discussed in Chapter 4 (p. 61), pregnant women normally develop refractoriness to infused vasopressors (Abdul-Karim, 1961). Women with early preeclampsia, however, have increased vascular reactivity to infused norepinephrine and angiotensin II (Raab, 1956; Talledo, 1968). Moreover, increased sensitivity to angiotensin II clearly precedes the onset of gestational hypertension (Gant, 1974).
Prostaglandins
Several prostanoids are thought to be central to preeclampsia syndrome pathophysiology. Specifically, the blunted pressor response seen in normal pregnancy is at least partially due to decreased vascular responsiveness mediated by endothelial prostaglandin synthesis. For example, compared with normal pregnancy, endothelial prostacyclin (PGI2) production is decreased in preeclampsia. This action appears to be mediated by phospholipase A2 (Davidge, 2014). At the same time, thromboxane A2 secretion by platelets is increased, and the prostacyclin:thromboxane A2 ratio decreases. The net result favors increased sensitivity to infused angiotensin II and, ultimately, vasoconstriction (Spitz, 1988). These changes are apparent as early as 22 weeks in women who later develop preeclampsia (Chavarria, 2003).
Nitric Oxide
This potent vasodilator is synthesized from L-arginine by endothelial cells. Withdrawal of nitric oxide results in a clinical picture similar to preeclampsia in a pregnant animal model (Conrad, 1989). Inhibition of nitric oxide synthesis increases mean arterial pressure, decreases heart rate, and reverses the pregnancy-induced refractoriness to vasopressors. In humans, nitric oxide likely is the compound that maintains the normal low-pressure vasodilated state characteristic of fetoplacental perfusion (Myatt, 1992; Weiner, 1992). It also is produced by fetal endothelium, and here it is increased in response to preeclampsia, diabetes, and sepsis (Parra, 2001; von Mandach, 2003).
The effects of nitric oxide production in preeclampsia are unclear (Davidge, 2014). It appears that the syndrome is associated with decreased endothelial nitric oxide synthase expression, thus increasing nitric oxide inactivation. These responses may be race related, with African-American women producing more nitric oxide (Wallace, 2009).
Endothelins
These 21-amino acid peptides are potent vasoconstrictors, and endothelin-1 (ET-1) is the primary isoform produced by human endothelium (George, 2011). Plasma ET-1 levels are increased in normotensive pregnant women, but women with preeclampsia have even higher levels (Ajne, 2003; Clark, 1992). According to Taylor and Roberts (1999), the placenta is not the source of increased ET-1 concentrations, and they likely arise from systemic endothelial activation. Interestingly, treatment of preeclamptic women with magnesium sulfate lowers ET-1 concentrations (Sagsoz, 2003).
Angiogenic and Antiangiogenic Proteins
Placental vasculogenesis is evident by 21 days after conception. There is an ever-expanding list of pro- and antiangiogenic substances involved in placental vascular development. The families of vascular endothelial growth factor (VEGF) and angiopoietin (Ang) are most extensively studied. Angiogenic imbalance is used to describe excessive amounts of antiangiogenic factors that are hypothesized to be stimulated by worsening hypoxia at the uteroplacental interface. Trophoblast of women destined to develop preeclampsia overproduces at least two antiangiogenic peptides that enter the maternal circulation (Karumanchi, 2014):
1. Soluble Fms-like tyrosine kinase 1 (sFlt-1) is a variant of the Flt-1 receptor for placental growth factor (PlGF) and for VEGF. Increased maternal sFlt-1 levels inactivate and decrease circulating free PlGF and VEGF concentrations, leading to endothelial dysfunction (Maynard, 2003). As shown in Figure 40-4, sFlt-1 levels begin to increase in maternal serum months before preeclampsia is evident. Haggerty and colleagues (2012) observed that these high levels in the second trimester were associated with a doubling of the risk for preeclampsia. This divergence from normal levels appears to occur even sooner with early-onset preeclampsia (Vatten, 2012).
2. Soluble endoglin (sEng) is a placenta–derived 65-kDa molecule that blocks endoglin, which is a surface coreceptor for the TGFβ family. Also called CD105, this soluble form of endoglin inhibits various TGFβ isoforms from binding to endothelial receptors and results in decreased endothelial nitric oxide-dependent vasodilatation (Levine, 2006; Venkatesha, 2006). Serum levels of sEng also begin to increase months before clinical preeclampsia develops (Haggerty, 2012).
