Key Points
- •
Asthma pathology has defining physiologic correlates, including airflow limitation, heightened airway responsiveness to stimuli and improved airflow in response to bronchodilators.
- •
Spirometry is the most common quantitative test used to measure airflow obstruction.
- •
Serial tests of lung function are valuable in following the course of asthma.
- •
Decreases in airflow plus hyperinflation (i.e. air trapping) are seen in acute asthma.
- •
During exacerbations, signs and symptoms of asthma often resolve before lung function returns to normal.
- •
Hypoxemia is common in asthma exacerbations while hypercapnia is a sign of more severe airflow limitation.
Asthma is characterized by intermittent airway obstruction, which commonly manifests as shortness of breath, cough, wheezing and chest tightness. However, even moderate degrees of airflow obstruction may not be symptomatic or clinically apparent. When present, the degree of airflow limitation varies greatly, from mild and self-limited to life-threatening. Between these extremes are gradations of obstruction that can be quantified. Testing airflow, along with the change in response to therapies or challenges (e.g. exercise, mannitol, methacholine), is useful when assessing and managing patients with asthma. This chapter deals with functional assessments of asthma in the pediatric patient, emphasizing tests that reflect airway and lung function. The primary focus is on studies that can be easily performed in an office or clinic setting, but tests that require more sophisticated equipment usually unavailable in these locations are also noted. Furthermore, because asthma often first presents in preschool children, tests of lung function that may be performed in the youngest patients are mentioned. Equipment necessary to measure pulmonary function in these young children typically exists only in centers with expertise in pediatric pulmonary medicine. However, knowledge of airway function close to the onset of disease is an important subject of ongoing investigations into the pathogenesis of the disorder, and newer techniques may be more amenable to widespread use in outpatient clinics.
A decline in airway function as measured by pulmonary function tests often reflects a change in airway structure; therefore, this chapter begins with an overview of the pathology of asthma. Greater detail on this subject can be found in other chapters. Appreciating the correlation between airway structure and function is important to clinical management. For example, understanding the pathologic findings in severe asthma helps one to recognize components contributing to obstruction that are poorly responsive to acute treatment and take time to resolve. This will be reflected in tests of airway function soon after a patient is ill. Similarly, tests that define airway responsiveness may also correlate with lung pathology in subjects in whom the disease is clinically quiescent.
This chapter will also review how tests of lung function can help identify diseases that may mimic asthma and will consider the ways in which pulmonary function tests may help guide therapy in both acute and chronic conditions. Studies involving infants, children and adolescents are cited whenever possible. Investigations into structural and functional assessments of the disease in adults are cited when they provide additional insight into the process.
The Pathology of Asthma
Fatal asthma is typified by marked airway inflammation with mucus and cellular debris, epithelial desquamation, subepithelial collagen deposition and airway wall thickening resulting in luminal obstruction. Several factors contribute to airway wall thickening, including smooth muscle hypertrophy, edema, goblet cell hyperplasia and tissue infiltration by inflammatory cells. These features have been noted at autopsy, not only in adults but also in pediatric patients of varying ages. Similar pathologic findings can be seen in biopsies from severe but nonfatal asthma.
With the more recent use of bronchoalveolar lavage (BAL) and endobronchial biopsies to address asthma pathogenesis, it is apparent that airway inflammation is a hallmark of even clinically mild asthma. Common features include infiltration of airways by eosinophils, activation of T cells within airways, increase in mast cell numbers and desquamation of airway epithelium. These studies have been performed primarily in adults, with a loose correlation found between indices of inflammation and the level of airway responsiveness to provocative agents such as histamine and methacholine. Less information is available from pediatric patients, but work using BAL in older children and adolescents suggests that pathologic findings are similar to the abnormalities described in adults. For example, Ferguson and colleagues reported an association between the level of airway responsiveness to histamine and both eosinophil numbers and mast cell tryptase within BAL fluid of 6- to 16-year-old children. A more recent study found that, among preschool children with wheezing, increased airway smooth muscle present in endobronchial biopsy was associated with having asthma at school age.
