Background
The use of wide pore lightweight polypropylene mesh to improve anatomical outcomes in the surgical repair of prolapse has been hampered by mesh complications. One of the prototype prolapse meshes has been found to negatively impact the vagina by inducing a decrease in smooth muscle volume and contractility and the degradation of key structural proteins (collagen and elastin), resulting in vaginal degeneration. Recently, bioscaffolds derived from extracellular matrix have been used to mediate tissue regeneration and have been widely adopted in tissue engineering applications.
Objective
Here we aimed to: (1) define whether augmentation of a polypropylene prolapse mesh with an extracellular matrix regenerative graft in a primate sacrocolpopexy model could mitigate the degenerative changes; and (2) determine the impact of the extracellular matrix graft on vagina when implanted alone.
Study Design
A polypropylene-extracellular matrix composite graft (n = 9) and a 6-layered extracellular matrix graft alone (n = 8) were implanted in 17 middle-aged parous rhesus macaques via sacrocolpopexy and compared to historical data obtained from sham (n = 12) and the polypropylene mesh (n = 12) implanted by the same method. Vaginal function was measured in passive (ball-burst test) and active (smooth muscle contractility) mechanical tests. Vaginal histomorphologic/biochemical assessments included hematoxylin-eosin and trichrome staining, immunofluorescent labeling of α-smooth muscle actin and apoptotic cells, measurement of total collagen, collagen subtypes (ratio III/I), mature elastin, and sulfated glycosaminoglycans. Statistical analyses included 1-way analysis of variance, Kruskal-Wallis, and appropriate post-hoc tests.
Results
The host inflammatory response in the composite mesh-implanted vagina was reduced compared to that following implantation with the polypropylene mesh alone. The increase in apoptotic cells observed with the polypropylene mesh was blunted in the composite (overall P < .001). Passive mechanical testing showed inferior parameters for both polypropylene mesh alone and the composite compared to sham whereas the contractility and thickness of smooth muscle layer in the composite were improved with a value similar to sham, which was distinct from the decreases observed with polypropylene mesh alone. Biochemically, the composite had similar mature elastin content, sulfated glycosaminoglycan content, and collagen subtype III/I ratio but lower total collagen content when compared to sham ( P = .011). Multilayered extracellular matrix graft alone showed overall comparable values to sham in aspects of the biomechanical, histomorphologic, or biochemical endpoints of the vagina. The increased collagen subtype ratio III/I with the extracellular matrix graft alone ( P = .033 compared to sham) is consistent with an ongoing active remodeling response.
Conclusion
Mesh augmentation with a regenerative extracellular matrix graft attenuated the negative impact of polypropylene mesh on the vagina. Application of the extracellular matrix graft alone had no measurable negative effects suggesting that the benefits of this extracellular matrix graft occur when used without a permanent material. Future studies will focus on understanding mechanisms.
Introduction
Lightweight polypropylene mesh used in the surgical repair of pelvic organ prolapse has been associated with significant mesh-related complications, most commonly mesh exposure and pain. Gynemesh PS (Ethicon, Sommersville, NJ), one of the most widely implanted polypropylene meshes, has been shown to induce a robust foreign-body response and degenerative changes in the vagina when implanted via sacrocolpopexy including a decrease in smooth muscle contractility and volume, a loss of mechanical integrity, a decrease in matrix structural proteins (collagen and elastin) accompanied by an increase in glycosaminoglycan (GAG) content, and an increase in the amount and activity of matrix-degrading proteases.
In theory, these negative effects could be overcome with the use of regenerative biomaterials either in combination with Gynemesh PS as a composite graft or alone with the elimination of permanent materials. However, given that the sacrocolpopexy is not an anatomical surgery and regenerative matrices are designed to restore or repair injured tissues in a site-specific manner, it is not clear whether the use of a regenerative matrix in sacrocolpopexy surgery would be successful.
Products derived from extracellular matrices (ECM) have been widely adopted in tissue-engineering applications and are considered a novel tool to facilitate tissue regeneration. It has been shown that these grafts, when placed in the appropriate micro- and loading environments, can promote site-specific functional tissue remodeling by limiting destructive inflammatory responses, recruiting requisite cell populations including progenitor cells, and supporting cell attachment, proliferation, and matrix production.
