Fig. 34.1
Pulmonary interstitial emphysema. (a) Severe. This was often seen in the presurfactant era. Note the overdistension and the pneumothorax on the right side (With courtesy of Pr C. ADAMSBAUM, Pediatric Radiology Department, Cochin-Saint-Vincent-de-Paul Hospital, Paris). (b) Mild. This is more often seen today when PIE occurs. It is limited to some linear and small cyst radiolucencies in a globally distended lung
The differential diagnosis includes lobar emphysema or, when localized, a cystic malformation of the lung (Greenough 2003). PIE must also be distinguished from air bronchogram in which branching radiolucencies follow the normal anatomic distribution of the bronchial tree.
PIE can be the cause of pneumothorax or pneumomediastinum, is frequently associated with BPD, and can be fatal with a failure to ventilate the baby.
Small subpleural blebs can be observed at autopsy but may be difficult to see post-mortem. Cystic spaces are present in the pulmonary parenchyma or in interlobular and subpleural connective tissue or in perivascular lymphatics (Greenough 2003).
34.3.2 Pneumothorax
A large pneumothorax is marked by a sudden deterioration with profound respiratory distress. Tachypnea is always present (Korones 2003). Grunting, signs of retraction with a high Silverman score, and cyanosis are present in all infants. On inspection, the side of the pneumothorax may be prominent compared to the other side. Abdominal distension may be observed as a result of the pressure of the pneumothorax on the diaphragm. Auscultation demonstrates decreased or absent breath sounds and a shift of heart sounds to the opposite site of the air leak. Low blood pressure and heart rate are very frequent (Ogata et al. 1976).
The diagnosis may be less obvious, also marked, in a ventilated infant, by deterioration of the respiratory status, mainly oxygenation (Ogata et al. 1976), and reduced chest wall movement. Trends in monitoring of TCPCO2 may help to make an earlier diagnosis (McIntosh et al. 2000). Presence of risk factors such as low gestational age, RDS, and high inflation pressure as well as the presence of PIE or pneumomediastinum on radiographs should raise the suspicion of possible pneumothorax. Small pneumothoraces may be discovered only on systematic chest radiographs.
Transillumination with a fiberoptic light source is a very useful tool, especially in small infants (Kuhns et al. 1975; Watkinson and Tiron 2001), allowing a very rapid diagnosis in an infant with sudden deterioration. The light is placed on the infant’s chest wall and shows increased transmission of light on the affected side which illuminates the hemithorax. It allows emergency treatment of the pneumothorax without waiting for the radiograph.
The diagnosis is based on chest radiograph, which is mandatory in infants in a stable condition. A large pneumothorax (Fig. 34.2a) is diagnosed by the absence of lung parenchyma and a collapsed lung. If the lung is not compliant, total collapse may not be seen and signs can be limited to a small shift of mediastinal structures to the opposite side or the identification of the pleural line (Fig. 34.2b). The diaphragm may be pushed downward. Sometimes, the differential diagnosis with large pneumomediastinum may be difficult. Small pneumothoraces on radiographs taken in the supine position may also be detected only by a difference of radiolucency in one lung which appears to be “clearer” despite the presence of lung parenchyma or limited to identification of a pleural line. Two techniques can be used to confirm the diagnosis: a lateral radiograph with horizontal beam while the infant is in the supine position, which shows an anterior collection of air, and (in a stable infant) an anteroposterior radiograph with horizontal beam taken with the infant kept in lateral decubitus on the opposite side of the suspected air leak, which displays the pleural line due to the upward movement of air.
