-
Chapter Contents
-
Introduction and definitions 175
-
Incidence and recurrence rate 176
-
The aetiology of IUGR 177
-
Physiological sequelae of IUGR 179
-
The consequences of IUGR 180
-
Primary prevention of IUGR 181
-
Antenatal screening and diagnosis 181
-
Management of IUGR and timing of delivery 183
-
Fetal therapy for IUGR 183
Introduction and definitions
A fetus is defined, antenatally, as small for gestational age (SGA) when its estimated fetal weight (EFW) is below a specified percentile for its gestation, for a given population. Thus, SGA is a statistical concept, based on the distribution of EFW within a population. The World Health Organization (WHO) has chosen the 10th percentile as an arbitrary threshold for identifying SGA ( ). Another commonly used threshold is the total population mean EFW minus two standard deviations of a population-based growth curve (i.e. setting the normal range for growth as roughly between the 2.5th and the 97.5th percentile) ( ). Babies defined as SGA using the 10th percentile threshold have a higher rate of adverse outcome than average-for-gestational-age babies ( ; ). However, not all fetuses with SGA have an abnormal pattern of growth. In reality, up to 70% of fetuses identified as SGA are ‘constitutionally small’ and healthy, while the remainder have intrauterine growth restriction (IUGR) with abnormal growth velocity and increased risk of adverse outcome ( ; ; ).
A fetus with IUGR is one that has failed to achieve its genetically determined growth potential, owing to one or more pathological factors ( Table 10.1 ). In comparison with a normally grown fetus with no structural or chromosomal defect, an IUGR fetus has at least a 10-fold increased risk of perinatal mortality ( ; ). In the developed world, IUGR is the commonest cause of stillbirth and the second leading cause of perinatal mortality, after preterm birth. A significant proportion of IUGR fetuses are not detected before birth and recent reports show that undiagnosed IUGR was a factor in over 50% of stillbirths in the UK ( ).
Fetal | Chromosomal anomaly : triploidy, trisomies (21,18,13), Turner syndrome, uniparental disomy, confined placental mosaicism |
Structural malformation : cardiac malformations, neural tube defects, gastroschisis, diaphragmatic hernia, renal agenesis, multiple malformations | |
Genetic abnormalities and syndromes : Bloom, Brachmann–de Lange, Cornelia de Lange, De Sanctis–Cachione, Donohue, Dubowitz, Fanconi, Johanson–Blizzard, Mulibrey nanism, osteochondrodysplasias, Prader–Willi, Roberts, Russell–Silver, Rubenstein–Taybi, Seckel, Williams–Beuren | |
Fetal infections : cytomegalovirus, toxoplasmosis, rubella, malaria, human immunodeficiency virus, varicella-zoster, herpes simplex, Epstein–Barr virus, congenital tertiary syphilis | |
Multiple pregnancies : monochorionic gestation, twin-to-twin transfusion | |
Maternal | Past obstetric history : previous intrauterine growth restriction, stillbirth, preterm birth |
Social/environmental/nutritional : extreme reproductive age, short interpregnancy interval, poor diet, anorexia nervosa, body mass index <20, active inflammatory bowel disease, smoking, substance abuse (alcohol, cocaine, opiates, amphetamines), self-neglect and/or psychiatric illness, medication (β-blockers, overtreatment of hypertension), high altitude | |
Vasculopathy : chronic hypertension, pre-eclampsia, diabetes mellitus, sickle-cell disease, antiphospholipid syndrome, systemic lupus erythematosus | |
Renal disease : glomerulonephritis, lupus nephritis, chronic renal failure | |
Thrombophilia : factor V Leiden G1691A, prothrombin G20210A | |
Hypoxia : severe anaemia, chronic lung disease, severe asthma, cyanotic heart disease | |
Reduced plasma volume expansion | |
Placenta | Abnormalities of the umbilical cord : single umbilical artery, velamentous insertion of the cord, overcoiled umbilical cord |
Tumours : chorioangiomatosis, placental mesenchymal dysplasia | |
Vascular : vascular malformations, fetal thrombotic vasculopathy, placental abruption | |
Abnormal placental development : abnormal trophoblast invasion, recurrent bleeding, placenta praevia, circumvallate placenta, confined placental mosaicism | |
Uterine | Uterine abnormality, uterine fibroids, assisted reproductive techniques |
Endocrine factors | Vascular endothelial growth factor, placental growth factor, fms-like tyrosine kinase 1, pregnancy-associated plasma protein A, insulin-like growth factor |
There are many reasons why IUGR poses a major challenge to clinicians and researchers. The aetiology of IUGR is heterogeneous with multiple causal pathways, and there is no proven therapy. The most effective intervention is delivery, thus many clinicians advocate early intervention for suspected IUGR. However, it is important to distinguish between a constitutionally small fetus from one that is IUGR, in order to avoid iatrogenic morbidity to the mother and baby due to unnecessary operative and/or preterm delivery. Central to this problem is the lack of standard definitions or uniform diagnostic criteria for IUGR. Consequently, many researchers have used the definitions for SGA and IUGR interchangeably, and used different thresholds to identify the normal range for growth. This has led to classification bias and difficulties in interpreting findings from IUGR studies. In essence, the use of a 10th percentile threshold alone fails to identify IUGR fetuses with abnormal growth pattern but whose EFW is above the threshold value ( ).