The Physiology of Asthma
Asthma is defined by physiologic abnormalities. As noted earlier, these abnormalities include variable airflow limitation, increased responsiveness of airways to provocation (i.e. bronchoconstriction) and improved airflow in response to bronchodilators (reversibility). Given these features and the common discrepancy between lung function and patient symptoms, asthma is a disease that is best quantified by objective testing. Several measures of lung mechanics have been used to describe asthma during both symptomatic and asymptomatic phases of the disease. These measures include lung volumes, the pressure-volume characteristics of the lung, resistance to airflow and flow rates. A discussion of each of these measures in childhood asthma is presented, emphasizing flow rates and lung volumes as these are most commonly used by practicing physicians treating children. Airway hyperresponsiveness, a fundamental feature of this disease, is also discussed.
Spirometry
Spirometry is the most common technique used to assess pulmonary function. It is helpful to understand several key elements of spirometric assessment before discussing the role of this test in patient care. First, values obtained by spirometry are reported as absolute volume and, more importantly, in reference to that predicted for normal lung function based on the age, sex, height and race or ethnicity of the patient. Therefore, it is critical that these demographic factors are correctly noted and used. If spirometry test results are unexpectedly higher or lower than suggested by the history and examination, one is wise to double-check the accuracy of these demographic data. Second, the quality of both patient technique and effort will greatly affect spirometry data. Studies suggest that many tests performed in routine outpatient clinics are of suboptimal quality but that limited staff training can significantly improve this. Most children can learn to perform spirometry tests with reasonable quality by the age of 5 to 7 years. Third, those interpreting spirometry tests should be properly trained. Physicians should be familiar with useful quality criteria that have been published by the American Thoracic Society/European Respiratory Society (ATS/ERS), including forced expiratory time, repeatability and back extrapolated volume. However, data suggest that the clinical utility of spirometry data in children may depend more on the experience of the interpreter than on rigid numerical test parameters. In other words, pediatric spirometry test results that do not meet published numerical quality standards are often still useful – especially when considered in the context of an individual patient.
Providers should also be aware of important efforts to improve spirometry interpretation. A notable international collaboration has recently produced the largest reference data set for spirometry to date. The Global Lung Function Initiative (GLI) reference equations include over 74,000 spirometric measurements from healthy, nonsmoking males and females aged 3 to 95 years, provided by over 70 organizations worldwide. This includes spirometric indices from five ethnic groups and over 30,000 healthy young people between 2.5 and 18 years old. Using modern statistical techniques, these data have been used to generate the Quanjer GLI-2012 prediction equations, endorsed by all major respiratory societies and incorporated by manufacturers into many lung function devices. Ongoing efforts will contribute even greater representation of ethnic backgrounds into this reference data set, providing more reliable normal values across age and demographic characteristics. Also, many providers have moved to reporting data as Z-scores rather than percent predicted of normal. A Z-score of −1.64 represents the 5 th percentile and has been proposed as a lower limit of normal for spirometry. Ninety-five percent of healthy subjects will fall within ± 2 Z-scores. Interpretation by lower limit of normal using Z-score is independent of age, height, sex and ethnic group, and may more clearly show how abnormal (i.e. far from the median) an individual’s lung function is at the time of testing. Similar prevalence rates for impaired FEV 1 /FVC ratio and FEV 1 have been reported when transitioning from the familiar Hankinson and Wang equations to the GLI-2012.
Definitive Characteristics of Asthma
A
Airflow Limitation
The usual method of measuring the degree of airflow limitation is to assess lung function during a maximal forced exhalation. The subject exhales forcibly from total lung capacity (TLC) to residual volume (RV) into either a spirometer or through a flow meter by which flow is integrated to give volume. The results are usually expressed as either a time-based recording of expired volume (spirogram) or a plot of instantaneous airflow against lung volume (maximal expiratory flow-volume [MEFV] curve). The tests of lung function derived from a spirogram are the forced vital capacity (FVC; TLC minus RV), the forced expiratory volume during the first second of exhalation (FEV 1 ), and the forced expiratory flow from 25% to 75% of the FVC (FEF 25–75 ). From the flow-volume curve, the maximal expiratory flow rate (MEFR) achieved approximates the peak expiratory flow rate (PEFR) obtained from a flow meter. Flow rates at and below 50% of the vital capacity (VC) are also obtained as part of this maneuver. Because airflow is related to lung volume, plethysmography combined with the MEFV maneuver plotted as a flow-volume curve or loop allows assessment of the relationship between airflow and absolute lung volumes ( Figure 30-1 ). Measurement of flow rates in this manner may be informative when an isovolumetric shift occurs (discussed later). The effect of lung volume on airflow also highlights the importance of subject effort and technique.

