6.1 Fluid replacement therapy
Body fluid composition
Body fluids are separated into two main compartments, intracellular (ICF) and extracellular (ECF) fluid. ECF is further separated into intravascular and interstitial fluid. The proportion of body weight that is water falls from about 78% in a term newborn to 60% in adults (Table 6.1.1). Intracellular water accounts for about 40% of body weight. Intravascular fluid (plasma) accounts for only about 5% of body weight. Interstitial fluid is proportionately higher than intravascular fluid in infants, with an interstitial to plasma volume ratio of 5 : 1, compared with 3 : 1 in adults. Large changes in body weight over 24 hours or less usually reflect changes in total body water (TBW), because it takes a much longer period for substantial weight change due to growth or subcutaneous tissue wasting. In the first few days of life, there is a shift of water from ECF to ICF, accompanied by a 7% loss of TBW, so this is a very vulnerable period for dehydration with illness. Body fat contains only 20% water, so obesity implies a relative reduction in percentage of body weight as water. Conversely, malnourished children may have up to 80% of body weight as water. The clinical consequences are that:
• the severity of dehydration may be underestimated in obese children
• nutritional wasting is easily confused with dehydration as the clinical signs are similar
ECF has relatively high sodium and chloride content. ICF has high potassium, phosphate, magnesium and protein concentrations (Fig. 6.1.1). Small intestinal secretions are high in sodium, diarrhoea fluid is high in potassium, gastric fluid is high in chloride and pancreatic secretions are high in bicarbonate (Table 6.1.2).
Regulation of extracellular fluids
• Antidiuretic hormone (ADH) helps maintain intravascular volume and osmolality.
• In unwell children, ADH secretion may occur despite normal or low osmolality. This decreases water excretion, further decreasing plasma osmolality.
• This must be taken into consideration when considering the volume and composition of fluid replacement.
Oedema
Oedema is increased interstitial fluid and occurs by several mechanisms:
• increased hydrostatic pressure in the capillaries: venous obstruction, congestive heart failure
• decreased plasma oncotic pressure: hypoproteinaemic states such as nephrotic syndrome, protein-losing enteropathy, severe liver disease, and severe protein-energy malnutrition or kwashiorkor
• increased capillary permeability: this can occur locally from insect bites, or in one organ, such as cerebral oedema from traumatic brain injury, brain infection or other brain injury, or generally as in capillary leak syndrome from severe septicaemia.
Assessment of dehydration
Clinical history and examination
History
• Diarrhoea: duration, frequency, basic estimate of volume (small, large, profuse), presence of blood, mucus
• Vomiting: duration, frequency, volume, presence of bile or blood, whether projectile
• Eating and drinking: frequency, thirst, type of fluid intake (e.g. breast milk, oral rehydration solution, cordials, hypercaloric feed supplements)
• Urine output: frequency, number of wet nappies
• Associated symptoms: fever, cough, shortness of breath, abdominal pain, seizures, rashes, etc.
• Nutritional status: view the child’s growth chart including trend in values. Check skin folds on triceps and buttocks for presence of wasting. Also check weight for height, and height for age (low height for age in a malnourished child is stunting, which suggests chronic undernutrition).
Examination
• General condition: drowsy, restless or irritable
• Vital signs: temperature, pulse volume and heart rate, respiratory rate, blood pressure
• Eyes: sunken, no tears on crying
• Mouth and tongue: dry mucous membranes
• Skin: skin turgor reduced or central capillary refill time increased
• Muscle tone: weak, floppy or unable to hold head up
• Chest: deep acidotic breathing
The signs of dehydration are listed in Table 6.1.3. Precise estimation of percentage of dehydration is not possible using clinical signs, but an indication of the degree of dehydration, sufficient for formulating a plan for fluid management, is possible. Combinations of these signs are more specific than any individual sign. Clinical signs may be combined to classify a child as having mild (3–5%) dehydration (usually accompanied by few, if any, clinical signs), moderate (6–9%) dehydration, or severe (> 10%) dehydration.
Weight change
Clinical evidence on assessing dehydration
• A change in weight, where available, is the most accurate way to assess the degree of dehydration.
• Clinical signs will allow an estimation of dehydration, with combinations of signs performing better than individual signs.
• This classification is most reliable:
• Additional history and laboratory tests (e.g. bicarbonate) may be helpful in selected cases.
Fluid requirements
Before administering fluid replacement, five questions need to be addressed:
Reasons for giving fluid can be broadly grouped into:
3. What type of fluid should I use?
4. How much fluid should I give?
5. How will I monitor the child receiving fluid replacement?
Resuscitation for shock
Fluid resuscitation involves the rapid restoration of intravascular volume and is needed where shock is present. The clinical signs of shock are poor peripheral perfusion, cool pale extremities, tachycardia with low-volume pulses, low blood pressure, high blood lactate levels or large base deficit. Although hypotension is a sign of shock, this is usually a late sign in children; the absence of hypotension should not delay appropriate treatment. Children with more than one of these cardiovascular signs require intravenous fluid resuscitation. If an intravenous cannula cannot be inserted in a child needing fluid resuscitation, use the intraosseous route to the circulation. A volume of 20 mL/kg of an isotonic fluid should be administered as a bolus (i.e. run through as rapidly as possible). As this fluid will replace intravascular volume, its osmolality should be similar to that of plasma. Examples of appropriate fluids are: 0.9% sodium chloride (also known as normal saline) or Hartmann’s solution (also known as Ringer’s lactate solution) (Table 6.1.4). In severe septic shock in adults and in severe malaria in children, there is some evidence that outcomes are better when albumin is used compared with crystalloids. If there is no improvement, a further 20 mL/kg should be administered. Any child receiving fluid resuscitation should be monitored closely and reassessed immediately after giving the fluid. If signs of hypovolaemia persist despite two 20 mL/kg boluses of fluid, the child should be monitored in an intensive care unit. Check for signs that are suggestive of an underlying cause of shock (e.g. sepsis, cardiogenic, metabolic, diabetic ketoacidosis, intussusception, poisoning).
