Background
Despite good anatomic and functional outcomes, urogynecologic polypropylene meshes that are used to treat pelvic organ prolapse and stress urinary incontinence are associated with significant complications, most commonly mesh exposure and pain. Few studies have been performed that specifically focus on the host response to urogynecologic meshes. The macrophage has long been known to be the key cell type that mediates the foreign body response. Conceptually, macrophages that respond to a foreign body can be dichotomized broadly into M1 proinflammatory and M2 proremodeling subtypes. A prolonged M1 response is thought to result in chronic inflammation and the formation of foreign body giant cells with potential for ongoing tissue damage and destruction. Although a limited M2 predominant response is favorable for tissue integration and ingrowth, excessive M2 activity can lead to accelerated fibrillar matrix deposition and result in fibrosis and encapsulation of the mesh.
Objective
The purpose of this study was to define and compare the macrophage response in patients who undergo mesh excision surgery for the indication of pain vs a mesh exposure.
Study Design
Patients who were scheduled to undergo a surgical excision of mesh for pain or exposure at Magee-Womens Hospital were offered enrollment. Twenty-seven mesh-vagina complexes that were removed for the primary complaint of a mesh exposure (n = 15) vs pain in the absence of an exposure (n = 12) were compared with 30 full-thickness vaginal biopsy specimens from women who underwent benign gynecologic surgery without mesh. Macrophage M1 proinflammatory vs M2 proremodeling phenotypes were examined via immunofluorescent labeling for cell surface markers CD86 (M1) vs CD206 (M2) and M1 vs M2 cytokines via enzyme-linked immunosorbent assay. The amount of matrix metalloproteinase-2 (MMP-2) and matrix metalloproteinase-9 (MMP-9) proteolytic enzymes were quantified by zymography and substrate degradation assays, as an indication of tissue matrix degradation. Statistics were performed with the use of 1-way analysis of variance with appropriate post hoc tests, t -tests, and Fisher’s Exact test.
Results
Twenty-seven mesh-vaginal tissue complexes were excised from 27 different women with mesh complications: 15 incontinence mid urethral slings and 12 prolapse meshes. On histologic examination, macrophages surrounded each mesh fiber in both groups, with predominance of the M1 subtype. M1 and M2 cytokines/chemokines, MMP-9 (pro- and active), and MMP-2 (active) were increased significantly in mesh-vagina explants, as compared with vagina without mesh. Mesh explants that were removed for exposure had 88.4% higher pro-MMP-9 ( P = .035) than those removed for pain. A positive correlation was observed between the profibrotic cytokine interleukin-10 and the percentage of M2 cells ( r = 0.697; P = .037) in the pain group.
Conclusion
In women with complications, mesh induces a proinflammatory response that persists years after implantation. The increase in MMP-9 in mesh explants that were removed for exposure indicates degradation; the positive association between interleukin-10 and M2 macrophages in mesh explants that are removed for pain is consistent with fibrosis.
Over the past decade, lightweight, wide-pore polypropylene mesh has been used increasingly in the repair of pelvic organ prolapse and stress urinary incontinence. Despite favorable anatomic and functional outcomes, mesh use has been associated with complications, most commonly mesh exposure through the vaginal epithelium and pain. Studies of similar meshes that are used in hernia repair have demonstrated that all polypropylene meshes induce a prolonged inflammatory response at the site of implantation. The magnitude and type of response is associated with the development of complications. Although it is tempting to extrapolate these findings to meshes that are applied to the vagina, data suggest that the host response at these 2 sites is distinct.
The host response after the placement of any foreign material into the body has been well described. After the initial recruitment of neutrophils, macrophages become the primary immune cell to be involved in the clearance of debris and the initiation of the host response. Although this is an essential initial component of healing, the long-term presence of activated inflammatory cells, such as macrophages at the mesh tissue interface, can impact negatively the ability of the mesh to function as intended.
