(5)
Division of Asthma, Allergy and Lung Biology, MRC Centre for Allergic Mechanisms for Asthma, King’s College London, London, UK
(6)
Newborn Unit, Neonatal Intensive Care Centre, King’s College London School of Medicine, King’s College Hospital, 4th Floor Golden Jubilee Wing, Denmark Hill, London, SE5 9RS, UK
Educational Aims
-
To describe methods of assessing fluid balance in prematurely born infants
-
To discuss the evidence for the type and magnitude of fluid given to prematurely born infants
Fluid balance in the sick, prematurely born must be carefully monitored; this includes hourly recording of all fluid input and output and at least daily weights. Prematurely born infants have a limited capacity to excrete a sodium load; sodium supplementation should be delayed until the onset of postnatal extracellular volume contraction. Colloid infusions should be used sparingly in sick, preterm infants; data from randomized trials have highlighted safer and at least equally effective alternatives, for example, in the treatment of hypotension. Fluid restriction in the perinatal period reduces morbidity.
25.1.1 Introduction
Fluid management of the prematurely born infant must reconcile the need to “grow the infant,” while too much fluid may increase morbidity including patent ductus arteriosus (PDA), necrotizing enterocolitis (NEC), and bronchopulmonary dysplasia (BPD); too little fluid may exacerbate electrolyte and metabolic disturbances, even acute renal failure. The sick, prematurely born infant’s fluid management is particularly complex for a number of reasons. Prematurely born infants have a high trans-epidermal water loss due to their skin immaturity and a large surface area to weight ratio; trans-epidermal water loss can be compounded if infants are nursed naked under a radiant warmer. In all infants, shortly after birth, there is a contraction of the extracellular compartment due to the loss of interstitial fluid, which is associated with postnatal weight loss (Modi et al. 2000). The timing of the diuresis/natriuresis, however, is related to the fall in the pulmonary vascular resistance, which may be variable in sick, prematurely born infants. Prematurely born infants have immature renal function, and in addition, respiratory water loss is influenced by the level of humidification of the inspired gases in ventilated infants. They may also be exposed to therapies that affect their fluid balance. For example, the majority of mothers of prematurely born infants will have been given antenatal corticosteroids in an attempt to improve lung immaturity, and antenatal corticosteroids may enhance fetal epithelial cell maturation and improve skin barrier function (Omar et al. 1999; Dimitriou et al. 2005). This results in lower insensible water loss and a higher urine output (Dimitriou et al. 2005). In this section, assessments of fluid balance are reviewed and the evidence for the type and magnitude of fluid given to prematurely born infants is discussed.
25.1.2 How to Assess Fluid Balance Status?
Fluid intake should be carefully recorded on an hourly basis, including all crystalloid and colloid input, plus medications and “flush” volumes. Urine output needs also to be accurately determined. One method is to collect urine output on open nappies, and for extremely low birth weight infants, cotton wool balls are placed on the nappy (Kavvadia et al. 2000a). As soon as the infant voids, the nappy or cotton wool ball should be weighed; otherwise, the volume may be underestimated due to losses from evaporation. An alternative method is to use a urine bag and aspirate urine with a fine catheter from the bag as soon as the urine is voided (Hartnoll et al. 2000). It is important, if the infant appears to be oliguric, to check that the bladder is not palpable. The magnitude of fluid input should be reduced if the infant develops acute renal failure defined as for any 24-h period a creatinine level greater than 132 μmol/l and urine output less than 1.0 ml/kg/h, except one day when a urine output of less than 0.5 ml/kg/h may indicate acute renal failure. Urine osmolality should also be measured; a level of between 200 and 400 mOsm/kg suggests a satisfactory fluid intake. Immature infants, however, have a limited ability to concentrate their urine and may pass urine of low osmolality while becoming dehydrated; hence, it is important to concurrently check the infant’s weight. Infants should be weighed on a daily basis, which is greatly facilitated by use of “bed” scales which remain inside the incubator and the infants are nursed upon them; hence, minimal handling is required to assess the weight.