FIGURE 40-4 Angiogenic and antiangiogenic factors in normotensive (NT) and preeclamptic (PE) women across pregnancy. Both pairs of factors are significantly divergent by 23 to 26 weeks. sFlt = soluble Fms-like tyrosine kinase 1; PlGF = placental growth factor. (Data from Myatt, 2013.)
The cause of placental overproduction of antiangiogenic proteins remains an enigma. Concentrations of the soluble forms are not increased in the fetal circulation or amnionic fluid, and their levels in maternal blood dissipate after delivery (Staff, 2007). Research currently is focused on immunological mechanisms, oxidative stress, mitochondrial pathology, and hypoxia genes (Karumanchi, 2014). Clinical research is directed at use of antiangiogenic proteins in the prediction and diagnosis of preeclampsia. In a systematic review, Widmer and associates (2007) concluded that third-trimester elevation of sFlt-1 levels and decreased PlGF concentrations correlate with preeclampsia development after 25 weeks. These results require verification in prospective studies. Subsequently, Haggerty and coworkers (2012) reported that doubling of expressions of sFlt-1 and sEng increased the preeclampsia risk 39 and 74 percent, respectively.
PATHOPHYSIOLOGY
Although the cause of preeclampsia remains unknown, evidence for its manifestation begins early in pregnancy with covert pathophysiological changes that gain momentum across gestation and eventually become clinically apparent. Unless delivery supervenes, these changes ultimately result in multiorgan involvement with a clinical spectrum ranging from an attenuated manifestation to one of cataclysmic deterioration that is life threatening for both mother and fetus. As discussed, these are thought to be a consequence of endothelial dysfunction, vasospasm, and ischemia. Although the many maternal consequences of the preeclampsia syndrome are usually described in terms of individual organ systems, they frequently are multiple, and they overlap clinically.
Cardiovascular System
Severe disturbances of normal cardiovascular function are common with preeclampsia syndrome. These are related to: (1) increased cardiac afterload caused by hypertension; (2) cardiac preload, which is affected negatively by pathologically diminished hypervolemia of pregnancy and is increased by intravenous crystalloid or oncotic solutions; and (3) endothelial activation with interendothelial extravasation of intravascular fluid into the extracellular space and importantly, into the lungs.
Hemodynamic Changes and Cardiac Function
The cardiovascular aberrations of pregnancy-related hypertensive disorders vary depending on several modifiers. These factors include hypertension severity, underlying chronic disease, preeclampsia severity, and in which part of the clinical spectrum these are studied. In some women, these cardiovascular changes may precede hypertension onset (De Paco, 2008; Easterling, 1990; Khalil, 2012; Melchiorre, 2013). Nevertheless, with the clinical onset of preeclampsia, cardiac output declines, due at least in part to increased peripheral resistance. When assessing cardiac function in preeclampsia, consideration is given to echocardiographic measures of myocardial function and to clinically relevant ventricular function.
Myocardial Function. Serial echocardiographic studies have documented in preeclampsia evidence for ventricular remodeling that is accompanied by diastolic dysfunction in 40 percent of women (Melchiorre, 2012). In some of these women, functional differences persisted up to 16 months after delivery (Evans, 2011). Ventricular remodeling was judged to be an adaptive response to maintain normal contractility with the increased afterload of preeclampsia. In the otherwise healthy pregnant woman, these changes are usually clinically inconsequential. But when combined with underlying ventricular dysfunction—for example, concentric ventricular hypertrophy from chronic hypertension—further diastolic dysfunction may cause cardiogenic pulmonary edema as discussed in Chapters 47 (p. 943) and 49 (p. 990).
Ventricular Function. Despite the relatively high frequency of diastolic dysfunction with preeclampsia, in most women clinical cardiac function is appropriate. This has been shown by several studies in which ventricular function was assessed using invasive hemodynamic methods (Hibbard, 2014). Importantly, both normally pregnant women and those with preeclampsia syndrome can have normal or slightly hyperdynamic ventricular function (Fig. 40-5). Thus, both have a cardiac output that is appropriate for left-sided filling pressures. Data from preeclamptic women obtained by invasive hemodynamic studies are confounded because of the heterogeneity of populations and interventions that also may significantly alter these measurements. Some of these are crystalloid infusions, antihypertensive agents, and magnesium sulfate.