Macrophages have been classified as having diverse and plastic phenotypes along a continuum between M1 (classically activated; proinflammatory) and M2 (alternatively activated; remodeling, homeostatic) extremes. M1 macrophages are characterized by the secretion of reactive oxygen species and proinflammatory cytokines and chemokines and can be identified via the cell surface marker CD86. Persistence of M1 macrophages can lead to tissue damage and destruction. In contrast, M2 macrophages secrete growth factors and antiinflammatory immune modulators and can be identified by the cell surface marker CD206. M2 macrophages participate in the constructive healing and remodeling phase of the foreign body response and result in tissue deposition and in growth. However, an overzealous M2 response can also lead to excess tissue deposition and fibrosis. As such, macrophage polarization and plasticity play an important and determinant role in tissue remodeling after injury and the integration of biomaterials. Limited data exist about the macrophage response after implantation of urogynecologic meshes particularly in regard to implantation in the vagina.
The objective of the current study was to characterize the macrophage response that is present in patients who undergo mesh excision surgery and to define differences in this response according to the 2 most common complications: mesh exposure and pain. Mesh-tissue constructs from women who underwent mesh excision surgery for the indication of pain and exposure were compared with full-thickness vaginal biopsy specimens from women who underwent prolapse repairs with the use of morphologic, biochemical, and immunologic endpoints.
Materials and Methods
Patient acquisition
Patients who were scheduled to undergo surgical excision of mesh as part of a larger study (Magee Mesh Biorepository IRB# 10090194) were offered enrollment. For inclusion in the current study, mesh had to be removed from the anterior or apical compartment for the primary indication of exposure or pain. Mesh exposure was defined as at least 2 mm of mesh visible through the vaginal epithelium; pain was defined as mesh being removed for the primary complaint of pain (with palpation, ambulation, or intercourse) without evidence of exposure. Patients were excluded from the study if they had acute infection (fever, worsening pain, and pus in area of mesh) or erosion into the bowel or bladder. Patients were also excluded if they were unable to provide informed consent, were undergoing chronic immunosuppressive therapy, or had an autoimmune disorder. After consent was obtained, baseline demographic data that were abstracted from the electronic medical record included age, race/ethnicity, body mass index (BMI), gravidity, parity, hormone use, menopausal status, and smoking status ( Table 1 ). Menopausal status was defined as premenopausal (regular menstrual periods within the last 12 months) and postmenopausal (no menstrual periods within the last 12 months). Hormone use was defined as current use of systemic estrogen with or without progesterone or vaginal estrogen for ≥ 3 months. Smoking was defined as current smoker (yes/no). Operative reports from the initial mesh surgery were reviewed, and the type of mesh was recorded.
Variables | Mesh complications (n = 27) | Prolapse (n = 30) | P value | |
---|---|---|---|---|
Mesh exposure (n = 15) | Pain (n = 12) | |||
Mean age, y±SD | 56.1 ± 8.0 | 52.1 ± 9.7 | 52.9 ± 9.2 | .527 |
Mean body mass index, kg/m 2 ± SD | 30.8 ± 5.9 | 27.3 ± 3.3 | 28.5 ± 4.0 | .158 |
Median gravidity, n (25 %ile, 75 %ile) | 3.0 (2.0, 4.0) | 3.5 (2.0, 5.75) | 2.0 (2.0, 3.0) | .644 |
Median parity, n (25 %ile, 75 %ile) | 2.0 (2.0, 3.0) | 3.0 (2.0, 5.0) | 2.0 (2.0, 3.0) | .899 |
Mean time implanted, mo ± SD | 36.9 ± 30.3 | 30.9 ± 18.0 | NA | .527 |
Menopausal status, n (%) | ||||
Premenopausal | 2 (13) | 3 (25) | 12 (40) | |
Postmenopausal | 13 (87) | 9 (75) | 18 (60) | .190 |
Smoking, n (%) | ||||
Nonsmoker | 12 (80) | 9 (75) | 27 (90) | |
Smoker | 3 (20) | 3 (25) | 3 (10) | .713 |
Race/ethnicity, n (%) | ||||
White | 15 (100) | 12 (100) | 29 (97) | |
Indian | 0 | 0 | 1 (3) | |
Other | 0 | 0 | 0 | 1.000 |
Hormonal usage, n (%) | ||||
Yes | 7 (47) | 7 (58) | 7 (23) | |
No | 8 (53) | 5 (42) | 23 (77) | .077 |
On the day of surgery, the excised mesh-tissue complex was placed in a sterile specimen container, immediately placed on ice, and sent for analysis. Samples from patients with mesh were age, BMI, and menopausal status–matched to full-thickness vaginal biopsy specimens that were obtained from the anterior vagina at the vaginal apex in mesh-naïve women with stage II or III prolapse with and without incontinence who underwent pelvic surgery, as described previously (IRB # 0412054). This group of vaginal biopsy specimens was selected as a control for the present study because there is evidence that the vaginal biopsy specimens from women with prolapse and incontinence have increased matrix metalloproteinases (MMPs) relative to women with normal pelvic organ support.
