FETAL HEART RATE MONITORING
Key Points
The goals for performance of fetal monitoring are to
• Decrease risk for stillbirth in the antepartum population
• Assess the physiologic stresses of labor on the metabolic status of the fetus
• Identify at-risk fetuses before permanent damage occurs, so that therapeutic interventions can be used to decrease complication rates
Background
The idea that abnormal fetal heart rates correlate with fetal distress was initially proposed by Kilian in 1848 (1). Since its inception, the goal of fetal heart rate monitoring (FHRM) has been early identification of fetuses at risk for hypoxic insult. FHRM now occurs nearly universally during the intrapartum period and in selected patients at high risk for stillbirth in the antepartum population.
• Retrospective studies have confirmed the safety of FHRM.
• Prospective trials have shown a decreased perinatal mortality rate associated with electronic FHRM (2–4).
• Despite improved perinatal mortality, the goal of reducing fetal asphyxia and brain damage remains elusive.
• The benefit of electronic FHRM is that it is very reliable in prediction of normal fetal acid–base status (it has a high specificity) when the pattern is normal or indeterminate:
• Normal FHRM patterns have a positive predictive value of approximately 99% for the absence of fetal acidosis.
• Electronic FHRM is of limited utility in that its sensitivity (the ability to identify abnormal fetuses) is poor when there is an abnormal pattern. Abnormal FHRM monitoring patterns have poor positive predictive value for the presence of significant fetal acidosis (5). Standardized guidelines for interpretation of fetal heart rate patterns and estimation of the risk for acidosis have been adopted by the National Institute of Child Health and Human Development to help identify the subset of patients at highest risk for the development of metabolic acidosis (6).
Epidemiology
• Electronic fetal monitoring is used in two settings:
• Intrapartum monitoring for the development of clinically significant fetal hypoxia/ acidosis during labor
• Antepartum monitoring for pregnancies deemed high risk because of maternal or fetal complications
• When intrapartum electronic fetal monitoring is used, the perinatal death rate decreases (7).
• Antepartum use of FHRM combined with evaluation of amniotic fluid volume decreases the stillbirth rate in populations at higher than average risk for stillbirth (women with chronic medical conditions, presence of intraamniotic infection, fetal congenital anomalies, and fetal intrauterine growth retardation) (8).
• Several retrospective studies published during the 1970s associated the use of electronic FHRM with an observed decrease in the fetal death rate (9–12). Other randomized controlled trials during the same era did not show similar benefit (13,14). In previously unmonitored populations with high perinatal mortality rates, randomized studies of electronic fetal monitoring confirmed a decrease in perinatal mortality (2). Using data from 1,732,211 pregnancies recorded in the US 2004 birth cohort–linked birth/infant death data set, the use of EFM was associated with a significant decrease in the risk for perinatal mortality (3). However, it should also be noted that electronic fetal monitoring has a high false-positive rate for identification of clinically significant fetal stress, which increases the incidence of interventions such as cesarean delivery or operative vaginal delivery (15). The percentage of these operative interventions that actually improve neonatal outcome is uncertain.
Physiologic Basis of Fetal Heart Rate Monitoring
• External monitoring
• The external fetal monitoring system consists of a transmitter that emits ultrasound waves that penetrate the uterine cavity and encounter interfaces of differing density.
• When the interfaces are moving, as the fetal heart chambers do with each cardiac cycle, the reflected signals undergo a Doppler shift, which is measured by the receiving device. The highest Doppler shift of the returning waveform passes to a cardiotachometer (Fig. 32-1).
• Fetal movement can also cause Doppler shifts and must be filtered out of external monitoring.
• Internal monitoring
• Internal FHRM is accomplished by attaching an electrode to the fetal scalp.
• The signal generated by electrical activity of the heart is acquired by the electrode, amplified, and transmitted to a cardiotachometer (Fig. 32-2).
• The cardiotachometer counts the interval between the R waves of the fetal electrocardiogram (ECG) and displays fetal heart rate as the reciprocal of this interval. A new rate is set with the transmission of each R wave, and the tiny differences in arrival intervals between R waves are recorded. These small, instantaneous differences are known as beat-to-beat variability and can be measured accurately only by internal monitoring systems.
• If the fetal R–R interval is shorter than 250 milliseconds (i.e., the heart rate exceeds 240 beats per minute [bpm]), the cardiotachometer cannot differentiate between arriving signals and will either divide the true rate in half or not register it at all.
• Fetal movement does not affect internal FHRM.