We chose to use MatriStem Surgical Matrix RS (MatriStem RS, ACell Inc, Columbia, MD), a noncross-linked degradable acellular porcine urinary bladder matrix, because it facilitates reconstructive remodeling of smooth muscle and connective tissue as shown in its application in cardiac defect repair, esophagus reinforcement, and chronic wound repairs. It is important to distinguish regenerative matrices such as MatriStem (noncross-linked) from biologic materials that have been chemically cross-linked (eg, Pelvicol, Bard, Murray Hill, NJ) to provide mechanical support similar to polypropylene mesh. Permanent cross-links can elicit more robust proinflammatory foreign-body responses resulting in encapsulation and fibrosis. Consequently, cross-linked matrices have been associated with comparable or increased complication rates relative to that for the polypropylene mesh in prolapse surgeries.
In this study, we used a 2-ply MatriStem in our composite graft to counteract the degenerative changes induced by Gynemesh PS (referred to as Gynemesh going forward) with minimal impact on its mechanical properties. To evaluate the potential of using MatriStem alone in prolapse repair, we chose a 6-layered product (MatriStem pelvic floor matrix) with mechanical properties in the range of those of polypropylene mesh and slowed degradation rate for replacement by host tissue. We hypothesized that an ECM graft, via its innate antiinflammatory and regenerative properties, would limit the negative impact of Gynemesh, and/or act as a physical barrier between the native tissue and the mesh. We further hypothesized that an ECM graft alone would maintain/restore the structural and functional properties of the vagina and support a novel tissue bridge between the vagina and the sacrum. The Gynemesh-MatriStem composite and MatriStem alone grafts were implanted in a rhesus macaque model via sacrocolpopexy. The grafted vaginal tissues were evaluated 3 months after surgery.
Materials and Methods
Animals
Middle-aged rhesus macaques were maintained and treated according to experimental protocols approved by the Institutional Animal Care Use Committee of the University of Pittsburgh (no. 13081928). Age, weight, parity, and Pelvic Organ Prolapse Quantification (POP-Q) stage were collected prior to and after surgery. The POP-Q stage was evaluated according to a modified POP-Q examination.
Surgical procedures
Sterile samples of Gynemesh, and 2-ply and 6-ply MatriStem Surgical Matrix were obtained and trimmed into straps appropriate for sacrocolpopexy (3-cm wide × 10cm long). Seventeen middle-aged parous rhesus macaques were implanted with a composite mesh comprised of Gynemesh plus 2-ply MatriStem (n = 9) or 6-ply MatriStem alone (n = 8) via sacrocolpopexy after hysterectomy ( Figure 1 ). For the composite, the precut MatriStem, trimmed to the size of Gynemesh, was sutured to the mesh with absorbable 3-0 Vicryl (Ethicon) before mesh insertion. At implantation, the composite mesh was placed with the MatriStem side facing the vaginal lumen, forming a layer between the Gynemesh and the vagina. The implantation and postoperative care were performed as previously described. Twelve weeks after surgery, the graft-vagina complex (GVC) was harvested for functional, morphological, and biochemical analyses. Sham (animals underwent the identical surgery without mesh insertion, n = 12) and Gynemesh implanted (animals underwent the identical surgery with insertion of Gynemesh, n = 12) tissues, properly stored from a previous study, were used for measurement of elastin content and collagen type III/I ratio. For histomorphometrics, biomechanical testing, collagen, and GAG content, previously published data on sham and Gynemesh-implanted vagina were used for comparison.
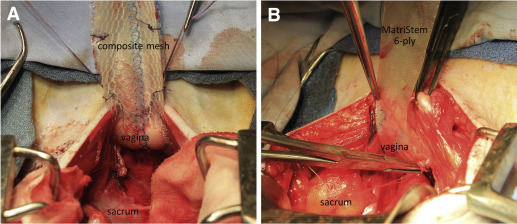
Ball-burst test for biomechanical properties
The passive mechanical properties of GVC were determined by the ball-burst test. All GVC samples used for ball-burst testing were obtained from the posterior vagina. Fifteen samples from composite mesh (n = 7) and MatriStem alone (n = 8) groups were tested whereas 2 GVC samples from the composite mesh group were too small to test (vagina in these animals was small). Structural properties including stiffness (N/mm), ultimate load (N), ultimate elongation (mm), and energy absorbed (N/mm) were determined. The contribution of soft tissues associated with the GVC was determined for ultimate load (load tissue ) and stiffness (k tissue ) by subtracting the average corresponding values of Gynemesh obtained ex vivo. For samples that were grafted with MatriStem alone, the tissue contribution was equal to those values obtained for the entire complex. Since noncross-linked ECM grafts degrade rapidly in vivo (10-ply 14 C-labeled ECM was completely degraded by 3 months), we assumed that MatriStem grafts were completely degraded during the implantation period (3 months) and replaced by host connective tissue, and thus, the ECM graft did not contribute to the mechanical integrity at the time of tissue procurement.