Replacing the fluid deficit
A patient’s fluid deficit refers to the degree of dehydration at the time of presentation (see assessment of dehydration above and Table 6.1.3). If shock is present, this should be corrected first as described above.
(e.g. 7% dehydration in a 12 kg child = 7 × 12 × 10 = 840 mL deficit).
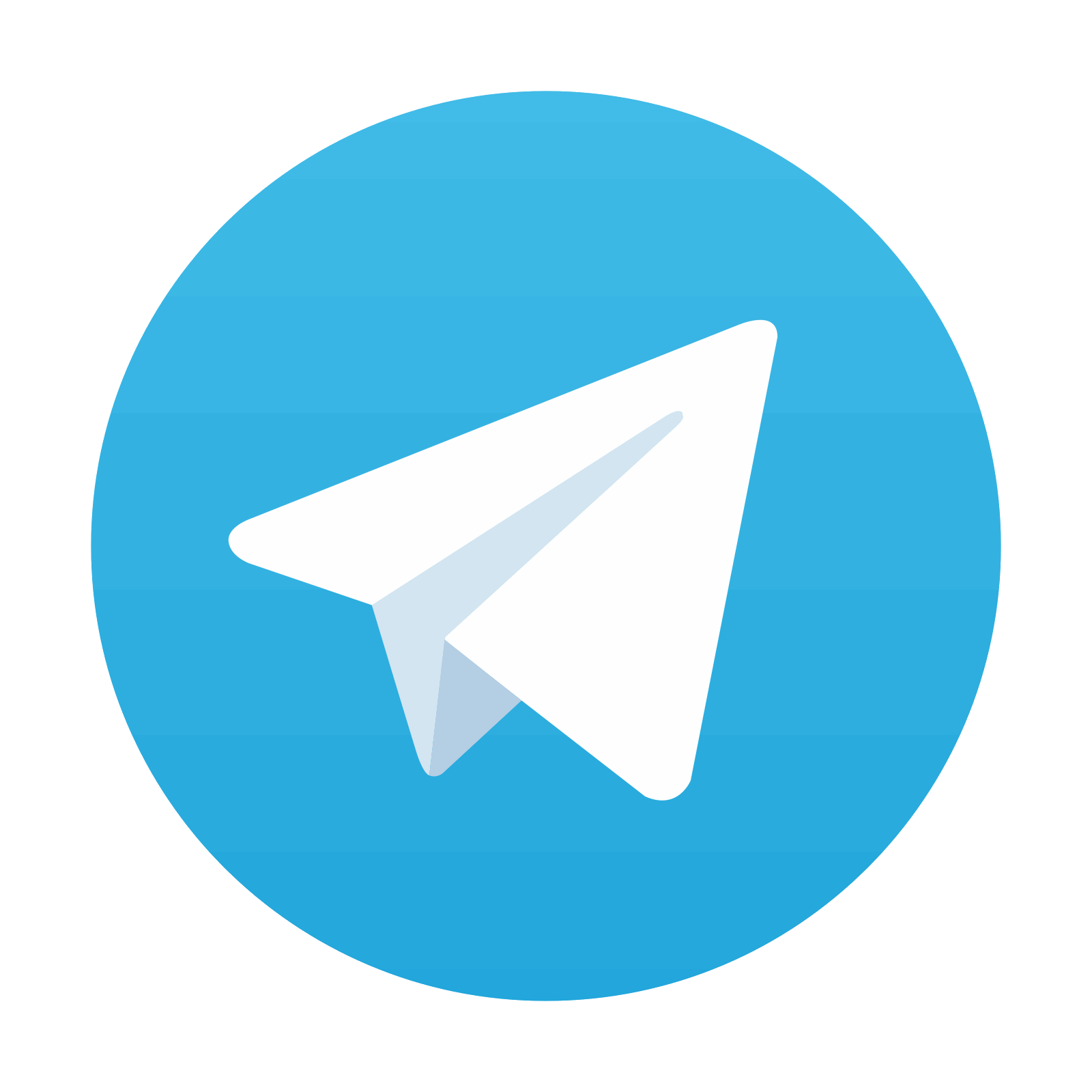
Stay updated, free articles. Join our Telegram channel

Full access? Get Clinical Tree
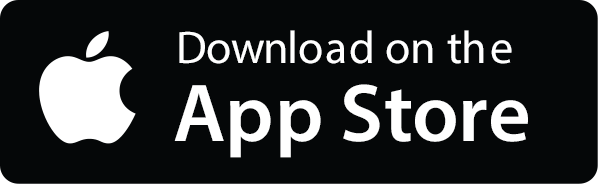
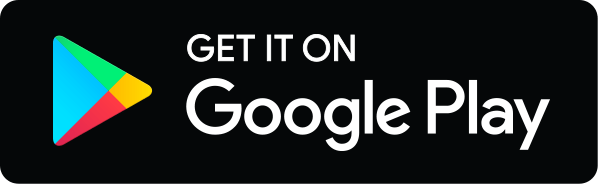