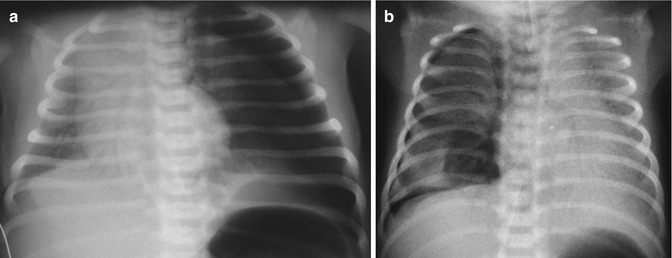
Fig. 34.2
Pneumothorax. (a) Large left pneumothorax with absence of parenchyma after the pleural line, collapsed lung, and shift of the mediastinal structures on the opposite side. (b) Small right pneumothorax. The pleural curve is well seen
Ultrasound has also been proposed, at least for traumatic pneumothorax (Dente et al. 2007), but there are no published reports in infants.
34.3.3 Pneumomediastinum
Pneumomediastinum occurs in about 2.5 per 1,000 live births (Morrow et al. 1967). Spontaneous pneumomediastinum in healthy infants is due to the same mechanisms as spontaneous pneumothorax. Postmature infants and infants with meconium aspiration syndrome are at increased risk.
These infants, when pneumomediastinum is isolated, are usually asymptomatic or present very few signs of respiratory distress, often limited to tachypnea, a bulging sternum, and muffled heart sounds. They may sometimes be cyanosed.
The diagnosis is frequently based on systematic radiographs of ventilated infants, or when indicated by light signs of respiratory distress. Air is seen surrounding the heart on the anteroposterior view, but not extending to the diaphragmatic border (Korones 2003). Occasionally, the thymus can be seen above the heart, which is highly suggestive of pneumomediastinum. A very specific sign is the “spinnaker sail sign” (Fig. 34.3) which is due to elevation of the thymus away from the heart as a consequence of air accumulation laterally to the cardiac borders (Lawal et al. 2009). The sail is separated from surrounding structures by radiolucency. The differential diagnosis must exclude bronchogenic cyst (Shah et al. 1999).
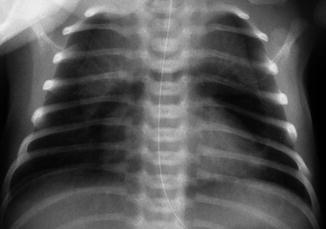
Fig. 34.3
Pneumomediastinum with the spinnaker sail sign
34.3.4 Pneumopericardium
Pneumopericardium may occur in 2 % of infants hospitalized to the NICU. Hook et al. found a rate of 2 % in about 2,400 VLBW infants and 3.5 % in 1,349 ventilated infants (Hook et al. 1995).
In the majority of cases, pneumopericardium occurs in ventilated, very preterm infants with high ventilation pressures. Glenski and Hall reported 19 cases of pneumopericardium and 16 of these infants presented RDS. The striking finding was the high peak inspiratory pressure, mean airway pressure, and long inspiratory times just before the onset of pneumopericardium (Glenski and Hall 1984). Pneumopericardium can also occur in infants on nasal CPAP (Heckmann et al. 1998).
Pneumopericardium may sometimes be asymptomatic and discovered incidentally on radiographs. However, it is usually responsible for cardiac tamponade with rapid deterioration including hypotension, impalpable peripheral pulses, and cyanosis. Bradycardia rapidly occurs. On auscultation, cardiac sounds are muffled or inaudible and a friction rub can rarely be heard (Greenough 2003). ECG monitoring shows a decrease of ECG voltages (Gan and Simpson 2005). The diagnosis is based on radiographs showing air surrounding the heart including the inferior surface (Fig. 34.4) and outlining the great vessels. The presence of air over the diaphragmatic surface of the heart distinguishes it from pneumomediastinum. The transverse heart diameter may be reduced. On lateral views, air is present in the anterior part of the heart, separating it from the sternum. Pneumopericardium is responsible for a high mortality rate (Hook et al. 1995) ranging between 60 and 90 % depending on management, and may be responsible for developmental delay.