In subjects with airflow obstruction due to asthma, the expected pattern of altered flow rates during an exacerbation can be predicted based on airway pathology and obstructive physiology. On spirometry, both the FEV 1 and FEF 25–75 are diminished, although the former is more preserved as a percent of predicted than the latter. Since the FVC is usually relatively preserved, the FEV 1 /FVC ratio is reduced. On the MEFV curve, the expiratory portion of the loop typically becomes concave, or ‘scooped out’ (see Figure 30-1 ) due to a greater impairment in flows at low lung volumes. These flows are the first to decrease and the last to return to normal. The MEFR, like its counterpart, the FEV 1 , is more preserved during acute attacks and is quicker to normalize.
In a minority of cases, the spirogram or MEFV curve alone will not reflect significant airway obstruction. However, if subjects are studied with both an MEFV maneuver and plethysmography to assess lung volumes, they will have a displacement of the flow-volume curve to a higher lung volume without a change in the configuration of the curve itself. Thus, if flow is measured as a percent of the VC, no change in flow is appreciated. However, when the same curve is plotted as a function of the absolute lung volumes present before and after onset of symptoms, substantial changes in flow become apparent at the same lung volume, that is, at an isovolume. This represents an isovolumetric shift to a higher lung volume. The factors responsible for isovolumetric shifts are poorly defined but may include closure of some airways with loss of the contribution of these more obstructed units to the flow-volume pattern.
In acute asthma, loss of symptoms and signs of asthma does not mean that lung function has returned to normal. Classic studies by McFadden and colleagues demonstrated that when patients with severe, acute asthma became asymptomatic, the overall mechanical function of their lungs in terms of the FEV 1 was still only 40% to 50% of predicted normal values. Thus loss of clinical signs of airway obstruction does not necessarily mean there has been physiologic recovery. This highlights the important role of measuring lung function when managing patients with asthma, especially those prone to severe airflow obstruction or those who have difficulty appreciating decline in lung function.
The use of peak flow meters within the home is an inexpensive method of monitoring a flow rate to assess asthma stability. Although there are limitations, in that the PEFR may be normal while other spirometric indices are abnormal, home monitoring of lung function can contribute to the care of selected patients. In this respect, significant changes in PEFR may be manifest before symptoms are evident, particularly in patients with limited recognition of early disease exacerbation. These devices may also be especially helpful in defining the presence and severity of nocturnal asthma. The diurnal variation of PEFR (i.e. the difference between morning and evening measurements) is normally less than 10%. A PEFR variability of greater than 15% to 20% has been used as one defining feature of nocturnal asthma. Patients with such variability should be regarded as having more severe asthma and inadequate disease control. Additionally, given that excessive diurnal variations in lung function during recovery from status asthmaticus have been associated with an increased risk of sudden death, this vulnerable period of time may warrant close monitoring both in the hospital and home environment. Therefore, in more severe patients or those with limited recognition of signs and symptoms of exacerbation, monitoring the PEFR as part of their daily routine may allow for earlier recognition of loss of control with more timely intervention. Finally, it should be noted that the PEFR maneuver is technique- and effort-dependent with most home meters. This should be considered in younger patients and those who may have secondary gain with asthma-related illness.
B
Heightened Airway Responsiveness
Airway responsiveness is commonly defined as the ease with which airways narrow in response to various nonallergic and nonsensitizing stimuli, including inhaled pharmacologic agents (e.g. histamine, methacholine, mannitol) as well as natural physical stimuli (e.g. exercise, exposure to cold air). Heightened airway responsiveness to several stimuli causing bronchoconstriction and reduced airflow is a hallmark of asthma. Even when conventional assessments of lung function are normal in children with chronic stable asthma, the airways often exhibit this heightened responsiveness. The most common method of quantifying airway responsiveness is to assess lung function (usually FEV 1 ) before and after inhaling increasing concentrations of methacholine. The test is concluded when a defined decrease in lung function has been achieved; for the FEV 1 , this is usually a 20% decrease from baseline values. The more responsive the airways, the less methacholine is needed to decrease lung function. Exercise often increases FEV 1 in healthy patients, therefore a decrease of as little as 10% from baseline may reflect exercise-induced bronchoconstriction.