Functional test for smooth muscle contractility
The contractile response to a single dose of potassium chloride (KCl, 120 mmol/L) was tested within 30 minutes of tissue harvest as described previously. To normalize for differences in tissue quantity, the data were expressed as force per tissue volume of each strip (mN/mm 3 ).
Immunofluorescent labeling of α-smooth muscle actin and in situ terminal deoxynucleotidyl transferase dUTP nick end labeling (TUNEL) labeling of cell apoptosis
GVCs were cryosectioned (7 μm) perpendicular to the longitudinal axis of the vagina. Hematoxylin-eosin and Masson trichrome staining were performed following manufacturer’s protocols (Sigma-Aldrich, St Louis, MO). For analysis, specimens were required to contain all layers of vagina, and those obliquely sectioned with subepithelium >1039 μm (mean of sham values that were sectioned perpendicularly plus 2SD) were excluded. One sample from composite mesh was excluded for not containing all layers of vagina.
Immunofluorescent labeling of α-smooth muscle actin and in situ TUNEL labeling of apoptotic cells were used to measure thickness of the subepithelial and smooth muscle layers, and the percentage of apoptotic cells in each vaginal layer as previously described.
Biochemical assays
Total collagen content and sulfated GAG content were measured as previously described, and expressed as percentage of tissue dry weight.
Mature elastin content was measured as the total content of desmosine and isodesmosine by ultra high-performance liquid chromography (UPLC). Briefly, hydrolyzed tissue was applied to Oasis mixed-mode cation-exchange (MCX) solid-phase extraction (SPE) columns (Waters, Milford, MA), and eluted with ammonium hydroxide/water/methanol (10/40/50). Stock solutions of desmosine, isodesmosine, and S-[beta-(4-pyridyl)ethyl]L-cysteine (S-LC, internal standard) (Santa Cruz Biotechnology Inc, Dallas, TX) were diluted to series concentration to create standard curves. S-LC (2 μmol) was added to each sample and standards as an internal control. A total of 3 μL of the prepared samples and standards were injected onto a prepared Waters Acquity UPLC ethylene bridged hybrid (BEH) C18 column and monitored by measuring absorbance at 270-nm wavelength. The elution time of isodesmosine was 1.4 minutes, desmosine was 1.9 minutes, and S-LC was 2.4 minutes. The amount of mature elastin in each sample was represented by the sum of desmosine and isodesmosine normalized by that for bovine elastin (Sigma-Aldrich).
Ratios of collagen subtype III/I in the tissue of GVC were determined as previously described and calculated as α1(III) ×2/α1(I) ×3 normalized by the ratio of purified collagen I/III.
Statistical analysis
Sample size was determined using previously published mechanical data (ultimate load and stiffness) for sham and Gynemesh, in which at least 8 animals were needed in each group to achieve significance with α error of 0.05 and power of 0.80. Data were tested for distribution before statistical analysis. For normally distributed data, a 1-way analysis of variance was used followed by the appropriate post-hoc tests including Dunnett for comparison to sham and pairwise test using Bonferroni multiple comparisons procedure between all groups. For nonparametric data, Kruskal-Wallis was used to compare overall significance and Mann-Whitney to compare between groups.
Materials and Methods
Animals
Middle-aged rhesus macaques were maintained and treated according to experimental protocols approved by the Institutional Animal Care Use Committee of the University of Pittsburgh (no. 13081928). Age, weight, parity, and Pelvic Organ Prolapse Quantification (POP-Q) stage were collected prior to and after surgery. The POP-Q stage was evaluated according to a modified POP-Q examination.
Surgical procedures
Sterile samples of Gynemesh, and 2-ply and 6-ply MatriStem Surgical Matrix were obtained and trimmed into straps appropriate for sacrocolpopexy (3-cm wide × 10cm long). Seventeen middle-aged parous rhesus macaques were implanted with a composite mesh comprised of Gynemesh plus 2-ply MatriStem (n = 9) or 6-ply MatriStem alone (n = 8) via sacrocolpopexy after hysterectomy ( Figure 1 ). For the composite, the precut MatriStem, trimmed to the size of Gynemesh, was sutured to the mesh with absorbable 3-0 Vicryl (Ethicon) before mesh insertion. At implantation, the composite mesh was placed with the MatriStem side facing the vaginal lumen, forming a layer between the Gynemesh and the vagina. The implantation and postoperative care were performed as previously described. Twelve weeks after surgery, the graft-vagina complex (GVC) was harvested for functional, morphological, and biochemical analyses. Sham (animals underwent the identical surgery without mesh insertion, n = 12) and Gynemesh implanted (animals underwent the identical surgery with insertion of Gynemesh, n = 12) tissues, properly stored from a previous study, were used for measurement of elastin content and collagen type III/I ratio. For histomorphometrics, biomechanical testing, collagen, and GAG content, previously published data on sham and Gynemesh-implanted vagina were used for comparison.