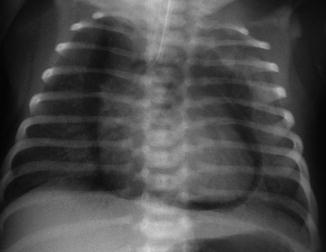
Fig. 34.4
Pneumopericardium. Air surrounding the heart, including the inferior part. This pneumopericardium was asymptomatic and discovered on systematic radiograph. It was treated conservatively and resolved within 24 h
34.3.5 Pneumoperitoneum
Pneumoperitoneum is due to the same mechanisms as pneumothorax and pneumomediastinum and can therefore occur spontaneously. It is usually associated with RDS and mechanical ventilation and occurs in infants with pneumothorax or pneumomediastinum.
It is clinically asymptomatic except when a large volume of air is present, leading to abdominal distension. In massive pneumoperitoneum, the inferior vena cava may be compressed, decreasing blood return and compromising systemic circulation.
The diagnosis is radiographic, showing air in the peritoneal space (Fig. 34.5a). The lateral radiograph of the abdomen shows air collected in the superior portion of the abdomen (Fig. 34.5b) (Sivit 2006).
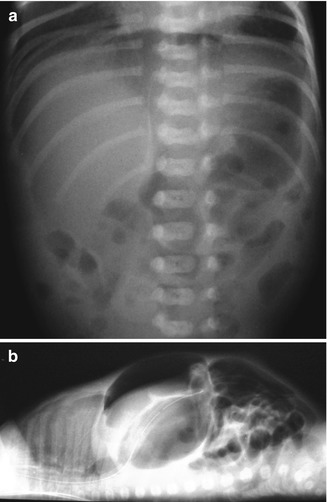
Fig. 34.5
Pneumoperitoneum. (a) Anteroposterior view showing air beneath the diaphragm, oval global translucency, and outlined falciform ligament of the liver. (b) Lateral view with horizontal beam showing air in the superior part of the abdomen and anterior to the viscera, just below the abdominal wall (Note that this pneumoperitoneum had a gastrointestinal origin)
The main problem is to distinguish the origin of the pneumoperitoneum, as rupture of the gastrointestinal tract must be excluded. The presence of large thoracic air leaks may help to guide the diagnosis, as well as evidence of a preexisting digestive disorder. The diagnosis of an isolated intestinal perforation can be difficult. Needle aspiration of intraperitoneal air can also guide the diagnosis by showing the absence of stools or bile. Intraperitoneal gas analysis has been proposed to distinguish between pneumoperitoneum due to thoracic air leaks and bowel perforation. A partial pressure of oxygen clearly lower than that delivered by the ventilator is suggestive of surgical pneumomediastinum (e.g., of digestive origin), while a clearly higher partial pressure of oxygen is suggestive of ventilator-induced pneumoperitoneum (Vanhaesebrouck et al. 1989). A procedure using a mixture of helium and oxygen (heliox) has also been proposed with an analysis of intraperitoneal air showing the presence of helium (Jaarsma et al. 1999).
34.3.6 Systemic Air Embolism
Systemic air embolism is an uncommon complication (Beluffi and Perotti 2009), resulting from direct communication between the airways, connective tissue, and pulmonary vessels.
In a review of 50 cases, Lee and Tanswell showed that an air leak syndrome was present in 94 % of cases in infants with air embolism, usually presenting severe respiratory failure treated with high ventilation pressures. Clinically, the infant presents with a sudden and dramatic deterioration with sudden collapse, pallor, cyanosis, hypotension, and electrocardiographic abnormalities. Blanching and migrating cutaneous pallor has also been reported. Air can be found in blood drawn from a catheter. There may be an unusually high oxygen tension on TcPO2 monitoring. Radiographs may show free air in the arterial and venous systems (Lee and Tanswell 1989). The prognosis is very poor with a very high mortality. In the previous series, only 4 out of 53 infants survived.
34.3.7 Subcutaneous Emphysema
Subcutaneous emphysema is usually secondary to pneumomediastinum and is not frequently observed in neonates. Subcutaneous emphysema is easily diagnosed by the presence of subcutaneous gas which is confirmed by radiographs.