The level of airway responsiveness to pharmacologic agents has been noted to correlate roughly with the severity of disease in both adults and children. Thus asthmatic subjects who are the most responsive are generally the most symptomatic (wheeze, cough, chest tightness) and require more medications to control their disease. Although there can be great variability in responsiveness within groups of patients classified by disease severity, the concept that the level of responsiveness correlates with disease severity is important when considering factors that lead to loss of asthma control. In this respect, the level of airway responsiveness is not static in either normal individuals or asthmatic subjects but may increase or decrease in response to various stimuli. When responsiveness increases, control of the disease is often lost in that this is when asthmatic subjects develop signs and symptoms of their disease. In general, stimuli that increase responsiveness are found in our environment and induce or exacerbate airway inflammation. For children, these stimuli commonly include various viral respiratory infections, air pollutants (including cigarette smoke) and allergens.
A viral respiratory infection is a common antecedent to acute episodes of asthma in children. This has been documented for several respiratory viruses, including respiratory syncytial virus and rhinovirus. In terms of air pollutants, both nitrogen dioxide and ozone have been shown to enhance airway responsiveness. Cigarette smoke is arguably the most serious environmental air pollutant in terms of the respiratory health of children and has been implicated in the onset as well as the perpetuation of the disease. In addition, exposure of atopic individuals to relevant allergens can lead to significant increases in airway responsiveness that persist for days to months. These classes of disease precipitants are often considered separately, but they assuredly have combined effects in an asthmatic subject’s airways which contribute to disease instability.
Just as airway responsiveness will increase in response to certain stimuli that lead to airway inflammation, the level of responsiveness will also decrease if measures are taken to decrease inflammation within airways. These measures include use of medications with anti-inflammatory properties (e.g. inhaled corticosteroids), long-acting bronchodilators and the avoidance of relevant allergens (atopic asthmatic subjects) and cigarette smoke.
C
Improved Airflow in Response to Bronchodilators
Increased pulmonary function, often measured by FEV 1 or FEV 25–75 and in response to short-acting bronchodilators such as inhaled β-agonists, is another defining feature of asthma. An increase in FEV 1 by 12% predicted or more is often used to define a significant response to bronchodilator medication. Greater improvement in FEF 25–75 is typically required (e.g. 25% predicted) and this more variable parameter is not used by all centers to determine response to bronchodilators. When testing response to other forms of inhaled bronchodilators it is important to understand the pharmacokinetics and predicted time needed for a medication to take effect.
Lung Volumes
During an exacerbation of asthma, all of the various capacities and volumes of gas contained in the lung may be altered to some extent. The RV, functional residual capacity (FRC) and TLC are usually increased (RV > FRC > TLC), whereas the VC and its subdivisions are decreased (see Figure 30-1 ). These alterations have been described during natural exacerbation of asthma in adults and children. Although laboratory induced changes in lung volumes (exercise, histamine challenge) may be immediately normalized with inhalation of a bronchodilator, it may take weeks after an episode of severe, acute asthma for the RV to return to a normal range. The mechanisms responsible for the increases in RV, FRC (hyperinflation) and TLC (overdistension) are not completely understood. However, several factors have been identified that may contribute, including a generalized decrease in the elastic properties of the lung, a ball-valve phenomenon caused by swollen and mucus-plugged airways, and tonic activity in the intercostal muscles and diaphragm during episodes of obstruction.
Arterial Blood Gases
The primary function of the lung is to provide for gas exchange such that oxygen is taken up and delivered to the body while carbon dioxide is eliminated. This function may be altered when control of asthma is lost. Several studies of acute asthma have correlated arterial blood gases with the level of airway obstruction. One of the classic descriptions is the work of McFadden and Lyons. These authors studied a large population (101 subjects) who, because of age (14 to 45 years) and medical history, were unlikely to have their asthma complicated by bronchitis and emphysema. This study and others (cited later) provide an important description of the expected abnormalities in gas exchange as a function of the degree of airway obstruction.