Tissue extract acquisition and histologic preparation
Excised tissue-mesh complexes and nonmesh control tissues were extracted in high salt extraction buffer as described previously. Additional pieces of vagina-mesh-complexes and nonmesh control tissue were embedded into O.C.T. compound (Tissue-Tek; Sakura Finetek USA Inc, Torrance, CA), flash frozen in liquid nitrogen, sectioned (7 μm), and stored at –80°C.
Cytokine and chemokine determination
Quantification of cytokines interleukin (IL)-10, IL-4, tumor necrosis factor-α (TNF-α), IL-12p70, and IL-12p40p70 and chemokines CXCL10 and CCL17 was performed with the use of commercially available enzyme-linked immunosorbent assay kits (Life Technologies, Carlsbad, CA, and R&D Systems, Minneapolis, MN, respectively). All samples were run in duplicate or triplicate with the use of 40-μg total protein per sample per assay. A patient sample that had been characterized previously for analyte amounts served as an internal control.
Zymographic analyses
Samples that contained 30 μg total protein were analyzed in duplicate by substrate zymography according to manufacturer’s instructions (Novex; Life Technologies). For quantification of active and proenzyme forms of MMP-2, band density was measured with ImageJ software (National Institute of Health, Bethesda, MD), and measurements were normalized to an internal control.
MMP-9 activity assay
Endogenous MMP-9 activity was measured with a Fluorokine E Human Active MMP-9 kit (R&D Systems). For each experiment, 20 μg of protein from each sample was tested in duplicate. Fluorescence was read with a Spectramax M2 spectrophotometer (Molecular Devices, Sunnyvale, CA) at an excitation wavelength of 320 nm and an emission wavelength of 405 nm. Data were analyzed with the use of a 4-parameter regression curve (Masterplex ReaderFit; Miraibio, San Francisco, CA) and normalized to nanogram-active MMP-9 per milligram of protein in each sample.
Immunofluorescent labeling
Tissue sections were quadruple-labeled for pan-macrophage marker CD68, M1 macrophage marker CD86, M2 macrophage marker CD206, and nuclear marker 4’,6-diamidino-2-phenylindole, as described, and imaged with a Nikon ECLIPSE 90i upright microscope (Nikon USA, Melville, NY). For mesh-vagina complexes, six ×200 images were acquired over 2 locations within the tissue. Three images were taken in an area of a mesh fiber (defined as a single fiber of polypropylene not immediately adjacent to other fibers), and 3 additional images were taken in the area of a mesh knot (defined as ≥3 single fibers immediately adjacent to each other). For control patients, six ×200 images were taken over 2 areas of each tissue. For each image, 2 trained technicians counted the number of total cells and the number of cells that coexpressed either CD68 and CD86 or CD68 and CD206 to define the M1 and M2 macrophage population, respectively. For each field the M2:M1 ratio was calculated, as described in Wolf et al, by the use of the formula (raw number of M2 macrophages+1)/(raw number of M1 macrophages +1) to avoid division by 0 in samples with no cells present.
Statistical analysis
Power analysis showed that 8 samples in each group were necessary to reach statistical significance for cytokines that used previously obtained IL-10 values in vaginal extracts from nonhuman primate with and without Gynemesh PS (Ethicon, Inc., Somerville, NJ) implanted via sacrocolpopexy. Statistical analysis was performed with SPSS software (version 21; IBM, Armonk, NY). For demographic data, a 1-way analysis of variance with Tukey post hoc testing, Kolmogorov-Smirnov tests, and Fisher’s Exact tests with significance level α = .05 were used. For the biochemical endpoints of mesh patients vs control patients and for the complication exposure vs pain, a 2-tailed Student’s independent samples t -test was performed. For histologic endpoints that compared areas of a mesh fiber with a mesh knot, a paired-sample t -test was used (α = .05); when we compared tissue removed for exposure vs pain, an independent-sample t -test was used (α = .05). Pearson’s correlations were used to correlate cytokines with histologic findings.