34.4 Management and Treatment Options
34.4.1 Pulmonary Interstitial Emphysema
34.4.1.1 Generalized PIE
Resolution of PIE with very short inspiratory time (Ti) has been reported (Meadow and Cheromcha 1985), but it is difficult to achieve adequate ventilation with this setting of Ti. The use of high-frequency ventilation may be a solution, at least to improve gas exchanges and to improve the radiological features of PIE, but not the long-term outcome (Keszler et al. 1991). The first studies were performed at a time when very high pressures were used for conventional ventilation (Frantz et al. 1983; Keszler et al. 1991), and it may therefore be difficult to extrapolate these results to considerably improved conditions of conventional ventilation. The use of continuous negative pressure has also been proposed (Cvetnic et al. 1989).
When PIE is very severe, precluding correct gas exchange, spontaneous or induced resolution into pneumothorax or pneumomediastinum may help to improve ventilation. Lung puncture (Milligan et al. 1984) or placement of chest tubes in large pneumatoceles (Arias-Camison et al. 2001) may therefore constitute a possible treatment option. Surgery has been proposed but with a high mortality and appears to be a drastic procedure in a potentially reversible disease. Dexamethasone has been reported to be effective (Fitzgerald et al. 1998) but must be considered carefully in view of the current knowledge on steroids in preterm infants.
34.4.1.2 Localized PIE
The ventilatory options proposed above can also be tried in localized or unilateral PIE, but additional treatments designed to limit inflation in the affected zone can also be proposed. They are therefore dependent on the possibility to limit ventilation to the non-affected lung, but with the risk of inducing PIE in the non-affected lung.
The first option consists of placing the baby in the lateral decubitus position to promote atelectasis of the affected lung (Cohen et al. 1984) associated with cautious respiratory care including minimal chest physiotherapy and suctioning.
Selective intubation, which is easier in the right bronchus than in the left bronchus, can then be attempted with gentle ventilation to the ventilated lung, possibly using high-frequency ventilation (Randel and Mannino 1989). The non-ventilated lung becomes atelectatic and PIE resolves within 48 h (Chan and Greenough 1992). It can be difficult to selectively intubate the left main bronchus, and various intubation techniques have been proposed (Chalak et al. 2007). Ventilation of one lung can also be obtained by occlusion of a mainstem bronchus by a Swan-Ganz catheter (Rastogi et al. 2007).
34.4.2 Pneumothorax
Pneumothorax is often asymptomatic especially spontaneous pneumothorax in otherwise healthy infants, and does not require any treatment. Increasing the oxygen concentration has been proposed to facilitate reabsorption of extra-alveolar air (Chernick and Avery 1963) as a nitrogen washout method. To our knowledge, this widely used practice has not being evaluated in controlled trials and must be used cautiously and avoided in preterm infants due to the potential risk of oxygen.
Even in mechanically ventilated infants, the need for supplementary procedures, such as needle aspiration or drain placement, has been reviewed in a recent series (Litmanovitz and Carlo 2008). This retrospective study included 14,614 infants admitted to the NICU from 1992 to 2005, including 307 (2.1 %) infants with pneumothorax. The charts of 136 mechanically ventilated infants were reviewed (19 term, 117 preterm). Seven of the term infants were treated without a chest tube (1 with needle aspiration) and 28 preterm infants were treated without chest tube (13 with needle aspiration). This clearly indicates that a chest tube may not be necessary in ventilated infants in whom pneumothorax is well tolerated.
Needle aspiration with a syringe and a butterfly needle may be a diagnostic and emergency treatment procedure, before insertion of a chest tube, in order to obtain immediate decompression. It is usually performed with a 22–18 G butterfly needle attached to a 20–30 ml syringe via a three-way tap. The needle is inserted through the skin and then shifted slightly before going through the muscle in order to avoid air entry through the hole created after removal of the needle.