Oxygen Tension
McFadden and Lyons found that the characteristic blood gas pattern in patients who were experiencing acute asthma was hypoxemia associated with respiratory alkalosis. The hypoxemia was the most consistent abnormality found in their study. A near linear correlation was found between values of FEV 1 and arterial oxygen tension ( Figure 30-2, top ). Patients with an FEV 1 of 50% to 85% of their predicted normal values were arbitrarily classified as having mild airway obstruction; those with values of 26% to 50%, moderate obstruction; and those with values of less than 25%, severe obstruction. The mean values of arterial oxygen tension (in mm Hg as measured at sea level) ranked by disease severity were 82.8, 71.3 and 63.1, respectively. Thus there was almost a 20-mm Hg difference in arterial oxygen tensions between the mild and severe groups. Just as important, it was also noted that some degree of hypoxemia was encountered at all levels of airway obstruction. In terms of studies in children, Weng and colleagues noted similar findings in asthmatic subjects who were 14 months to 14 years old. The study found that all symptomatic asthma patients were hypoxemic, with the level of hypoxemia correlating with the degree of airflow obstruction.

Several mechanisms are likely to contribute to the hypoxemia just described. The primary mechanism is thought to be an alteration in ventilation-perfusion ratios. For severely obstructed subjects, in whom atelectatic alveoli are still being perfused, transitory anatomic shunts may also contribute to the hypoxemia. In the most severely obstructed subjects, alveolar hypoventilation is also likely to be important.
The normal response of the body to a decrease in arterial oxygen is to increase ventilation. A reduced chemosensitivity to hypoxia coupled with a blunted perception of dyspnea may predispose patients to fatal asthma attacks. Kikuchi and colleagues found that adult patients with a history of near-fatal asthma had respiratory responses to hypoxia that were significantly lower than responses in normal subjects and in asthmatic subjects without near-fatal attacks. The lower hypoxic response was seen in conjunction with a blunted perception of dyspnea. These abnormalities could occur because of preexisting genetic factors as well as adaptation of the body to recurrent hypoxia. The relative importance of these and other factors is unknown. Children with poor perception of airway obstruction may be at risk for fatal or near-fatal asthma.
Carbon Dioxide Tension
McFadden and Lyons demonstrated that respiratory alkalosis often accompanies hypoxemia in asthmatic subjects experiencing exacerbations. In terms of carbon dioxide tensions, their study suggested that most attacks were associated with alveolar hyperventilation and that hypercapnia was not likely to occur until extreme degrees of obstruction were reached. Plotting airway obstruction (percent predicted FEV 1 ) against the carbon dioxide tension indicated that hypercapnia was not seen until the FEV 1 fell to less than 20% of its predicted value (see Figure 30-2 , bottom). Thus a ‘normal’ or elevated PaCO 2 in a patient with acute asthma is cause for concern.
Arterial Values of pH
Arterial pH values in acute asthma typically reflect this respiratory alkalosis. In the study of McFadden and Lyons, 73 of the 101 subjects had a respiratory alkalosis (mean pH 7.46); 21, normal pH; and 7, respiratory acidosis (mean pH 7.32). Weng and colleagues reported similar results in children. A metabolic acidosis may also be seen in acute asthma. Although this is not commonly seen in adults, it has been noted in combination with a respiratory acidosis in children with severe asthma. This acid-base imbalance is usually associated with very severe airway obstruction. Although the mechanisms responsible for the metabolic acidosis remain to be clarified, we know that these subjects are in imminent danger of respiratory failure.