To improve the identification of SGA fetuses with adverse outcomes, the 10th percentile threshold of a ‘standard’ or ‘healthy obstetric population’ with low-risk pregnancies and normal perinatal outcome should be used instead of a growth curve based on the entire obstetric population ( ). In addition, since IUGR is more common in babies born preterm, the growth standard should be ultrasound-based fetal weight reference rather than neonatal birthweight reference ( ). The use of a growth standard that takes into account biological variation in fetal size due to fetal gender, ethnicity, maternal size and parity, or a ‘customised growth standard’, also improves the antenatal detection of SGA fetuses with adverse outcomes ( ; ). Defining a fetus as SGA using both the population-based and the customised standard was associated with a fivefold increase in the detection rate of fetuses with adverse outcomes ( ). However, in reality, the use of a customised growth standard is limited by a number of factors: (1) a large pregnancy database with sufficient numbers of normal pregnancies with different biological characteristics, mentioned above, is required to create an accurate customised growth standard; (2) fetal gender cannot always be identified accurately by ultrasonography, and, even when this is correctly identified, the parents may not want this information to be revealed; and (3) the customised growth standard may be less accurate for fetuses of mixed heritage. After birth, IUGR can be confirmed in a newborn by measuring an index of body mass or malnutrition, known as neonatal ponderal index. This is calculated as (birthweight (g)/height (cm) 3 ) × 100. A ponderal index below the 10th percentile is more closely associated with adverse perinatal outcome than birthweight alone ( ; ).
Incidence and recurrence rate
Using the 10th percentile threshold, at least 10% of singleton pregnancies will be labelled as SGA. The incidence of SGA is higher in multiple pregnancies, with approximately 20% of dichorionic and 30% of monochorionic twins being categorised as SGA ( ). The true incidence of IUGR in the general obstetric population is likely to be underestimated since it is often undetected. For example, it is found that approximately 30% of babies born before 35 weeks of gestation were below the 10th percentile for gestational age compared with 4.5% of babies born at term (37 or more weeks of gestation) ( ). Thus, the incidence of SGA is six times higher in babies born preterm than in those born at term. IUGR occurs in 30–40% of pregnancies affected by pre-eclampsia ( ). In women who had a previous IUGR pregnancy, the recurrence rate is about 20% ( ). However, this is increased to approximately 50% in women with previous early IUGR (before 34 weeks of gestation), with a highest recurrence risk in women with pre-existing renal disease and superimposed pre-eclampsia.
The aetiology of IUGR
The consequences of IUGR are shown in Table 10.2 . Normal fetal growth requires both a normal fetus and an adequate supply of substrates (i.e. oxygen and nutrients) from the maternal circulation into the fetal circulation via the placenta. The latter depends on adequate levels of substrates within the maternal circulation, adequate maternal blood flow to the placenta, and a normally functioning placenta. It was initially thought that about 80–90% of IUGR was caused by problems with the supply of substrates while an intrinsic fetal factor is the cause of a further 15–20% of cases. However, recent investigations have shown that normal endocrine signalling also plays an important role in the control and regulation of fetal and placental growth.
INTRAPARTUM | NEONATAL | LONG-TERM | ADULTHOOD |
---|---|---|---|
|
|
|
|
Factors affecting fetal growth potential (IUGR of fetal origin)
Chromosomal abnormalities, especially triploidy and trisomies 13, 18 and 21, are responsible for about 7% of fetuses with IUGR; however this figure is as high as 19% in tertiary referral centres ( ). Structural abnormalities are responsible for about 10–20% of IUGR. Genetic abnormalities and syndromes are commonly associated with IUGR. Congenital infections account for about 5% of IUGR cases ( ). IUGR is more common if fetal infection occurs early in pregnancy. Common causes of fetal infection in the developed world include cytomegalovirus and toxoplasmosis, with rubella occurring less often owing to vaccination. Common causes of fetal infections in the developing world include malaria, which accounts for up to 70% of SGA babies in endemic areas of sub-Saharan Africa ( ), and congenital human immunodeficiency virus (HIV) infection due to maternal-to-child transmission in utero.
Multiple pregnancies are associated with a high incidence of IUGR, especially in monochorionic or higher order gestations. A growth discordance of 15% or more occurs in up to 30% of twin pregnancies and this can be used as a marker for IUGR; however, both twins can be affected ( ; ). This has been attributed to ‘uterine overcrowding’. Chorionicity is the most important determinant of perinatal and long-term outcomes. There is a higher incidence of chromosomal and structural abnormalities associated with monochorionicity; problems specific to twinning are discussed in Chapter 23 ( ).