• Uterine contractions
• Assessment of uterine contraction patterns is necessary to determine the pattern of any accelerations and decelerations in the fetal heart rate.
• External acquisition of uterine contractile activity is obtained using a transducer, which acts as a strain gauge and senses changes in uterine shape and rigidity. When the transducer’s plunger is depressed, an electrical signal is emitted and recorded on the tracing paper as an upward deflection, indicating a uterine contraction. The external transducer does not sense true intrauterine pressure, and the early stages of a contraction may not depress the plunger enough to record small changes in uterine pressure.
• Internal intrauterine catheters are open-ended, fluid-filled catheters that measure the intrauterine pressure as the difference in hydrostatic pressure present between the closed intrauterine space and the external environment.
• Maternal–fetal nutrient exchange
• Eighty-five percent of the uterine blood flow supplies the fetoplacental circulation, while the remaining 15% nourishes the myometrial wall.
• Oxygen crosses the placental barrier by simple diffusion and is more avidly bound to fetal hemoglobin than to maternal hemoglobin.
• During a normal uterine contraction, there is a short interruption of blood flow through the intervillous space when the pressure generated by the myometrial contraction exceeds the blood pressure in the spiral arterioles and the intervillous space adjacent to the placenta. If the contractions are prolonged or tetanic (16), the interruption of placental perfusion may be prolonged and fetal oxygen reserves may be exceeded, leading to fetal hypoxia.
• Any factor that decreases uterine blood flow or that affects oxygen diffusion may decrease the amount of oxygen delivered to the fetus.
Maternal position (17), exercise, hypotension, or chronic maternal medical conditions such as hypertension and diabetes may be associated with decreased placental blood flow.
Placental infarcts or premature placental separation may impair oxygen delivery to the fetus.
• Neurologic control of fetal heart rate
• The average baseline fetal heart rate is about 160 bpm in the early second trimester. An atrial pacemaker determines the baseline fetal heart rate.
• Opposing effects of parasympathetic and sympathetic innervation of the heart modulate variations in this rate. Initially, the fetal heart rate is predominantly regulated by the sympathetic nervous system and chemoreceptors. As the nervous system matures, parasympathetic regulation becomes more pronounced. This results in a decrease in average baseline heart rate and an increase in its variability.
Parasympathetic nerve impulses arise in the brainstem and reach the heart via the vagus nerve.
Sympathetic stimuli are carried from the brainstem to the heart via cervical sympathetic nerve fibers.
Parasympathetic tone primarily affects the short-term beat-to-beat variability, whereas sympathetic stimulation is the strongest determinant of long-term variability and accelerations in heart rate (18).
Even combination autonomic blockade may not abolish all variability, which suggests that unknown, nonautonomic neural factors may play a role in fetal heart rate variability (19).
• Parasympathetic tone increases as gestation advances, which gradually decreases the baseline fetal heart rate.
• Transient loss of variability may result from infection, drugs, hypoxia, or sleep cycles.
• Prematurity is also associated with decreased variability, but by 28 weeks of gestation, the neurologic pathways stimulating variability are functional. Consequently, it should be kept in mind that a persistent absence of variability beyond 28 weeks of gestation occurs less than 15% of the time. Such a finding may indicate the occurrence of a significant insult to the fetal central nervous system.
Figure 32-1. Schematic diagram of an indirect fetal monitoring system. The fetal heart rate (FHR) is obtained using Doppler techniques and then counted by a cardiotachometer. Uterine activity is measured by tocodynamometry. (UC, uterine contraction.) (Reproduced with permission from Berkowitz KM, Nageotte M. Intrapartum fetal monitoring. In: Moore T, et al., eds. Gynecology and obstetrics: a longitudinal approach. New York: Churchill-Livingstone, 1993:568.)
Figure 32-2. Schematic diagram of a direct fetal monitoring system depicting internal fetal scalp electrode and intrauterine pressure catheter. (FHR, fetal heart rate; UC, uterine contraction.) (Reproduced with permission from Berkowitz KM, Nageotte M. Intrapartum fetal monitoring. In: Moore T, et al., eds. Gynecology and obstetrics: a longitudinal approach. New York: Churchill-Livingstone, 1993:569.)