Electrolytes and creatinine should be measured at least on a daily basis and more frequently if the infant is developing or has developed renal failure. Creatinine is derived from phosphocreatine in muscle and excreted in urine and is used as an index of the glomerular filtration rate (GFR). The serum level at birth reflects the maternal concentration. Creatinine clearance and the GFR increase with increasing postnatal age in premature infants (Gallini et al. 2000). Serum creatinine falls in the first week after birth as the maternally derived creatinine is excreted; therefore, failure to see the expected decline or a rise in the creatinine level indicates a reduced GFR.
25.1.3 Which Fluids?
Sodium supplementation should be delayed until the onset of postnatal extracellular volume contraction, which is indicated by marked weight loss (Modi et al. 2000). Prematurely born infants have a limited capacity to excrete a sodium load (Robillard et al. 1992), and in a randomized trial which included 25–30-week gestation infants, early (4 mmol/kg/day from the second day) compared to delayed (when weight loss of 6 % of birth weight had been achieved) supplementation was associated with delayed loss of body water (Hartnoll et al. 2000). In addition, by the end of the first week, 35 % of the babies in the delayed group but only 8.7 % of the early intake group no longer required supplementary oxygen (Hartnoll et al. 2000).
Colloid infusions should be used sparingly in sick, preterm infants, not least as there may be a safer, cheaper, and an at least equally effective alternative (Greenough 1998). In a randomized trial, isotonic saline and albumin were equally as effective in reducing the hematocrit in polycythemic infants (packed cell volume greater than 65 %) (Wong et al. 1997). Colloid infusions should not be used as first-line treatment for hypotension as several studies have demonstrated it is the volume infused rather than the protein load which is more efficacious in treating hypotension (Greenough 1998). In addition, in a randomized trial, isotonic saline was equally as effective as a similar volume of colloid in treating hypotension (So et al. 1997). Indeed, results highlight crystalloid may be more appropriate to treat hypovolemic hypotension, as the infants given albumin had significantly higher weight gain in the first 48 h, suggesting they suffered fluid retention (So et al. 1997). Albumin should not be given as part of the initial resuscitation process, as low Apgar scores may indicate myocardial depression in which case volume load is contraindicated. Asphyxia also results in increased capillary permeability and protein leak into the lung could impair resuscitation (Jobe et al. 1985). Furthermore, we have demonstrated that the amount of colloid infused in the perinatal period significantly correlated with the duration of oxygen dependency (Kavvadia et al. 2000a) and abnormal perinatal lung function (Marks et al. 1978). Colloid was prescribed to hypotensive infants (Kavvadia et al. 2000a) and thus it might be that the adverse outcomes were related to the severity of the underlying illness rather than colloid infusion per se. An alternative explanation, however, is that when colloid is given to infants with increased vascular permeability, it leaks into the lungs, worsening respiratory function (Marks et al. 1978) and necessitating higher levels of respiratory support, and hence could predispose to BPD. There are also no data to support the use of albumin infusion to “treat” low serum albumin levels. Two randomized, but small trials have been performed with no differences in mortality or morbidity (Jardine et al. 2010). In one of the studies (Greenough et al. 1993), albumin infusion was associated with a significant increase in the albumin level and a reduction in weight, but no significant changes in the peak inflating pressures.