Factors leading to substrate deprivation (IUGR of maternal origin)
Fetal deprivation of nutrition or oxygen may unusually occur as a consequence of maternal undernutrition. This is associated with anorexia nervosa, body mass index less than 20 ( ; ; ), substance abuse and self-neglect, including smoking, moderate to high alcohol intake, cocaine, opiates and amphetamines ( ; ; ; ; ; ). Other maternal factors associated with IUGR include extremes of reproductive age, and poor socioeconomic status ( ; ; ; ; ). Severe maternal medical disorders may affect uterine artery blood flow; for example, vasculopathy associated with long-term diabetes ( ), chronic hypertension ( ) and renal disease ( ; ). Maternal obesity increases the risk of developing these medical disorders and their complications in pregnancy, including IUGR. Severe maternal anaemia ( ), poorly controlled asthma ( ) or cyanotic heart disease may reduce maternal oxygen supply to the placenta ( ). Certain maternal drug treatments such as beta-adrenoreceptor antagonists, phenytoin and long-term corticosteroids have been shown to have a negative effect on fetal growth ( ).
Factors affecting the transfer of substrates (IUGR of placental origin)
Poor placental function and resulting substrate deprivation are responsible for the majority of IUGR pregnancies. Placentae of IUGR pregnancies show reduced cytotrophoblast invasion and reduced surface area, increased thickness of the exchange barrier formed by the trophoblast and the fetal capillary endothelium, reduced villous density with fewer loops and coils, atheromatous change within the maternal spiral arteries, increased placental apoptosis and piling up of trophoblast due to shedding of apoptotic cells ( ; ; ; ; ; ). Normal placental development requires successful trophoblast invasion into the inner third of the myometrium and the entire length of maternal spiral arteries. The trophoblast cells destroy the muscular and elastic walls of these vessels, resulting in large flaccid uteroplacental vessels ( ). Conversion of high-resistance spiral arteries into low-resistance uteroplacental vessels is necessary to accommodate the massive increase in uterine blood flow observed in pregnancy to meet the metabolic demands of the fetoplacental unit.
To assess changes in volume blood flow within the placental circulation requires the measurement of vessel diameter, which is difficult for small vessels. However, changes in vascular resistance in the uteroplacental circulation can be assessed non-invasively using Doppler ultrasound waveform analysis of pulsatile flow in the umbilical artery (fetoplacental circulation) and uterine artery (maternoplacental circulation). The shape of the uterine or umbilical artery waveform showing high diastolic velocity with continuous diastolic flow indicates a high-flow, low-resistance uteroplacental circulation. The waveform can also be quantified by using the ratio of systolic to diastolic maximum velocity (S/D), the pulsatility index (PI; peak systolic flow minus end-diastolic flow divided by mean flow) or the resistance index (RI; peak systolic flow minus end-diastolic flow divided by peak systolic flow). The PI is the most widely studied Doppler index.
It has been shown that trophoblast invasion in early pregnancy is more extensive in pregnancies with low-resistance compared with high-resistance Doppler indices in the uterine artery ( ). In non-pregnant women the high-resistance uteroplacental vasculature leads to a rapid rise and fall in velocity during systole, an early diastolic ‘notch’ in the waveform, and low end-diastolic flow velocities ( ). Spiral artery transformation results in a significant reduction in uterine artery resistance (decreased PI and RI) between 8 and 26 weeks of gestation, and a loss of the diastolic ‘notch’ between 20 and 26 weeks of gestation ( ; ). Diastolic flow in the umbilical artery also increases as gestation increases in a normal pregnancy, producing a fall in the PI ( ; ).
Placentae from pre-eclamptic pregnancies share many histological features with the placentae of IUGR pregnancies, suggesting a common pathogenesis. In addition, both pre-eclamptic and IUGR pregnancies are associated with increased resistance or impedance to uterine artery blood flow ( ; ). Pre-eclampsia is a pregnancy-specific systemic endothelial disorder which affects about 2% of pregnancies. Although the development of maternal hypertension in the second half of the pregnancy with the presence of significant proteinuria is diagnostic of pre-eclampsia, the aetiology is heterogeneous with variable clinical presentation ( ). Early-onset pre-eclampsia (before 34 weeks of gestation) is associated with a higher incidence of poor placental function, a more severe IUGR with a higher risk of maternal mortality and morbidity ( ). Conversely, late-onset pre-eclampsia is more prevalent but with minimal placental dysfunction.
A single umbilical artery (SUA) is associated with a 20-fold increased risk of cardiac anomalies and a threefold increased risk of renal anomalies. However, even in fetuses with isolated SUA there is a twofold increased risk of IUGR ( ). Both acquired (e.g. antiphospholipid syndrome) and inherited thrombophilias predispose to IUGR, as they may increase the risk of thrombotic lesions within the placenta ( ). It has been suggested that there is an increased frequency of the prothrombin mutation and the methyltetrahydrofolate reductase mutation in mothers of IUGR babies ( ). Tobacco smoking increases the risk of SGA and acts in a dose- and gestation-dependent manner to reduce birthweight, probably via adverse effects on placental blood flow ( ). A recent SCOPE study showed that the SGA rate in women who stopped smoking by 15 weeks of gestation was equivalent to that of non-smokers ( ).