Definitions of Terms
The process of fetal assessment requires definition of fetal heart rate baseline, reactivity, variability, and the presence and patterns of accelerations and decelerations. The analysis of these characteristics is then interpreted in the context of the setting in which the testing is occurring. In antepartum testing situations, the FHR pattern is assessed for a fixed time interval and interpreted as normal or abnormal assessment of fetal acid–base and oxygenation status. In the case of intrapartum FHR monitoring, the FHR pattern is evaluated continuously and categorized as normal (Category 1, Fig. 32-3), indeterminate (Category 2, Fig. 32-4), or abnormal (Category 3, Fig. 32-5) (6). Several factors must be considered in order to properly characterize an FHR pattern.
• Fetal heart rate baseline.
• The National Institute for Child Health and Human Development defines baseline fetal heart rate as “the approximated mean fetal heart rate, rounded to increments of 5 bpm during a 10-minute window, excluding periodic or episodic changes, periods of marked fetal heart rate variability, or segments of the baseline that differ by greater than 25 bpm” (20). A normal fetal heart rate baseline is 110 to 160 bpm.
• Fetal heart rate reactivity.
• Fetal heart rate reactivity is two or more accelerations in a 20-minute period (21).
• The presence of accelerations and reactivity indicates the presence of an intact and functioning fetal central nervous system and the existence of normal intrauterine acid–base and oxygenation conditions.
• Variability.
• Baseline variability is defined as fluctuations from baseline fetal heart rate of two cycles or more per minute (22).
• Beat-to-beat variability is the small, instantaneous differences in the R–R interval:
It is literally the difference in the FHR baseline from one beat to the next beat.
Beat-to-beat variability can only be measured accurately by internal monitoring systems.
• Long-term variability refers to state changes induced by alternating periods of fetal sleep and activity.
• Gradations of variability include undetectable, minimal, moderate, marked, and sinusoidal.
• Accelerations (see Fig. 32-3).
• Accelerations are elevations of fetal heart rate at least 15 bpm above baseline, lasting at least 15 seconds.
• Accelerations are typically abrupt in onset and are associated with fetal movements.
• Any factor that suppresses central nervous system activity (sleep, drugs, acidosis, etc.) can also suppress accelerations and reactivity.
• Decelerations: Episodic patterns of decelerations are not associated with uterine contractions. Periodic patterns of deceleration occur in association with uterine contractile activity.
• Early decelerations (Fig. 32-6)
Early decelerations are secondary to fetal head compression and are benign. They can be seen during labor in Category 1 FHR patterns.
Early decelerations are uniformly shaped decelerations of minimal amplitude, have a slow onset and slow return to baseline, and are in phase with the uterine contraction cycle. The time from onset of deceleration to nadir is typically greater than 30 seconds.
An early deceleration begins early in the contraction cycle, drops to its lowest point with the peak of the contraction force, and returns to baseline before the contraction ceases.
The amplitude of the deceleration roughly parallels the strength of the contraction but rarely exceeds 30 bpm below the baseline. The nadir of the contraction occurs with the peak of the contraction.
• Variable decelerations (Figs. 32-4 and 32-5)
Variable decelerations are V-shaped decelerations associated with umbilical cord compression and reduction in blood flow through the umbilical vein. During labor, variable decelerations are features of Category 2 or Category 3 FHR patterns.
Variable decelerations have an abrupt onset and quick return to baseline. The duration, intensity, and timing of each variable deceleration relative to its contraction may differ markedly. The onset of the deceleration to the nadir occurs within 30 seconds. The deceleration itself lasts more than 15 seconds but less than 2 full minutes. Accelerations occurring just prior to the onset of the decelerations are commonly referred to as “shoulders” and are not part of the variable deceleration.
• Late decelerations (Fig. 32-7)
Late decelerations are named for the timing of their onset and cessations. The decrease in fetal heart rate occurs after the start of a uterine contraction, and the return to baseline is delayed until after the end of the contraction.
The onset of a late deceleration occurs up to 30 seconds after the start of a contraction, and its nadir occurs well after peak contraction strength has been reached.
The decreased fetal heart rate continues after the contraction has ended, with return to baseline delayed for up to 30 seconds.
• Normal, indeterminate, and abnormal fetal heart rate patterns.