25.1.4 How Much Fluid?
Excessive fluid input increases the likelihood of PDA and BPD development. In a retrospective analysis of data from 1,382 ELBW infants of birth weight between 401 and 1,080 g, multivariate logistic regression demonstrated that higher fluid in the infant and less weight loss during the first 10 days were significantly related to death or BPD (Oh et al. 2005). Yet administration of diuretics to infants with RDS results in no long-term benefits and only transient improvements in lung function (Brion and Soll 2011). An alternative approach has been to use fluid restriction to try and prevent morbidity and mortality in prematurely born infants. There has been a number of randomized trials comparing restricted versus liberal water intake. Meta-analysis of five such studies (Bell and Acarregui 2008) demonstrated that restricted water intake increased postnatal weight loss and significantly reduced the risks of PDA and necrotizing enterocolitis (NEC), but not BPD and intracranial hemorrhage (ICH). In only one of the studies, however, were antenatal steroids and postnatal surfactant given routinely (Kavvadia et al. 2000a). That study (Kavvadia et al. 2000a) demonstrated no statistically significant differences in PDA, ICH, NEC, or BPD, but significantly more (43 % versus 19 %) of the liberal versus restricted group received postnatal corticosteroids, as it was considered they were at high risk of developing BPD. Importantly, there were no statistically significant differences in the incidence of acute renal failure (Kavvadia et al. 2000a) or electrolyte or metabolic disturbances (Kavvadia et al. 2000b). Those results (Kavvadia et al. 2000a, b) support the conclusion in the Cochrane review (Bell and Acarregui 2008) that the most prudent prescription for water intake of prematurely born infants is careful restriction of water intake so that physiological needs are met without allowing significant dehydration. Whether fluid restriction is beneficial in specific situations remains to be appropriately tested. One of three Cochrane reviews at the protocol stage has now been reported and demonstrated no eligible trials.
Essential to Remember
-
In the perinatal period, prematurely born sick infants should be fluid restricted, but ensuring their physiological needs are met without significant dehydration.
-
Sodium supplementation should be delayed until the onset of postnatal extracellular volume contraction, which is indicated by marked weight loss.
-
Colloid infusions should be used sparingly in sick, preterm infants.
Future Perspectives
-
More accurate techniques to assess fluid balance in extremely low birth weight infants need to be devised.
-
Further studies are required to determine the most appropriate amounts of fluid input in the present population of extremely low birth weight infants.
25.2 In the Pediatric Patient
Stacey Valentine7 and Adrienne G. Randolph8
(7)
Division of Pediatric Critical Care, Department of Pediatrics, University of Massachusetts Children’s Medical Center, Worcester, MA, USA
(8)
Department of Anesthesia, Children’s Hospital, Boston, MA, USA
Educational Aims
-
To understand the pathophysiology of fluid transport in the healthy and injured lung
-
To understand the implications of fluid management in the mechanically ventilated child with respiratory failure
-
To identify the key outcomes of fluid overload in patients at risk for and with acute lung injury
-
To identify the key differences in fluid management in acute lung injury with and without shock
-
To understand how the type of fluid administered may impact clinical outcomes
25.2.1 Fluid Management in the Mechanically Ventilated Patient
The challenge of fluid management in critically ill pediatric patients with respiratory failure is to maintain intravascular volume to ensure adequate end-organ perfusion while minimizing extravascular lung water and subsequent pulmonary edema (Ware and Matthay 2000; Wiedemann et al. 2006; Calfee and Matthay 2007). Mechanically ventilated pediatric patients are at risk for developing acute lung injury (ALI) and its more severe form, acute respiratory distress syndrome (ARDS). Evidence for fluid management in patients with respiratory failure is extrapolated from research on fluid management in acute lung injury. Over the past 30 years, clinical evidence has indicated that positive fluid balance is associated with worsened clinical outcomes in patients with acute lung injury (ALI) and acute respiratory distress syndrome (ARDS) (Ware and Matthay 2000; Wiedemann et al. 2006; Calfee and Matthay 2007; Zimmerman et al. 2009; Ahmed et al. 2009; Thakur et al. 2010; McIntyre et al. 2000; Luce 1998; Schuster 1993). Both ALI and its more severe form, ARDS, are the result of a large-scale pulmonary inflammatory response, leading to hypoxemia and respiratory failure. The common pathway of this inflammatory response is diffuse alveolar damage, resulting in pulmonary edema secondary to a pulmonary endothelium and epithelium that is more permeable to water and proteins (Ware and Matthay 2000). Prevention of ALI and ARDS is key in the management of mechanically ventilated children. The major risk factor identified in pediatric patients developing ALI is severe sepsis with pneumonia as a source (Zimmerman et al. 2009). Research on prevention of ALI is sparse, with early identification tools currently under way; the focus of research in ALI and ARDS has been on understanding the pathophysiology of the disease and supportive management (Ahmed et al. 2009; Thakur et al. 2010). The challenge of fluid management in critically ill patients with respiratory failure is to continually assess the patient’s intravascular volume status and carefully titrate fluid management to maintain adequate perfusion. Once reached, it is important to carefully restrict all fluids administered to the patient, including all intravenous infusion, antibiotics, and flushes. This has led to intense debate in the optimal fluid management of patients both to prevent and to treat ALI.