FIGURE 40-5 Ventricular function in normally pregnant women (striped area) and in women with eclampsia (boxed area) is plotted on a Braunwald ventricular function curve. Normal values are from Clark, 1989, and those for eclampsia are from Hankins, 1984. LVSWI = left ventricular stroke work index; PCWP = pulmonary capillary wedge pressure.
Ventricular function studies of preeclamptic women from several investigations are shown in Figure 40-6. Although cardiac function was hyperdynamic in all women, filling pressures were dependent on the volume of intravenous fluids. Specifically, aggressive hydration resulted in overtly hyperdynamic ventricular function in most women. However, this was accompanied by elevated pulmonary capillary wedge pressures. In some of these women, pulmonary edema may develop despite normal ventricular function because of an alveolar endothelial-epithelial leak. This is compounded by decreased oncotic pressure from a low serum albumin concentration (American College of Obstetricians and Gynecologists, 2012b).
FIGURE 40-6 Ventricular function in women with severe preeclampsia–eclampsia plotted on the Braunwald ventricular function curve. The pulmonary capillary wedge pressures (PCWP) are lower in those managed with restricted fluid administration (striped area in A) compared with women managed with aggressive fluid therapy (striped area in B). In those managed with aggressive fluid infusions, eight developed pulmonary edema despite normal to hyperdynamic ventricular function in all but one. LVSWI = left ventricular stroke work index. (Data for A from Benedetti, 1980; Hankins, 1984; data for B from Rafferty, 1980; Phelan, 1982.)
Thus, increased cardiac output and hyperdynamic ventricular function is largely a result of low wedge pressures and not a result of augmented myocardial contractility. By comparison, women given appreciably larger volumes of fluid commonly had filling pressures that exceeded normal, but their ventricular function remained hyperdynamic because of concomitantly increased cardiac output.
From these studies, it is reasonable to conclude that aggressive fluid administration to otherwise normal women with severe preeclampsia substantially elevates normal left-sided filling pressures and increases a physiologically normal cardiac output to hyperdynamic levels.
Blood Volume
For nearly 100 years, hemoconcentration has been a hallmark of eclampsia. This was not precisely quantified until Zeeman and colleagues (2009) expanded the previous observations of Pritchard and associates (1984) and showed in eclamptic women that the normally expected hypervolemia is severely curtailed (Fig. 40-7). Women of average size should have a blood volume of nearly 4500 mL during the last several weeks of a normal pregnancy. In nonpregnant women, this volume approximates only 3000 mL. With eclampsia, however, much or all of the anticipated normal excess 1500 mL is lost. Such hemoconcentration results from generalized vasoconstriction that follows endothelial activation and leakage of plasma into the interstitial space because of increased permeability. In women with preeclampsia, and depending on its severity, hemoconcentration is usually not as marked. Women with gestational hypertension, but without preeclampsia, usually have a normal blood volume (Silver, 1998).
FIGURE 40-7 The two bar graphs on the left compare mean blood volumes in nonpregnant and term normally pregnant women. On the right, graphs display values for a group of 29 women with eclampsia and their nonpregnant values. The red bar reflects values for a subset of 14 who had a subsequent normotensive pregnancy. Extensions above bars represent one standard deviation. Comparison between values with identical lowercase letters, that is, a-a, b-b, c-c, d-d, are significant p < .001. (Data from Zeeman, 2009, with permission.)
For women with severe hemoconcentration, it was once taught that an acute fall in hematocrit suggested resolution of preeclampsia. In this scenario, hemodilution follows endothelial healing with return of interstitial fluid into the intravascular space. Although this is somewhat correct, it is important to recognize that a substantive cause of this fall in hematocrit is usually the consequence of blood loss at delivery (Chap. 41, p. 783). It may also partially result from increased erythrocyte destruction as subsequently described. Vasospasm and endothelial leakage of plasma may persist for a variable time after delivery as the endothelium is restored to normalcy. As this takes place, vasoconstriction reverses, and as the blood volume increases, the hematocrit usually falls. Thus, women with eclampsia:
• Are unduly sensitive to vigorous fluid therapy administered in an attempt to expand the contracted blood volume to normal pregnancy levels
• Are sensitive to amounts of blood loss at delivery that are considered normal for a normotensive woman.