Negative pressure must be maintained in the syringe while inserting the needle into the pleura. To avoid lung puncture, it is preferable not to remove all air. Note that in the series already cited (Litmanovitz and Carlo 2008), infants treated by needle aspiration were more likely to have a chest drain than those treated by purely expectant management.
Chest drains are usually used in ventilated babies with deterioration of gas exchange or tension pneumothorax. Placement in an anterior position is essential to obtain more effective drainage (Allen et al. 1981) but is not easily achieved via the midaxillary site (fourth to sixth intercostal spaces) usually used for insertion in order to minimize cosmetic sequelae. Chest drains inserted too deeply can also be ineffective. Two types of chest tubes can be used: the standard 10–14 Fr drain, which requires blunt dissection through the intercostal space, and a 5–6 Fr pigtail catheter (Wood and Dubik 1995) which minimizes chest wall and lung trauma due to the smaller diameter and decreased depth of insertion. Chest tubes are usually safe despite a recent report of an accident in which the catheter impaled the lung (Brooker et al. 2007). The tube is connected to an underwater sealed drain and suction is applied at a negative pressure of 10–20 cm H2O. Heimlich valves can be used for transport. The position of the drain must be checked by radiograph, including a lateral horizontal beam chest film that shows the anteroposterior position (Fig. 34.6a, b). If drainage is insufficient, the drain position must be checked and modified if the catheter is too deep, or a second catheter can be inserted, especially when the first catheter appears to be occluded. Air bubbling in the bottle indicates that pneumothorax is still leaking. When bubbling has stopped for 12–48 h, the drain is clamped for several hours and can be removed if air does not accumulate in the pleura, e.g., if the radiograph shows no recurrence of the pneumothorax and unclamping of the drain does not result in new bubbles in the bottle. Complications of chest drains include lung perforation, chylothorax, pericardial effusion, phrenic nerve injuries, and bronchopleural fistula (Greenough 2003).
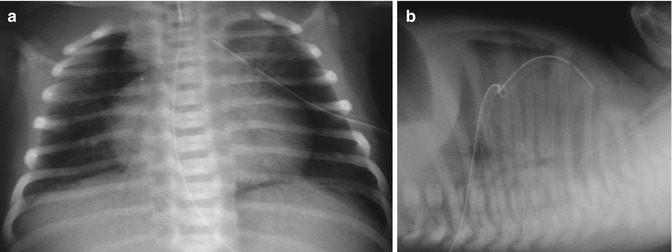
Fig. 34.6
Drained pneumothorax. (a) Anteroposterior radiograph. Note that the drain is a little too deep. (b) Lateral radiograph with horizontal beam. The drain is in anterior position and the pneumothorax is well drained
34.4.3 Pneumomediastinum
Pneumomediastinum is usually asymptomatic and therefore does not require any treatment, except a careful monitoring of the baby to detect a possible evolution towards a pneumothorax. As for pneumothorax, resorption can be accelerated by cautious administration of oxygen to term infants. Exceptionally, when pneumomediastinum induces a respiratory or circulatory dysfunction, drainage may be attempted but is usually difficult. The use of high-frequency oscillation (HFO) has been proposed (Miyahara et al. 1999).