Results
Demographic data
Twenty-seven mesh-vaginal tissue complexes were excised from 27 women: 15 incontinence midurethral slings and 12 prolapse meshes. Four of the 27 meshes were implanted via abdominal sacrocolpopexy, and the remainder were inserted transvaginally. There were no differences in patient age, race/ethnicity, BMI, gravidity, parity, hormone use, menopausal status, smoking status, or duration of mesh implantation (all P > .05; Table 1 ). In addition, there were no differences in patient demographics or experimental endpoints when data were separated by prolapse meshes vs midurethral slings. The specific meshes that were excised are listed in Table 2 . Mesh type was not found in 3 meshes that were removed for pain and 1 mesh that was removed for exposure. Meshes were explanted from 4.5–93 months after the index surgery.
Mesh device | Removal because of exposure (n = 15) | Removal because of pain (n = 12) |
---|---|---|
AMS Monarc TOT a | 1 | 0 |
AMS Perigee a | 1 | 0 |
Bard Ajust Single Incision Sling b | 0 | 1 |
Bard Soft Mesh b | 0 | 1 |
Boston Scientific Lynx TVT c | 1 | 1 |
Boston Scientific Obtryx TOT c | 1 | 0 |
Boston Scientific Solyx mini sling c | 0 | 1 |
Boston Scientific Uphold c | 1 | 0 |
Caldera Desara Sling System for SUI d | 1 | 0 |
Coloplast Novasilk e | 1 | 0 |
Gynecare Prolift mesh kit f | 1 | 1 |
Gynecare Gynemesh PS f | 4 | 0 |
Gynecare TVT Secur f | 1 | 2 |
Gynecare TVT f | 1 | 1 |
Gynecare TOT f | 0 | 1 |
Original medical records not available g | 1 | 3 |
a American Medical Systems, Minnetonka, MN
b C.R. Bard, Inc., Murray Hill, NJ
c Boston Scientific Corporation, Marlborough, MA
d Caldera Medical, Inc., Agoura Hills, CA
e Coloplast Corporation, Minneapolis, MN
f Ethicon, Inc., Somerville, NJ
g Of the 27 patients who were enrolled in this study, mesh brand information could not be determined for 4 of the patients.
Biochemical endpoints
All M1 proinflammatory and M2 proremodeling cytokines and chemokines were increased in mesh explants as compared with nonmesh tissue ( Table 3 ), which indicated a robust, active, and ongoing host response to polypropylene long after implantation. Examination of the proremodeling cytokines IL-4 and -10 showed a 1.18-fold increase ( P = .011) and 1.45-fold increase ( P = .016), respectively, in mesh-vagina explants vs controls. Proinflammatory cytokines TNF-α, IL-12p40p70, and IL-12p70 had a 2.13-fold increase ( P < .001), a 2.19-fold increase ( P < .001), and a 1.22-fold increase ( P = .001), respectively, in mesh explants vs controls. The proinflammatory chemokine CXCL10 and the proremodeling chemokine CCL17 were increased 3.38-fold ( P = .016) and 7.26-fold ( P < .001) relative to controls, respectively. Comparison of the ratio of the M2 proremodeling cytokines (IL-10+IL-4) with the M1 proinflammatory cytokines (TNF-α+IL-12p70) revealed a decrease in mesh explants as compared with controls ( P = .003), which indicated a shift towards a proinflammatory profile.