Endocrine factors
Important regulators of fetal growth, differentiation and function are the insulin-like growth factors (IGF), including IGF-1 and 11, IGF-binding proteins (IGFBP) 1–6, IGF receptors 1 and 2 and IGFBP-specific proteases. IGF plays an important role in trophoblast invasion ( ), and abnormalities in IGF signalling have been associated with IUGR ( ). A reduced fetal serum IGF-1 is associated with IUGR, while overexpression of IGF-11 is associated with fetal overgrowth, as observed in Beckwith–Wiedemann syndrome. In addition, low plasma IGFBP-1 in early pregnancy is associated with an increased risk of pre-eclampsia. Pregnancy-associated plasma protein A (PAPP-A) is a trophoblast-derived protease that cleaves IGFBP-4, thus increasing the bioavailability of IGF ( ). A low maternal serum PAPP-A in the first trimester of pregnancy is also associated with an increased risk for subsequent development of pre-eclampsia ( ), and PAPP-A levels are significantly lower in women developing early-onset than late pre-eclampsia ( ).
In addition, decreased levels of angiogenic proteins, such as vascular endothelial growth factor (VEGF) and placental growth factor (PlGF), and increased levels of angiogenic inhibitor, such as fms-like tyrosin kinase 1 (sFlt-1), are associated with reduced trophoblast invasion ( ; ; ). There is an inverse correlation between maternal PlGF and uterine artery PI ( ). Elevated maternal sFlt-1 is associated with increased uterine artery PI, pre-eclampsia and IUGR; and higher sFlt-1 levels were observed in women with adverse pregnancy outcomes resulting in delivery before 34 weeks of gestation. An inverse correlation has been shown between birthweight and maternal corticotrophin-releasing hormone (CRH) levels at 33 weeks of gestation. An elevated CRH level is associated with a 3.6-fold increased risk of IUGR ( ). In addition, there is a relationship between birthweight and maternal neurokinin B and nitric oxide metabolite levels ( ).
Physiological sequelae of IUGR
The majority of fetuses with IUGR will be substrate-deprived due to the abnormal transfer of oxygen and nutrients (glucose, amino acids, lipids) across the placenta. Insights into the fetal responses to such deprivation are important as they: (1) underpin the rationale for the techniques used to monitor fetal wellbeing; (2) often lead to improved short-term survival; and (3) can also cause some of the short- and long-term complications associated with IUGR.
Fetal heart rate
Acute fetal hypoxaemia is associated with well-described changes in fetal heart rate (FHR); these underpin the use of electronic fetal monitoring on the labour ward ( Ch. 12 ). However, experiments in fetal sheep where the fetoplacental circulation was embolised every day for 10 days leading to chronic hypoxaemia and a 50% reduction in umbilical flow did not change baseline FHR or lead to decelerations or changes in short-term variability ( ). These suggest that changes in FHR patterns are likely to be a late occurrence in the sequence of haemodynamic change seen in the IUGR fetus ( Fig. 10.1 ), and agree with studies from human fetuses with absent end-diastolic flow in the umbilical artery. Here, abnormal FHR patterns were only observed when the middle cerebral artery (MCA) began to lose its compensatory maximal dilation, i.e. the fetus was entering a preterminal phase ( ).

Regional blood flow
In contrast, changes in regional blood flow with maintained, chronic fetal hypoxaemia are well described in a number of animal species. In fetal sheep blood flow to the brain, heart and adrenal glands increases rapidly with the onset of acute hypoxaemia and remains high if hypoxaemia is maintained; this effect is observed from mid- to late gestation ( ; ; ). The effect of the increased blood flow is to maintain substrate delivery that is critically important for the maintenance of growth and optimal function of these key organs. Doppler studies in fetuses with IUGR suggest that vascular resistance is increased in a variety of vessels, including the splanchnic, renal and pulmonary circulations, suggesting that the reductions in blood flow to the gut, kidneys and lungs that are observed during acute hypoxia in fetal sheep are maintained during sustained hypoxaemia ( ; ; ). Detection of substrate deficiency occurs both locally and at central chemoreceptors and is sensitive and rapid; detection of cerebral vasodilation is therefore an early finding in IUGR.
Abnormal cerebral blood flow is an extremely sensitive test for the prediction of IUGR, but it has poor specificity in the detection of adverse perinatal outcome ( ). Whilst redistribution of cardiac output optimises short-term survival with chronic hypoxaemia, there is some evidence that it is responsible for some of the immediate postnatal complications observed in growth-restricted neonates. ) described a reduction in flow velocity, which persisted until the end of the first week of life, in both superior mesenteric artery and the coeliac axis in growth-restricted compared with normally grown neonates of similar gestational age. The increased incidence of necrotising enterocolitis (NEC) observed in IUGR fetuses may be related to ischaemic damage occurring either in utero or following delivery ( ). Oligohydramnios, a common finding in IUGR pregnancies, is probably related to reduced renal perfusion and glomerular filtration. Again, chronic reduction in lung growth might underlie the excess incidence of chronic lung disease observed in infants with severe IUGR ( ). Because of the correlation between the growth of an organ and its blood supply, organ size appears to be a sensitive indicator of redistribution of cardiac output; indeed, relative shortening of the femurs in the early second trimester has been reported as an early sign of incipient growth restriction, often preceding other evidence by several weeks ( ).