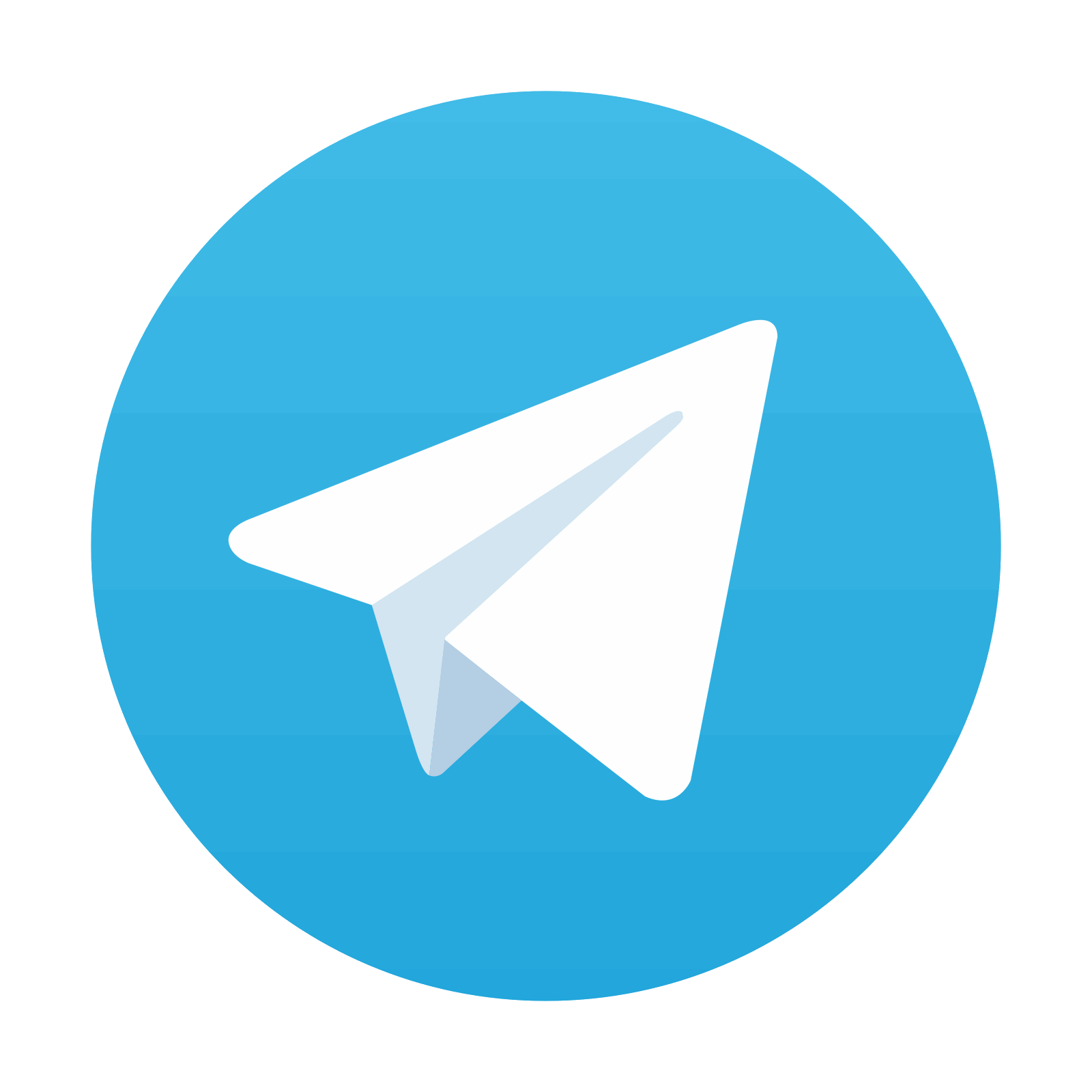
Stay updated, free articles. Join our Telegram channel

Full access? Get Clinical Tree
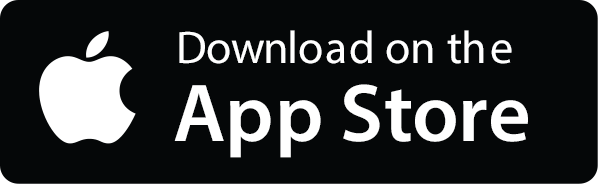
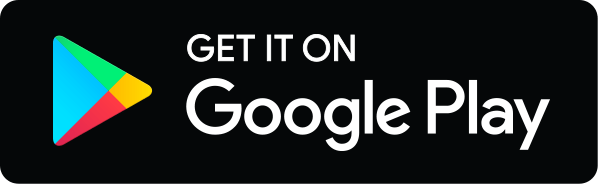