Biomarkers | Mesh complications (exposure + pain) (n = 27), mean ± SD | Prolapse control (n = 30), mean ± SD | P value |
---|---|---|---|
Cytokines & chemokines a | |||
Interleukin-4 | 3.316 ± 0.860 | 2.800 ± 0.567 | .011 |
Interleukin-10 | 17.738 ± 9.957 | 12.218 ± 5.932 | .016 |
Tumor necrosis factor-α | 15.963 ± 6.392 | 7.487 ± 2.224 | .000 |
Interleukin-12p40p70 | 28.519 ± 16.437 | 13.029 ± 7.227 | .000 |
Interleukin-12p70 | 2.070 ± 0.471 | 1.691 ± 0.291 | .001 |
CXCL10 | 62.534 ± 71.835 | 18.492 ± 61.065 | .016 |
CCL17 | 33.940 ± 38.156 | 4.674 ± 3.681 | .000 |
Proteases | |||
Pro–matrix metalloproteinase2 b | 1.192 ± 0.851 | 1.324 ± 0.971 | .601 |
Active matrix metalloproteinase2 b | 1.521 ± 1.636 | 0.733 ± 0.783 | .038 |
Pro–matrix metalloproteinase-9 c | 3.496 ± 2.643 | 1.226 ± 1.715 | .000 |
M2/M1 cytokines & chemokines | |||
(Interleukin-4+interleukin-10)/(tumor necrosis factor-α +interleukin-12p70) | 1.209 ± 0.562 | 1.633 ± 0.486 | .003 |
CCL17/CXCL10 | 0.852 ± 0.921 | 0.661 ± 0.597 | .364 |
Pro- and active MMP-9 were 2.85-fold increased ( P < .0001) and 2.91-fold increased ( P < .0001), respectively, in mesh explants as compared with controls. Although pro–MMP-2 was similar in complexes with and without mesh, active MMP-2 was 2.08-fold increased ( P = .038).