In addition to the redistribution of cardiac output, chronic hypoxia can also influence the venous pattern of blood flow and return of blood to the heart. Approximately 25–50% of blood returning from the placenta via the umbilical vein is shunted via the ductus venosus (DV), which connects with the inferior cava near its entrance to the heart ( ; ). This well-oxygenated blood is preferentially streamed through the foramen ovale into the left atrium and from there towards the cardiac and cerebral circulations ( ). In both human and other mammalian fetuses the amount of blood flowing through the DV increases during hypoxia ( ; ). A direct consequence of increased DV shunting is that the proportion of umbilical vein flow that passes via the hepatic circulation is reduced; a fall in hepatic perfusion and decreased substrate supply can impair liver function and glycogen synthesis and storage (reflected in a decrease in growth velocity in the abdominal circumference). Not surprisingly, flow velocity is maintained in the DV even with severe IUGR ( ), because the DV and left atrium are linked by a direct column of blood. Increases in left atrial filling pressure, due mainly to impaired ventricular contractility, but also increased afterload, will lead to reduced forward flow in the DV during atrial systole. Decreased or absent flow during atrial systole is a late finding in the haemodynamic response to hypoxia and, because it indicates cardiac compromise, correlates strongly with poor perinatal outcome ( ).
The consequences of IUGR
The short-term consequences of IUGR include the increased risk of perinatal mortality, and perinatal morbidity, including birth asphyxia, meconium aspiration and the consequences of preterm birth. About half of IUGR survivors will have significant short- or long-term morbidity ( ). The extent and severity of morbidity depend on the cause and severity of IUGR and the neonatal consequence of fetal adaptation to chronic hypoxia, complications during labour and delivery, the gestational age at birth and immaturity of function, place of birth and the presence of chromosomal or structural anomalies. The commonest causes of death are prolonged hypoxia, birth asphyxia, prematurity and congenital anomalies. It is anticipated that a preterm IUGR baby will have more complications than a term IUGR baby and would require specialist neonatal care from birth. An IUGR fetus with chronic hypoxia is at increased risk of birth asphyxia at all gestational ages ( ). In the immediate neonatal period, birth asphyxia and metabolic acidosis may lead to multiorgan dysfunction, including hypoxic–ischaemic encephalopathy, seizures, intraventricular haemorrhage, respiratory distress due to surfactant deficiency, meconium aspiration or pulmonary haemorrhage, persistent pulmonary hypertension, hypotension due to myocardial dysfunction, transient tricuspid valve insufficiency, renal failure due to acute cortical necrosis, coagulopathy and elevated aminotransferase due to liver hypoxia, hypocalcaemia, and adrenal insufficiency due to haemorrhage ( ; ; ).
The fetal redistribution of circulation to vital organs in response to hypoxaemia and substrate deprivation may lead to problems with neonatal adaptation to extrauterine life. For example, there is an increased risk of NEC due to bowel ischaemia. The risk of NEC is higher in IUGR babies with absent or reversed end-diastolic flow in the umbilical artery before birth ( ). Since the reduced splanchnic blood flow may persist in the first week of life, oral feeding should be introduced cautiously during this time. Other complications include hypoglycaemia due to limited glycogen stores; polycythaemia due to the increased synthesis of erythropoietin in chronic hypoxia and consequently hyperviscosity, renal vein thrombosis and hyperbilirubinaemia; respiratory distress syndrome; problems with thermoregulation due to limited adipose tissue stores and a relatively large body surface area; retinopathy of prematurity; and hyperglycaemia due to low insulin secretion in very preterm neonates.
Beyond the neonatal period, there is an increased risk of developing neurological abnormalities including cognitive delay and cerebral palsy, especially in babies born preterm ( ; ). Magnetic resonance imaging of the brain of IUGR infants has shown reduced volume of cerebral cortical grey matter ( ). About 90% of babies with SGA will show catch-up growth by 6 months of age, but 10% will remain below the third percentile for height at 2–3 years of age and may benefit from growth hormone therapy ( ). However, animal models have shown that IUGR is associated with reduced leptin levels, a satiety factor which controls appetite and food intake ( ). Although the level of leptin increased in the adult IUGR offspring, there is a decreased anorexic response to leptin, and this is especially demonstrated in IUGR offspring with rapid catch-up growth ( ). It has been suggested that altered control of appetite in the adult IUGR offspring leads to adult obesity. Besides obesity, there is a growing body of evidence to support the concept that there are fetal origins for adult diseases such as adult hypertension, high cholesterol levels, type 2 diabetes mellitus, abnormal coagulation factors, coronary heart disease, stroke, bronchitis, premature menopause in women and Down syndrome pregnancy ( ; ; ; ; ; ; ; ). One hypothesis is that the IUGR fetus is programmed to exhibit the ‘thrifty phenotype’ where the metabolism is permanently reset to adapt to poor nutritional conditions, such that the extrauterine exposure to western diet predisposes the offspring to obesity and metabolic syndrome as an adult.