Hematological Changes
Several hematological abnormalities are associated with the preeclampsia syndrome. Among those commonly identified is thrombocytopenia, which at times may become severe enough to be life threatening. Occasionally, the levels of some plasma clotting factors may be decreased, and erythrocytes display abnormal morphology and undergo rapid hemolysis.
Thrombocytopenia
Decreased platelet concentrations with eclampsia were described as early as 1922 by Stancke. The platelet count is routinely measured in women with any form of gestational hypertension. The frequency and intensity of thrombocytopenia vary and are dependent on the severity and duration of the preeclampsia syndrome and the frequency with which platelet counts are performed (Heilmann, 2007; Hupuczi, 2007). Overt thrombocytopenia—defined by a platelet count < 100,000/μL—indicates severe disease (see Table 40-2). In general, the lower the platelet count, the higher the rates of maternal and fetal morbidity and mortality (Leduc, 1992). In most cases, delivery is advisable because thrombocytopenia usually continues to worsen. After delivery, the platelet count may continue to decline for the first day or so. It then usually increases progressively to reach a normal level within 3 to 5 days. As discussed on page 768, in some instances with HELLP syndrome, the platelet count continues to fall after delivery. If these do not reach a nadir until 48 to 72 hours, then preeclampsia syndrome may be incorrectly attributed to one of the thrombotic microangiopathies (George, 2013). These syndromes are discussed in Chapter 56 (p. 1116).
Other Platelet Abnormalities
In addition to thrombocytopenia, there are myriad other platelet alterations described with the preeclampsia syndrome. These were reviewed by Kenny and coworkers (2014) and include platelet activation with increased α-degranulation producing β-thromboglobulin, factor 4, and increased clearance. Paradoxically, in most studies, in vitro platelet aggregation is decreased compared with the normal increase characteristic of pregnancy. This likely is due to platelet “exhaustion” following in vivo activation. Although the cause is unknown, immunological processes or simply platelet deposition at sites of endothelial damage may be implicated. Platelet-bound and circulating platelet-bindable immunoglobulins are increased, which suggest platelet surface alterations.
Neonatal Thrombocytopenia
Severe thrombocytopenia does not develop in the fetus or infant born to preeclamptic women despite severe maternal thrombocytopenia (Kenny, 2014; Pritchard, 1987). Importantly, maternal thrombocytopenia in hypertensive women is not a fetal indication for cesarean delivery.
Hemolysis
Severe preeclampsia is frequently accompanied by evidence of hemolysis as manifest by elevated serum lactate dehydrogenase levels and decreased haptoglobin levels. Other evidence comes from schizocytosis, spherocytosis, and reticulocytosis in peripheral blood (Cunningham, 1985; Pritchard, 1954, 1976). These derangements result in part from microangiopathic hemolysis caused by endothelial disruption with platelet adherence and fibrin deposition. Sanchez-Ramos and colleagues (1994) described increased erythrocyte membrane fluidity with HELLP syndrome. Cunningham and coworkers (1995) postulated that these changes were due to serum lipid alterations, and Mackay and associates (2012) found substantively decreased long-chain fatty acid content in erythrocytes of preeclamptic women. Erythrocytic membrane changes, increased adhesiveness, and aggregation may also promote a hypercoagulable state (Gamzu, 2001).
HELLP Syndrome. Subsequent to reports of hemolysis and thrombocytopenia with severe preeclampsia, descriptions followed that abnormally elevated serum liver transaminase levels were commonly found and were indicative of hepatocellular necrosis (Chesley, 1978). Weinstein (1982) referred to this combination of events as the HELLP syndrome—and this moniker now is used worldwide. Also, and as shown in Table 40-2, facets of the HELLP syndrome are included in criteria that differentiate severe from nonsevere preeclampsia. The HELLP syndrome is discussed in further detail on page 742.
Coagulation Changes
Subtle changes consistent with intravascular coagulation, and less often erythrocyte destruction, commonly are found with preeclampsia and especially eclampsia (Kenny, 2014). Some of these changes include increased factor VIII consumption, increased levels of fibrinopeptides A and B and of D-dimers, and decreased levels of regulatory proteins—antithrombin III and protein C and S. Except for thrombocytopenia discussed above, coagulation aberrations generally are mild and are seldom clinically significant (Kenny, 2014; Pritchard, 1984). Unless there is associated placental abruption, plasma fibrinogen levels do not differ remarkably from levels found in normal pregnancy. Fibrin degradation products such as d-dimers are elevated only occasionally. Fibronectin, a glycoprotein associated with vascular endothelial cell basement membrane, is elevated in women with preeclampsia (Brubaker, 1992). As preeclampsia worsens, so do abnormal findings with thromboelastography (Pisani-Conway, 2013). Despite these changes, routine laboratory assessments of coagulation, including prothrombin time, activated partial thromboplastin time, and plasma fibrinogen level, were found to be unnecessary in the management of pregnancy-associated hypertensive disorders (Barron, 1999).