Studies with pulmonary biopsies of patients with ARDS have demonstrated that interstitial and alveolar edema occur early when inflammatory cells are present in the interstitium (Ware and Matthay 2000). Animal models of ALI have suggested that lower cardiac filling pressures and intravascular pressures can limit extravascular lung water accumulation (Craig et al. 2010; Folkesson and Matthay 2006; West 2000).Patients with lower pulmonary artery occlusion pressures (PAOP) have been found to have significantly improved survival than those with higher PAOP, while studies lowering intravascular hydrostatic pressures have been shown to reduce pulmonary edema (West 2000; Ware and Matthay 2005; Schuster and Haller 1990; Sakka et al. 2002; Berkowitz et al. 2008; Cheng et al. 2005). Until 2006, fluid management was guided by observational studies and small randomized controlled trials, supporting the notion that a restrictive fluid strategy improves survival in patients with ARDS (Wiedemann et al. 2006). In 2006, the ARDS Network found a significant reduction in the duration of mechanical ventilation (p < 0.001), length of stay (LOS) in the intensive care unit (p < 0.001), and oxygenation index in patients with ALI exposed to a conservative versus liberal fluid strategy (Wiedemann et al. 2006). However, optimizing fluid management in patients with lung injury is challenging, given that ALI and ARDS are known to occur in states of sepsis and shock. The major goal of fluid management in patients with ALI and ARDS is to adequately fluid-resuscitate the patient in shock but aggressively fluid-restrict and diurese the patient when out of shock.
25.2.2 Pathophysiology
Understanding the physiology of normal fluid clearance in the lung is crucial to managing a patient’s fluid status with respiratory failure (Ware and Matthay 2005; Ware 2006). Normal alveolar epithelium is composed of two types of cells, flat type I cells, which make up 90 % of the alveolar surface area, and cuboidal type II cells, which make up the remaining 10 %. Type II cells regulate ion transport, produce surfactant, and are responsible for further proliferation of type I cells (Ware and Matthay 2005). Alveolar fluid clearance across the alveolar-capillary membrane is an active process. The driving force for alveolar fluid clearance is the active transport of sodium and chloride across the alveolar epithelium and occurs primarily by ATII cells (Folkesson and Matthay 2006). Sodium uptake occurs on the apical surface through amiloride-sensitive channels and is then pumped actively from the basolateral surface into the lung interstitium by the Na-K-ATPase (Folkesson and Matthay 2006; West 2000; Ware and Matthay 2005; Schuster and Haller 1990). This fluid follows the sodium gradient by a simple osmotic mechanism, partially mediated by aquaporins. Tight junctions sustain the apical and basolateral cell polarity, thereby regulating fluid permeability (Sakka et al. 2002).
Fluid accumulates in the alveolar space when this protective mechanism fails, either by overwhelming the active reabsorption mechanism or injuring the capillary-alveolar membrane. Increased fluid accumulation secondary to a dysfunctional reabsorption mechanism can be caused by an increase in pulmonary capillary pressure or rapid shifts in thoracic pressure. Increased permeability of the capillary-alveolar barrier is commonly a result of overwhelming inflammation. Injury to the lung parenchyma, either by direct injury or indirect injury by a systemic process leading to pro-inflammatory and cytokine release, causes disruption of the tight junctions that regulate fluid movement, resulting in unregulated leakage of fluid and protein into the interstitium and alveolar space (West 2000; Ware and Matthay 2005; Schuster and Haller 1990; Sakka et al. 2002; Berkowitz et al. 2008).