Primary prevention of IUGR
Prepregnancy counselling and health education, as well as optimising any pre-existing medical conditions before pregnancy, are important. Prepregnancy folic acid supplementation to prevent neural tube defects should be advocated, as well as the importance of good nutrition, the avoidance of harmful substances such as smoking, alcohol, illicit drug abuse, and reduction of caffeine intake ( ; ). In addition, women should be informed that smoking cessation before the third trimester will reduce the risk of IUGR ( ). In women who have a high risk of pre-eclampsia, low-dose aspirin reduces the risk of developing pre-eclampsia by 17% and reduces the risk of SGA by 10% ( ).
Since maternal obesity increases the risk of hypertensive disorders in pregnancy, which in turn increases the risk of IUGR ( ), it may be beneficial to encourage weight loss before pregnancy, or to limit weight gain during pregnancy in obese women ( ). Women with eating disorders should also be counselled regarding the increased risk of IUGR pregnancies. It has been shown that a pregnant woman with a body mass index of less than 20 has a higher risk of an SGA fetus when the total gestational weight gain is less than 8 kg, in comparison with a weight gain of more than 16 kg ( ). However, the provision of social support for women of low socioeconomic status has not been shown to reduce the incidence of SGA, as poor diet is the probable mechanism by which socioeconomic status influences growth ( ).
In order to prevent congenital infection and IUGR, all women should be vaccinated against rubella before pregnancy. In malaria-endemic areas, the routine use of antimalarial drugs and insecticide-treated nets for women in their first two pregnancies has been shown to reduce the incidence of low-birthweight babies ( ; ). Antiretroviral treatment in women with HIV infection can reduce in utero maternal-to-child-transmission of HIV from 25% to 2%, and thus prevent IUGR due to congenital HIV infection ( ).
Antenatal screening and diagnosis
The antenatal diagnosis of IUGR relies on a number of observations, including: (1) current fetal size, taking into account the fetal growth potential such as ethnicity, fetal gender, maternal height and parity; (2) serial ultrasound measurements of fetal size to demonstrate reduced growth velocity, where the EFW decreases in its percentile value for gestational age; (3) indicator of fetal compromise, where fetal Doppler demonstrates redistribution of fetal circulation in response to hypoxaemia, particularly a decreased impedance to MCA blood flow; and (4) indicator of poor uteroplacental function, where uterine artery Doppler (UAD) indicates a persistent high-resistance circulation at 24 or more weeks of gestation and/or umbilical artery Doppler waveform shows increased impedance to blood flow.
However, in an unselected obstetric population, the evidence does not support the use of routine fetal biometry after 24 weeks of gestation, routine umbilical artery Doppler ultrasound, routine UAD ultrasound or routine antenatal electronic fetal heart monitoring for the prediction of IUGR ( ; ; ). Each screening tool will have a higher positive predictive value if there is an increased risk of IUGR based on maternal history and clinical examination. Therefore, the principle of antenatal care is the identification of pregnancies at high risk of IUGR through history, examination, and biochemical and ultrasound screening.
Ultrasound assessment of gestational age and fetal size
A reliable estimate of gestational age is a prerequisite for the early detection of IUGR, interpretation of serum screening tests and planning for elective delivery. Gestational age is the strongest determinant of fetal size and perinatal outcome. The last menstrual period (LMP) should not be used alone to date a pregnancy. Even if LMP is recalled accurately, it is not a precise indicator of the conception date ( ; ; ). This is because the timing of ovulation varies cyclically, even in women with regular menstrual cycles. In addition, errors in recall are common, with a tendency to digit preference when recalling dates, and falsely identifying non-menstrual bleeding as a menstrual period ( ; ).
Dating methods using ultrasonographic assessment of fetal size before 20 weeks of gestation have been shown to reduce the percentage of pregnancies classified as ‘postterm’ by up to 74% when compared with dating based on LMP only. In the first trimester of pregnancy (up to 14 weeks), ultrasound measurement of the fetal crown–rump length (CRL) provides a relatively accurate estimate of gestational age, with a standard deviation of approximately 7 days ( ; ). The CRL measurement becomes less reliable beyond 13 weeks because the fetus becomes increasingly flexed with advancing gestation.
In the second trimester, the most accurate estimate of gestational age is the head circumference (HC), while the most sensitive indicator of fetal size is the abdominal circumference (AC) ( ; ). The accuracy of dating using HC is improved with the addition of AC and femur length (FL) measurements, such that fetal biometry in the second trimester also provides an accurate estimate of gestational age within 7 days ( ). In addition, the calculation of EFW is more accurate using all three biometric measures than any one measurement alone ( ). Using the Hadlock formula, which combines HC, AC and FL, the EFW is within 15% of the actual fetal weight when the fetus weighs between 1500 and 3999 g ( ). Errors as high as 25% between the predicted and actual fetal weight occur when a fetus weighs below 1500 g, with the tendency to overestimate fetal weight; or when a fetus weighs above 4000 g, with the tendency to underestimate ( ).