Volume Homeostasis
The normal pregnancy-induced extra- and intracellular volume increases that are accompanied by vasodilatation undergo further complex shifts with the preeclampsia syndrome. In addition to blood volume changes shown in Figure 40-7, there are many hormonal, fluid, and electrolyte aberrations.
Endocrine Changes
Plasma levels of renin, angiotensin II, angiotensin 1–7, aldosterone, and atrial natriuretic peptide are substantively increased during normal pregnancy. Deoxycorticosterone (DOC) is a potent mineralocorticoid that is also increased remarkably in normal pregnancy (Chap. 4, p. 71). This compound results from conversion of plasma progesterone to DOC rather than increased maternal adrenal secretion. Because of this, DOC secretion is not reduced by sodium retention or hypertension. This may explain why women with preeclampsia retain sodium (Winkel, 1980). In pregnancy, the mineralocorticoid receptor becomes less sensitive to aldosterone (Armanini, 2012).
Vasopressin levels are similar in nonpregnant, normally pregnant, and preeclamptic women even though the metabolic clearance is increased in the latter two (Dürr, 1999).
Atrial natriuretic peptide is released during atrial wall stretching from blood volume expansion, and it responds to cardiac contractility (Chap. 4, p. 61). Serum levels rise in pregnancy, and its secretion is further increased in women with preeclampsia (Borghi, 2000; Luft, 2009). Levels of its precursor—proatrial natriuretic peptide—are also increased in preeclampsia (Sugulle, 2012).
Fluid and Electrolyte Changes
In women with severe preeclampsia, the volume of extracellular fluid, manifest as edema, is usually much greater than that in normal pregnant women. The mechanism responsible for pathological fluid retention is thought to be endothelial injury (Davidge, 2014). In addition to generalized edema and proteinuria, these women have reduced plasma oncotic pressure. This reduction creates a filtration imbalance and further displaces intravascular fluid into the surrounding interstitium.
Electrolyte concentrations do not differ appreciably in women with preeclampsia compared with those of normal pregnant women. This may not be the case if there has been vigorous diuretic therapy, sodium restriction, or administration of free water with sufficient oxytocin to produce antidiuresis.
Following an eclamptic convulsion, the serum pH and bicarbonate concentration are lowered due to lactic acidosis and compensatory respiratory loss of carbon dioxide. The intensity of acidosis relates to the amount of lactic acid produced—metabolic acidosis—and the rate at which carbon dioxide is exhaled—respiratory acidosis.
Kidney
During normal pregnancy, renal blood flow and glomerular filtration rate are increased appreciably (Chap. 4, p. 63). With development of preeclampsia, there may be a number of reversible anatomical and pathophysiological changes. Of clinical importance, renal perfusion and glomerular filtration are reduced. Levels that are much less than normal nonpregnant values are infrequent and are the consequence of severe disease.
A small degree of decreased glomerular filtration may result from reduced plasma volume. Most of the decrement, however, is from increased renal afferent arteriolar resistance that may be elevated up to fivefold (Conrad, 2014; Cornelis, 2011). There are also morphological changes characterized by glomerular endotheliosis blocking the filtration barrier. This is discussed further subsequently. Diminished filtration causes serum creatinine levels to rise to values seen in nonpregnant individuals, that is, 1 mg/mL, and sometimes even higher (Lindheimer, 2008a). Abnormal values usually begin to normalize 10 days or later after delivery (Spaan, 2012a).
In most preeclamptic women, urine sodium concentration is elevated. Urine osmolality, urine:plasma creatinine ratio, and fractional excretion of sodium also indicate that a prerenal mechanism is involved. Kirshon and coworkers (1988) infused dopamine intravenously into oliguric women with preeclampsia, and this renal vasodilator stimulated increased urine output, fractional sodium excretion, and free water clearance. As was shown in Figure 40-6, sodium-containing crystalloid infusion increases left ventricular filling pressure, and although oliguria temporarily improves, rapid infusions may cause clinically apparent pulmonary edema. Intensive intravenous fluid therapy is not indicated as “treatment” for preeclamptic women with oliguria. Exceptions are diminished urine output from hemorrhage or fluid loss from vomiting or fever.