25.2.3 Measuring Outcomes of Fluid Management in the Mechanically Ventilated Patient
Fluid management is crucial in the management of mechanically ventilated patients. However, measuring the outcomes of fluid management in respiratory failure can be challenging. Outcome measures include direct physiologic measurements of fluid balance in the lung, i.e. measuring extravascular lung water (EVLW) (Schuster 1993; Sakka et al. 2002; Berkowitz et al. 2008) and clinical outcome measures. Determining the target for EVLW is difficult to generalize without the clinical context (Schuster 1993). Clinical measures of fluid management in the patients with respiratory failure include duration of mechanical ventilation, measured as ventilator-free days at day 28; oxygenation index and PaO2/FiO2 ratio; and measures of non-pulmonary organ function, measured as organ-failure-free days at day 28 (Wiedemann et al. 2006). Many of these measures were used in the early clinical trials to demonstrate improved clinical outcomes with a restrictive fluid management and are summarized in Table 25.1.
Table 25.1
Summary of clinical trials in fluid management
Year |
Author |
Entry criteria |
# pts |
Fluid targets |
Outcomes |
Independent predictor of mortality |
---|---|---|---|---|---|---|
Protocol group |
Protocol/control (p-value) | |||||
Simmons et al. |
ARDS |
111 adults |
<3 kg weight gain day 14 |
Survival
67/0 % |
N/A | |
Schuller et al. |
ARDS |
36 adults |
<1L (+) balance 36 h |
Survival
74/50 % p = 0.05 |
Yes | |
Humphrey et al. |
ARDS |
40 adults |
25 % reduction of Ppw |
Survival
75/29 % p < 0.02 |
Yes | |
Alsous et al. |
ARDS |
36 adults |
Negative fluid balance in first 3 days after admission |
Survival
100/31 %
RR 5.0
95 % CI 2.3–10.9 |
Yes | |
Sakr et al. |
ALI/ARDS |
393 adults |
Fluid balance 96 h (L)
Survivors/non-survivors |
Fluid balance
Survivors/non-survivors
1.4 ± 6.5/3.9 ± 7.8 p = 0.001 |
Yes | |
Wiedemann et al. |
ARDS |
1,000 adults |
Conservative fluidb vs. liberal fluidb protocol |
Mortality
25.5/28.4% p = 0.30
VFDs
14.6 ± 0.5/12.1 ± 0.5 p < 0.001 |
No | |
Rosenberg et al. |
ARDS |
844 adults |
(−) Fluid balance day 4 |
Mortality OR
0.50 95 % CI 0.28–0.89 p < 0.001
cVFDs
15 + 11/10 + 11 p = 0.001 |
Yes | |
Murphy et al. |
ALI in septic shock |
212 adults |
AIFR and CLFMa
CLFMa
AIFRa
Neithera |
Mortality
18.3 %
41.9 %
56.6 %
77.1 %
p < 0.001 |
Yes | |
Stewart et al. |
ARDS |
244 adults |
Conservativeb vs. restrictive protocolb |
Mortality
25 %
28 %
p = 0.3
cVFDs
15.1 ± 1/13.1 ± 1
p = 0.04 |
No |

Full access? Get Clinical Tree
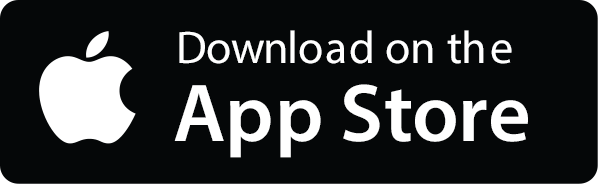
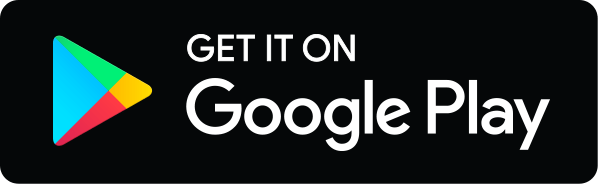