Most obstetric units use a combination of the LMP and dating based on ultrasound fetal biometry, only favouring the ultrasound dates if the discrepancy is greater than 7, 10 or 14 days depending on gestational age at first ultrasound scan. However, it is recommended that the use of CRL measurement between 10 and 13 weeks alone should be used to determine gestational age ( ). For women who booked late for antenatal care, the use of ultrasound for dating is less reliable beyond 24 weeks, owing to the increased variation in fetal growth rate and imprecise measurements as a result of descent of the fetal head into the pelvis, shadowing from fetal bony parts and fetal crowding ( ). The transcerebellar diameter is a useful adjunct in determining gestational age in the second and third trimester because its measurement is independent of fetal head shape and there is a tendency for cerebellar growth to be preserved in IUGR ( ). However, if pregnancy dating is performed beyond 24 weeks, serial ultrasound scans should also be performed to confirm a normal growth velocity, instead of a one-off measurement of fetal size.
Identification of high-risk pregnancy
Once the best estimate of gestational age is determined, the principle of antenatal care is the recognition of pregnancies at high risk of IUGR through history, examination, and biochemical and ultrasound screening.
Maternal history
In addition to the risk factors mentioned in the aetiology section, women with recurrent bleeding should be regarded as high-risk. A previous IUGR pregnancy increases the risk of recurrent IUGR in subsequent pregnancies ( ).
Clinical examination
The traditional screening methods for fetal growth using abdominal palpation or measurement of symphysis–fundal height (SFH) have poor detection rates for IUGR. In a low-risk population, abdominal palpation has a sensitivity of only 21% and a specificity of 96% for detecting SGA fetuses ( ). After 20 weeks of gestation, the SFH in centimetres from the upper edge of the pubic symphysis to the top of the uterine fundus approximates to the number of weeks of gestation. Differences in maternal build and subjectivity of this test have led to a considerable variation in the results of studies using SFH, with the largest study showing a sensitivity of only 27%, and a specificity of 88% for detecting SGA fetuses ( ). The selection of women with SFH that is below expectation for ultrasound fetal biometry reduces the false-positive rate for detecting SGA when compared with a policy of scanning all women in the third trimester, but the sensitivity is not improved with this method ( ).
Maternal serum screening
The combined test is offered to all women in the first trimester for Down syndrome screening. This involves fetal nuchal translucency scan and maternal serum PAPP-A and human chorionic gonadotrophin (hCG) levels. Women who missed the first trimester screening have the option of serum screening in the second trimester, including alpha-fetoprotein, oestriol, hCG and inhibin A. Pregnancies identified as high-risk for Down syndrome due to significantly reduced PAPP-A levels should be monitored for fetal growth, even when invasive testing showed normal fetal karyotype.
Other methods of screening which have not been adopted in clinical practice include using angiogenic markers such as PlGF or sFlt-1. These have sensitivities of 55–60% and specificities of 60–70% of predicting pre-eclampsia or IUGR ( ). In addition, sFlt-1 had a higher sensitivity of 80% and a specificity of 94% for predicting adverse outcome resulting in delivery before 34 weeks of gestation.
Ultrasound screening
Ultrasound screening for structural malformation is offered to all women between 20 and 22 weeks of gestation. In the absence of structural malformations, the markers associated with an increased risk of IUGR include bright or echogenic fetal bowel, single umbilical artery, isolated short femur (where the femur is below the fifth percentile for gestational age but the abdominal circumference measurements and the EFW are within the normal range) ( ). Beyond 24 weeks of gestation, there is no evidence that routine ultrasound scan to assess growth in a low-risk population improves perinatal outcome, and it has been suggested that this may increase the rate of caesarean section.
Screening for adverse outcome using UAD has produced variable results depending on gestational age, presence of risk factors and severity of outcome. In a study of high-risk multiparous women, abnormal UAD had a sensitivity of 80% and a positive predictive value of 70% for identifying women who subsequently developed pre-eclampsia, SGA below the fifth percentile, placental abruption, preterm birth and perinatal mortality ( ). In a recent systematic review and meta-analysis of 74 studies totalling 79 547 patients with pre-eclampsia and 61 studies of 41 141 IUGR pregnancies, it was reported that an abnormal UAD waveform had a higher predictive value for both pre-eclampsia and IUGR when performed in the second trimester (beyond 16 weeks of gestation) than in the first trimester ( ). Screening with UAD was also more predictive of pre-eclampsia than IUGR, and increased PI with diastolic notching was the best predictor of pre-eclampsia (positive likelihood ratio of 21 for high-risk patients and 7.5 for low-risk patients). Increased PI and diastolic notching were the best predictors of IUGR in low-risk patients (positive likelihood ratio 9.1). For severe IUGR, the predictive value was higher (positive likelihood ratio 14.6).
Identifying IUGR using ultrasound fetal biometry
Ultrasound of fetal size alone has a low predictive ability and high false-positive rate for detecting IUGR. The fetal AC indicates the nutritional state of the fetus, as this is a measure of subcutaneous, intra-abdominal and extraperitoneal fat and liver size. An AC below the 10th centile was found to predict SGA with an odds ratio of 13.5 in a low-risk population and 18.4 in a high-risk population ( ). A more recent study has shown that the accuracy of predicting SGA was similar when either the AC or the EFW alone was below the 10th centile. In addition, the likelihood of SGA was found to be greater when both the AC and the EFW were below the 10th centile ( ). Although EFW calculations do not aid in the diagnosis of IUGR, they are helpful in planning delivery and neonatal care. Serial measurements of fetal biometry every 2–4 weeks should be used to identify any deviation of growth across the centiles.