Plasma uric acid concentration is typically elevated in preeclampsia. The elevation exceeds the reduction in glomerular filtration rate and likely is also due to enhanced tubular reabsorption (Chesley, 1945). At the same time, preeclampsia is associated with diminished urinary excretion of calcium, perhaps because of increased tubular reabsorption (Taufield, 1987). Another possibility is from increased placental urate production compensatory to increased oxidative stress.
Proteinuria
As shown in Table 40-1, some degree of proteinuria will establish the diagnosis of preeclampsia syndrome. Proteinuria may develop late, and some women may already be delivered or have had an eclamptic convulsion before it appears. For example, 10 to 15 percent of women with HELLP syndrome did not have proteinuria at presentation (Sibai, 2004). In another report, 17 percent of eclamptic women did not have proteinuria by the time of seizures (Zwart, 2008).
Problematically, the optimal method of establishing abnormal levels of either urine protein or albumin remains to be defined. Chen and associates (2008) have shown that clean-catch and catheterized urine specimens correlate well. But dipstick qualitative determinations depend on urinary concentration and are notorious for false-positive and -negative results. For a 24-hour quantitative specimen, the “consensus” threshold value used is > 300 mg/24 h (Task Force, 2013). Tun and colleagues (2012) have shown equivalent efficacy using protein excretion of 165 mg in a 12-hour sample. Importantly, these values have not been irrefutably established.
Determination of urinary protein:creatinine ratio may supplant the cumbersome 24-hour quantification (Ethridge, 2013; Kyle, 2008; Morris, 2012). In a systematic review, Papanna and associates (2008) concluded that random urine protein:creatinine ratios that are below 130 to 150 mg/g, that is, 0.13 to 0.15, indicate a low likelihood of proteinuria exceeding 300 mg/day. Midrange ratios, that is, 300 mg/g or 0.3 have poor sensitivity and specificity. Stout and colleagues (2013) found that values < 0.08 or > 1.19 had negative- or positive-predictive values of 86 and 96 percent, respectively. At this time, most recommend that with midrange ratio values, 24-hour protein excretion should be quantified.
There are several methods used to measure proteinuria, and none detect all of the various proteins normally excreted (Nayeri, 2013). A more accurate method involves measurement of albumin excretion. Albumin filtration exceeds that of larger globulins, and with glomerular disease such as preeclampsia, most of the protein in urine is albumin. There are test kits that permit rapid measurement of urinary albumin:creatinine ratios in an outpatient setting (Kyle, 2008).
Although worsening or nephrotic-range proteinuria has been considered by most to be a sign of severe disease, this does not appear to be the case (Airoldi, 2007). Certainly, this concept was not accepted by the 2013 Task Force. Finally, increasing proteinuria is more common in multifetal pregnancy complicated by preeclampsia (Zilberberg, 2013).
Anatomical Changes
Sheehan and Lynch (1973) commonly found changes identifiable at autopsy by light and electron microscopy in the kidneys of eclamptic women. Glomeruli are enlarged by approximately 20 percent, they are “bloodless,” and capillary loops variably are dilated and contracted. Endothelial cells are swollen—termed glomerular capillary endotheliosis by Spargo and associates (1959). Endothelial cells are often so swollen that they block or partially block the capillary lumens (Fig. 40-8). Homogeneous subendothelial deposits of proteins and fibrin-like material are seen.
FIGURE 40-8 Schematic showing glomerular capillary endotheliosis. The capillary of the normal glomerulus shown on the left has wide endothelial fenestrations, and the pedicels emanating from the podocytes are widely spaced (arrow). The illustration on the right is of a glomerulus with changes induced by the preeclampsia syndrome. The endothelial cells are swollen and their fenestrae narrowed, as are the pedicels that now abut each other.