Pro–MMP-9 was 1.88-fold higher with exposure than pain ( P = .036). No statistical difference was observed for active MMP-9 ( P = .067). Values for individual cytokines and chemokines and for levels of pro- and active MMP2 did not differ based on indication for mesh removal ( Table 4 ).
Biomarkers | Mesh, mean ± SD | P value | |
---|---|---|---|
Exposure | Pain | ||
Cytokines & chemokines a | |||
Interleukin-4 | 3.307 ± 0.973 | 3.328 ± 0.736 | .952 |
Interleukin-10 | 19.323 ± 11.665 | 15.757 ± 7.308 | .342 |
Tumor necrosis factor-α | 15.954 ± 5.011 | 15.976 ± 8.039 | .994 |
Interleukin-12p40p70 | 31.739 ± 20.242 | 24.493 ± 9.235 | .231 |
Interleukin-12p70 | 2.040 ± 0.564 | 2.108 ± 0.342 | .705 |
CXCL10 | 71.384 ± 86.873 | 51.472 ± 48.495 | .459 |
CCL17 | 38.445 ± 49.238 | 28.312 ± 17.132 | .467 |
Proteases | |||
Pro–matrix metalloproteinase-2 b | 0.965 ± 1.018 | 1.420 ± 0.606 | .200 |
Active matrix metalloproteinase-2 b | 1.278 ± 0.887 | 1.764 ± 2.164 | .483 |
Pro–matrix metalloproteinase-9 c | 4.860 ± 3.464 | 2.586 ± 1.660 | .036 |
Active matrix metalloproteinase-9 c | 6.063 ± 4.674 | 3.578 ± 1.338 | .067 |
M2/M1 cytokines & chemokines | |||
(Interleukin-4+interleukin-10)/(tumor necrosis factor-α+interleukin-12p70) | 1.266 ± 0.640 | 1.138 ± 0.462 | .551 |
CCL17/CXCL10 | 0.864 ± 1.165 | 0.837 ± 0.529 | .937 |
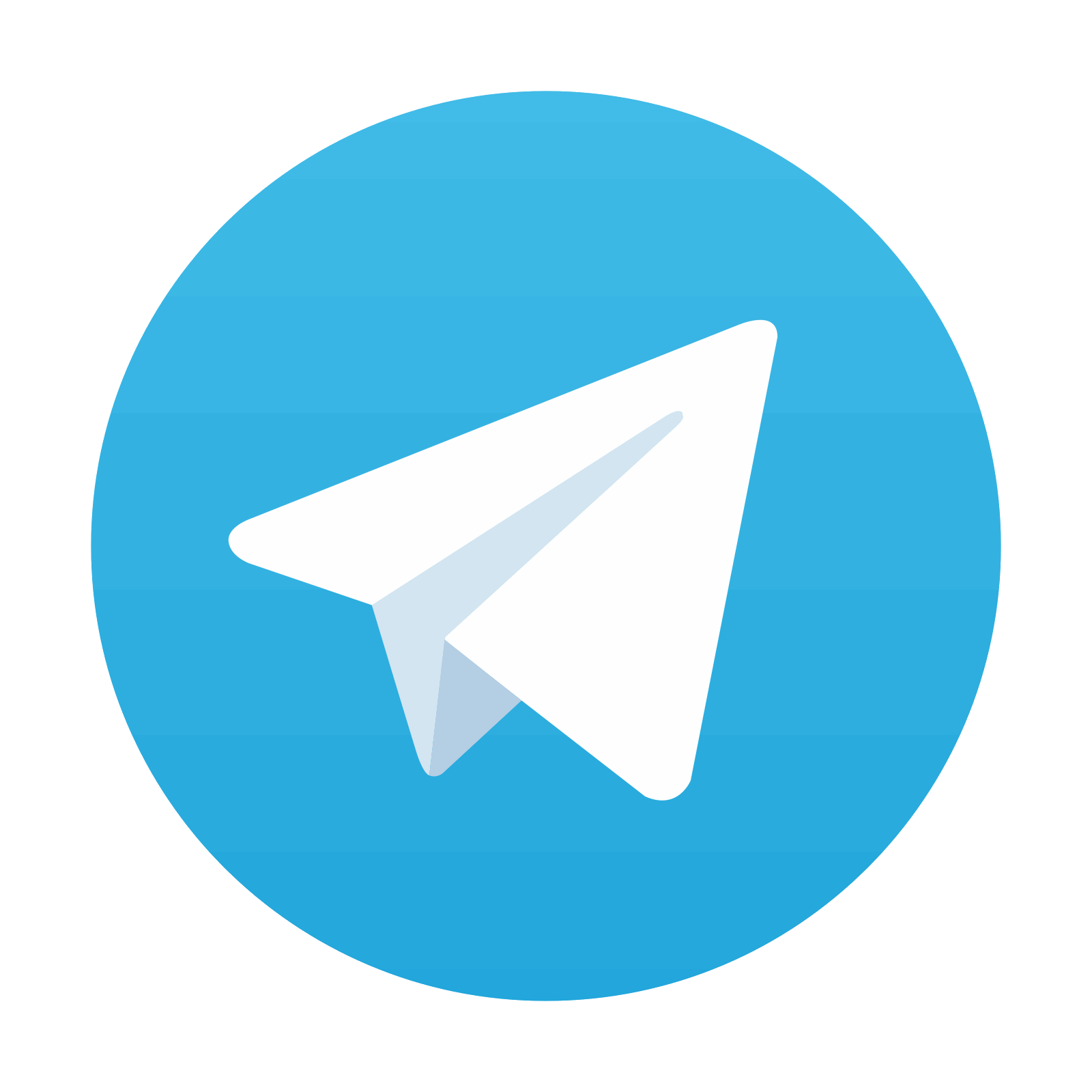
Stay updated, free articles. Join our Telegram channel

Full access? Get Clinical Tree
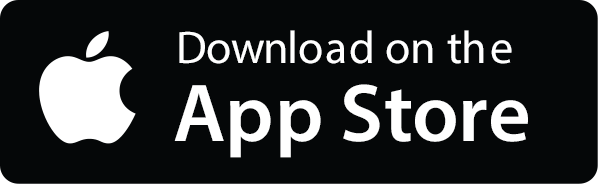
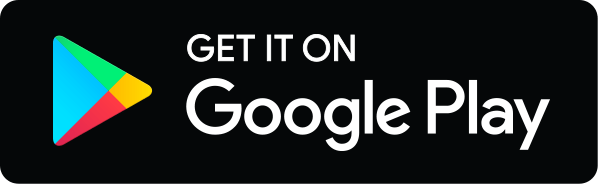