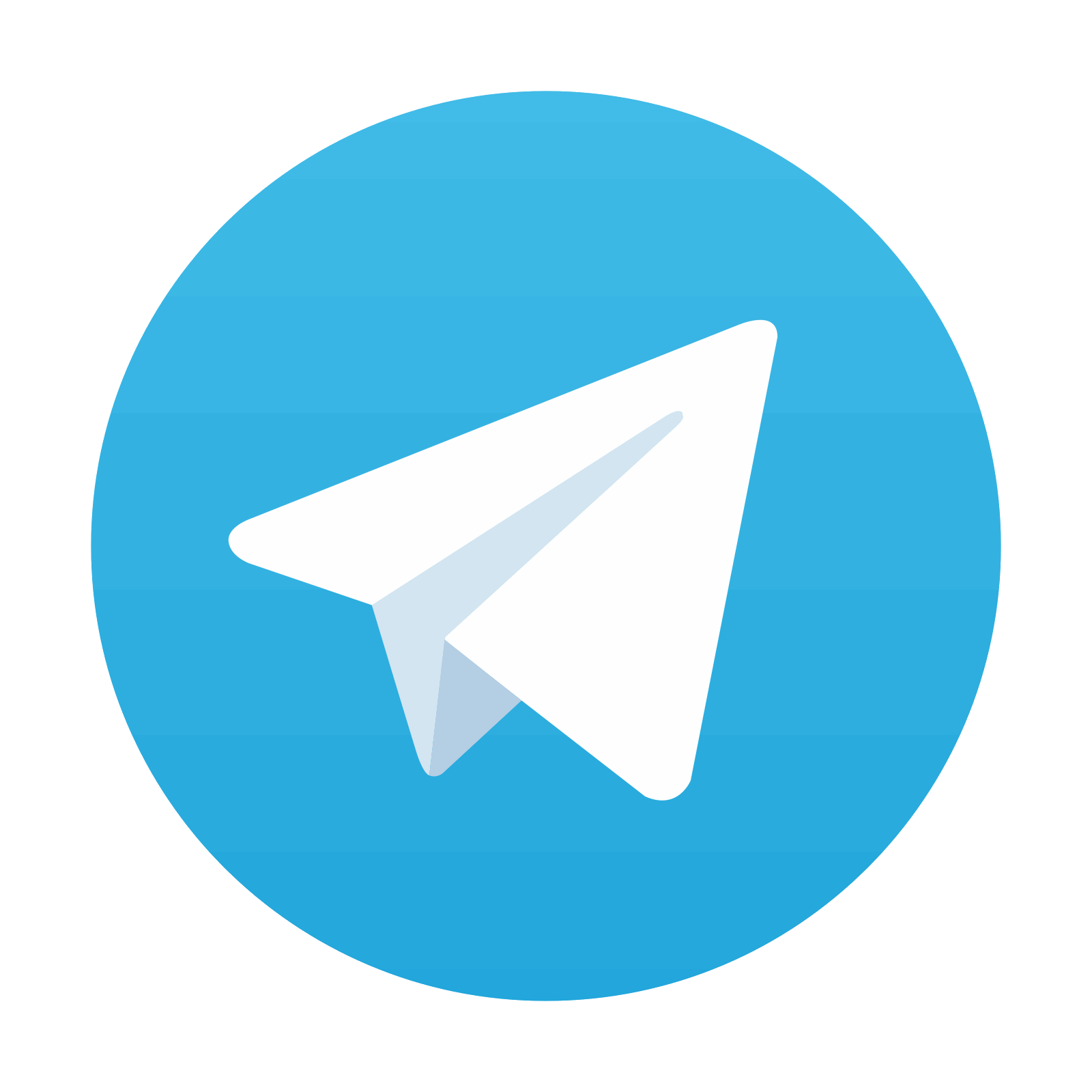
Stay updated, free articles. Join our Telegram channel

Full access? Get Clinical Tree
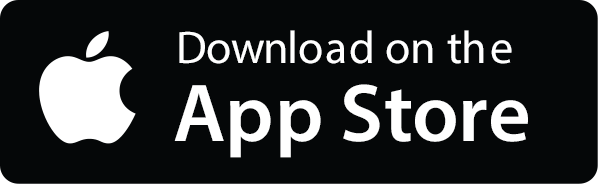
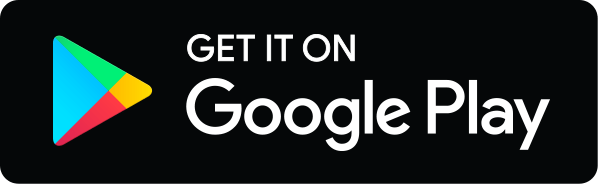