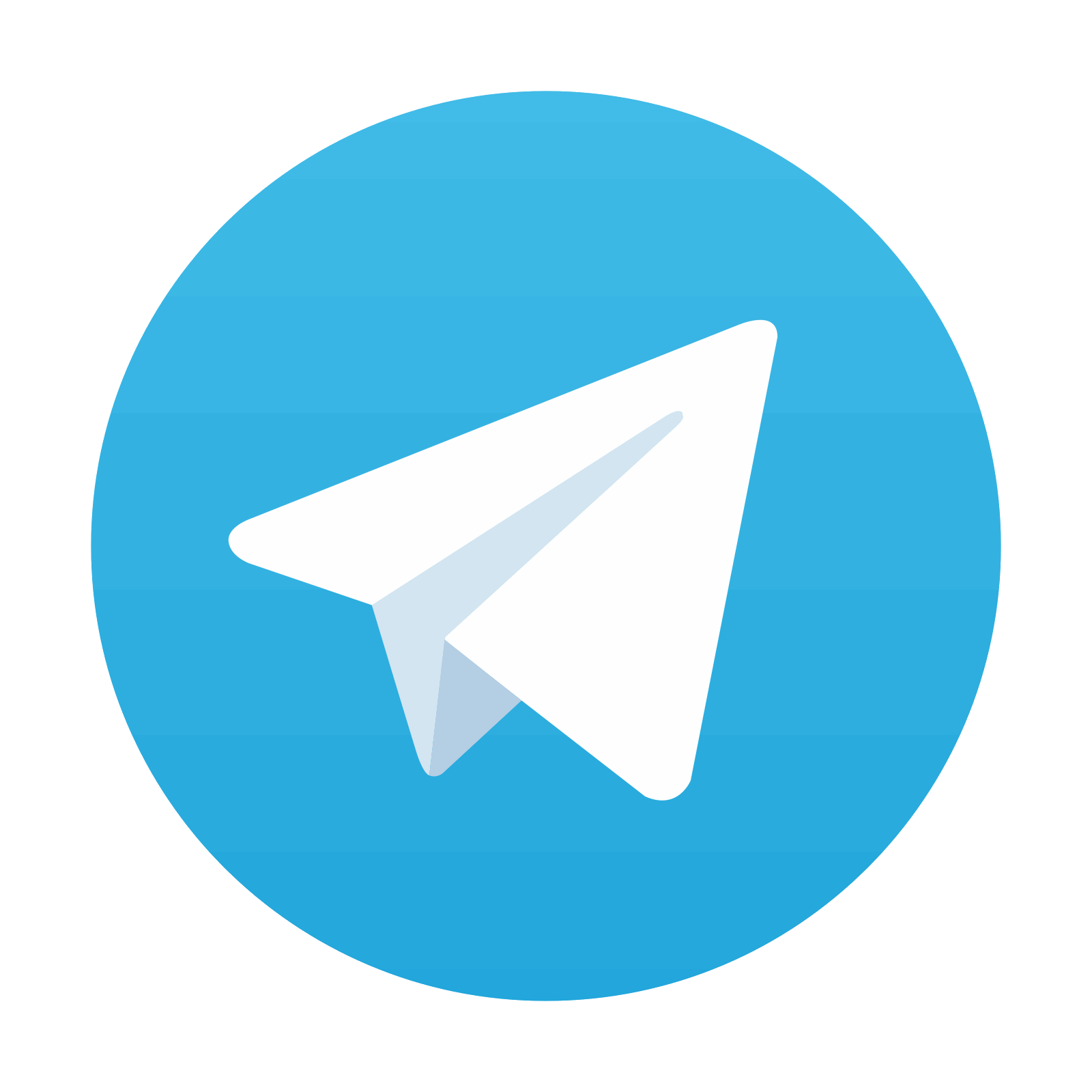
Stay updated, free articles. Join our Telegram channel

Full access? Get Clinical Tree
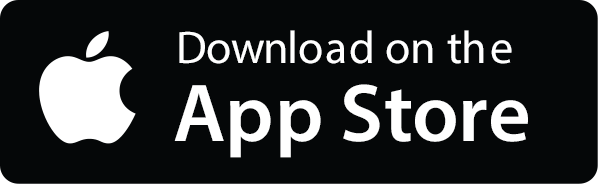
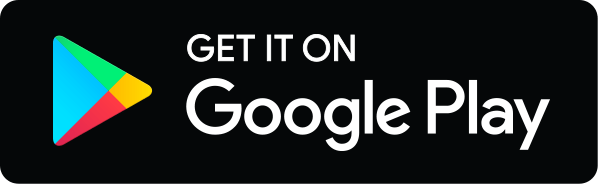