• The FHR pattern is categorized by assessment of the following four variables:
Baseline fetal heart rate
Presence of accelerations or decelerations
Frequency, type, and severity of decelerations
Presence or absence of variability
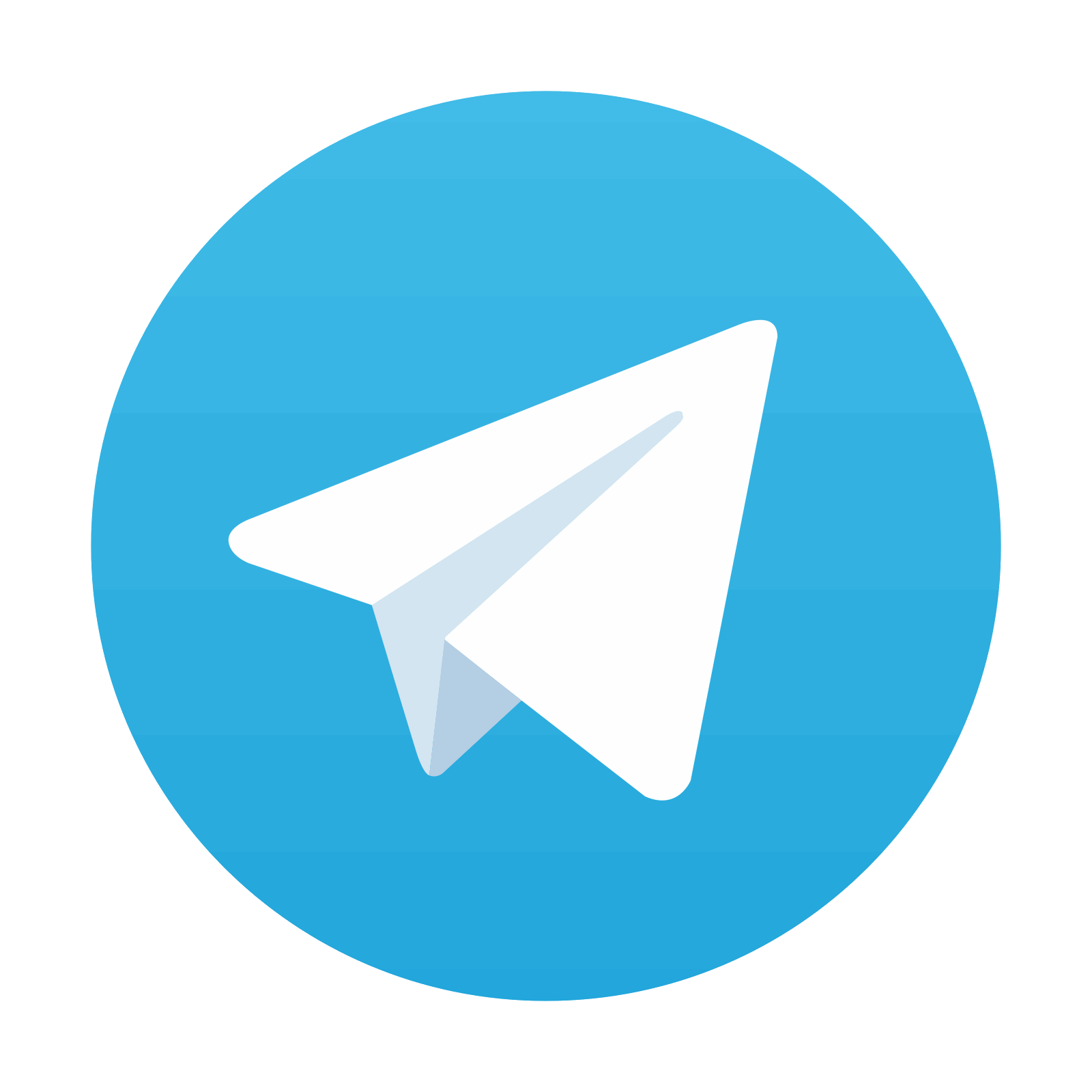
Stay updated, free articles. Join our Telegram channel

Full access? Get Clinical Tree
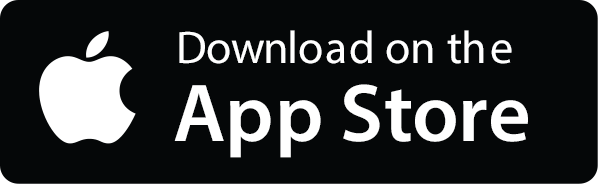
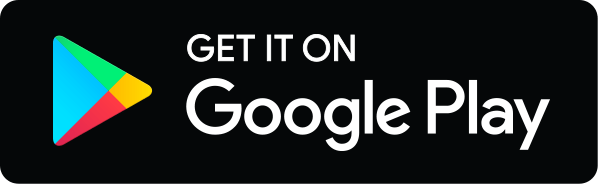