Ultrasound assessment of liquor volume
Liquor volume is assessed by measuring the maximum pool depth, or, more accurately, by adding the pool depth in each quadrant of the uterus to produce the amniotic fluid index. Although oligohydramnios is associated with more severe IUGR, the predictive value of these measurements is limited, so they are used as an adjunct to other elements of the fetal assessment.
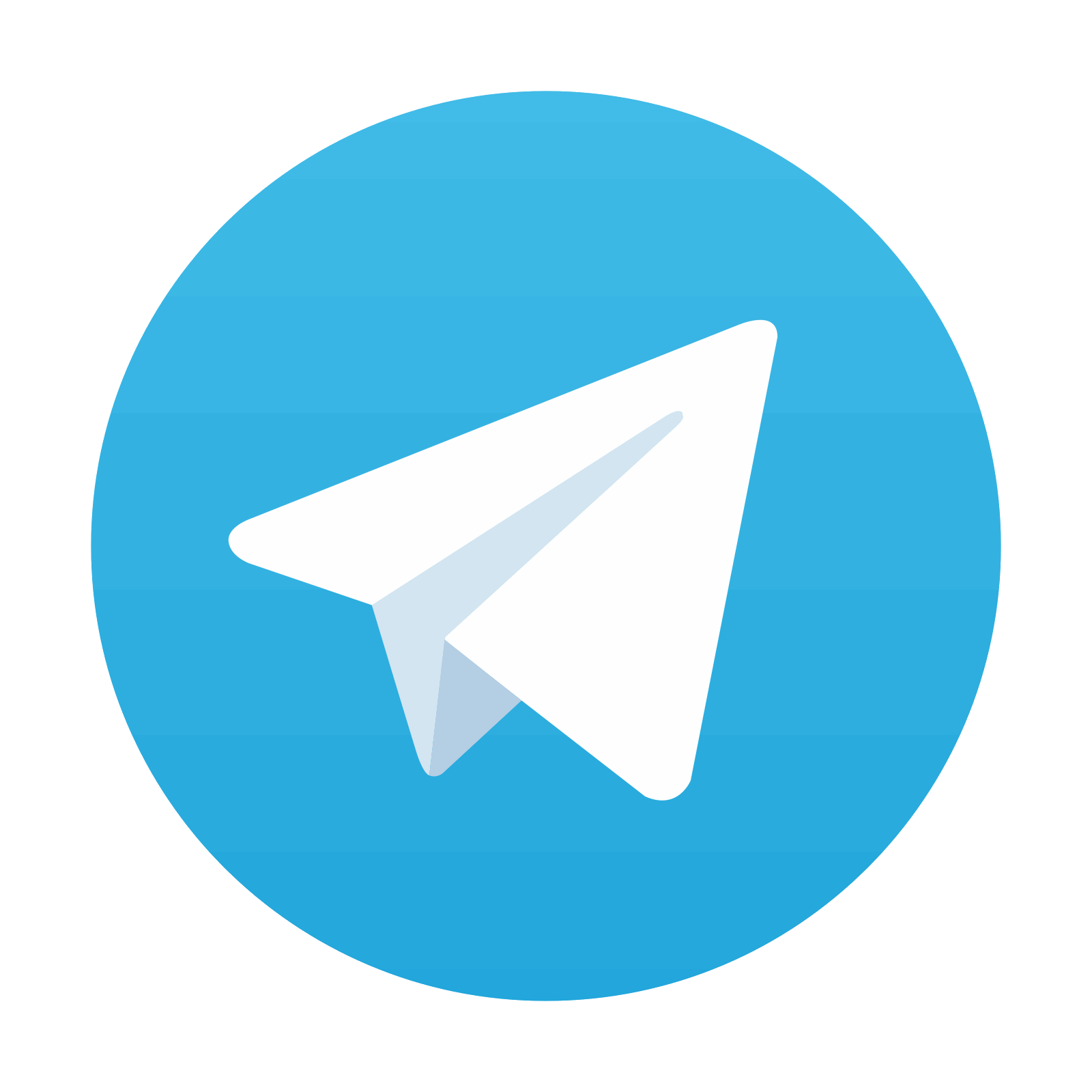
Stay updated, free articles. Join our Telegram channel

Full access? Get Clinical Tree
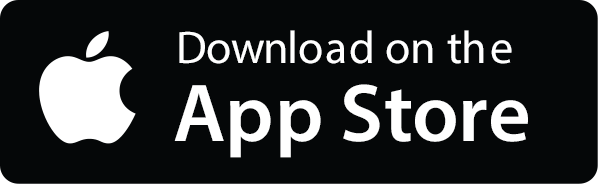
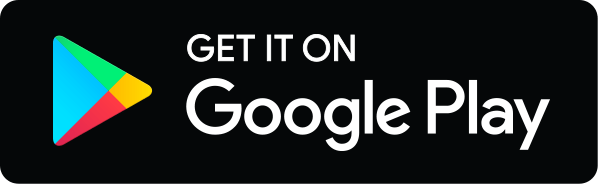