Ball-burst test for biomechanical properties
The passive mechanical properties of GVC were determined by the ball-burst test. All GVC samples used for ball-burst testing were obtained from the posterior vagina. Fifteen samples from composite mesh (n = 7) and MatriStem alone (n = 8) groups were tested whereas 2 GVC samples from the composite mesh group were too small to test (vagina in these animals was small). Structural properties including stiffness (N/mm), ultimate load (N), ultimate elongation (mm), and energy absorbed (N/mm) were determined. The contribution of soft tissues associated with the GVC was determined for ultimate load (load tissue ) and stiffness (k tissue ) by subtracting the average corresponding values of Gynemesh obtained ex vivo. For samples that were grafted with MatriStem alone, the tissue contribution was equal to those values obtained for the entire complex. Since noncross-linked ECM grafts degrade rapidly in vivo (10-ply 14 C-labeled ECM was completely degraded by 3 months), we assumed that MatriStem grafts were completely degraded during the implantation period (3 months) and replaced by host connective tissue, and thus, the ECM graft did not contribute to the mechanical integrity at the time of tissue procurement.
Functional test for smooth muscle contractility
The contractile response to a single dose of potassium chloride (KCl, 120 mmol/L) was tested within 30 minutes of tissue harvest as described previously. To normalize for differences in tissue quantity, the data were expressed as force per tissue volume of each strip (mN/mm 3 ).
Immunofluorescent labeling of α-smooth muscle actin and in situ terminal deoxynucleotidyl transferase dUTP nick end labeling (TUNEL) labeling of cell apoptosis
GVCs were cryosectioned (7 μm) perpendicular to the longitudinal axis of the vagina. Hematoxylin-eosin and Masson trichrome staining were performed following manufacturer’s protocols (Sigma-Aldrich, St Louis, MO). For analysis, specimens were required to contain all layers of vagina, and those obliquely sectioned with subepithelium >1039 μm (mean of sham values that were sectioned perpendicularly plus 2SD) were excluded. One sample from composite mesh was excluded for not containing all layers of vagina.
Immunofluorescent labeling of α-smooth muscle actin and in situ TUNEL labeling of apoptotic cells were used to measure thickness of the subepithelial and smooth muscle layers, and the percentage of apoptotic cells in each vaginal layer as previously described.
Biochemical assays
Total collagen content and sulfated GAG content were measured as previously described, and expressed as percentage of tissue dry weight.
Mature elastin content was measured as the total content of desmosine and isodesmosine by ultra high-performance liquid chromography (UPLC). Briefly, hydrolyzed tissue was applied to Oasis mixed-mode cation-exchange (MCX) solid-phase extraction (SPE) columns (Waters, Milford, MA), and eluted with ammonium hydroxide/water/methanol (10/40/50). Stock solutions of desmosine, isodesmosine, and S-[beta-(4-pyridyl)ethyl]L-cysteine (S-LC, internal standard) (Santa Cruz Biotechnology Inc, Dallas, TX) were diluted to series concentration to create standard curves. S-LC (2 μmol) was added to each sample and standards as an internal control. A total of 3 μL of the prepared samples and standards were injected onto a prepared Waters Acquity UPLC ethylene bridged hybrid (BEH) C18 column and monitored by measuring absorbance at 270-nm wavelength. The elution time of isodesmosine was 1.4 minutes, desmosine was 1.9 minutes, and S-LC was 2.4 minutes. The amount of mature elastin in each sample was represented by the sum of desmosine and isodesmosine normalized by that for bovine elastin (Sigma-Aldrich).
Ratios of collagen subtype III/I in the tissue of GVC were determined as previously described and calculated as α1(III) ×2/α1(I) ×3 normalized by the ratio of purified collagen I/III.
Statistical analysis
Sample size was determined using previously published mechanical data (ultimate load and stiffness) for sham and Gynemesh, in which at least 8 animals were needed in each group to achieve significance with α error of 0.05 and power of 0.80. Data were tested for distribution before statistical analysis. For normally distributed data, a 1-way analysis of variance was used followed by the appropriate post-hoc tests including Dunnett for comparison to sham and pairwise test using Bonferroni multiple comparisons procedure between all groups. For nonparametric data, Kruskal-Wallis was used to compare overall significance and Mann-Whitney to compare between groups.
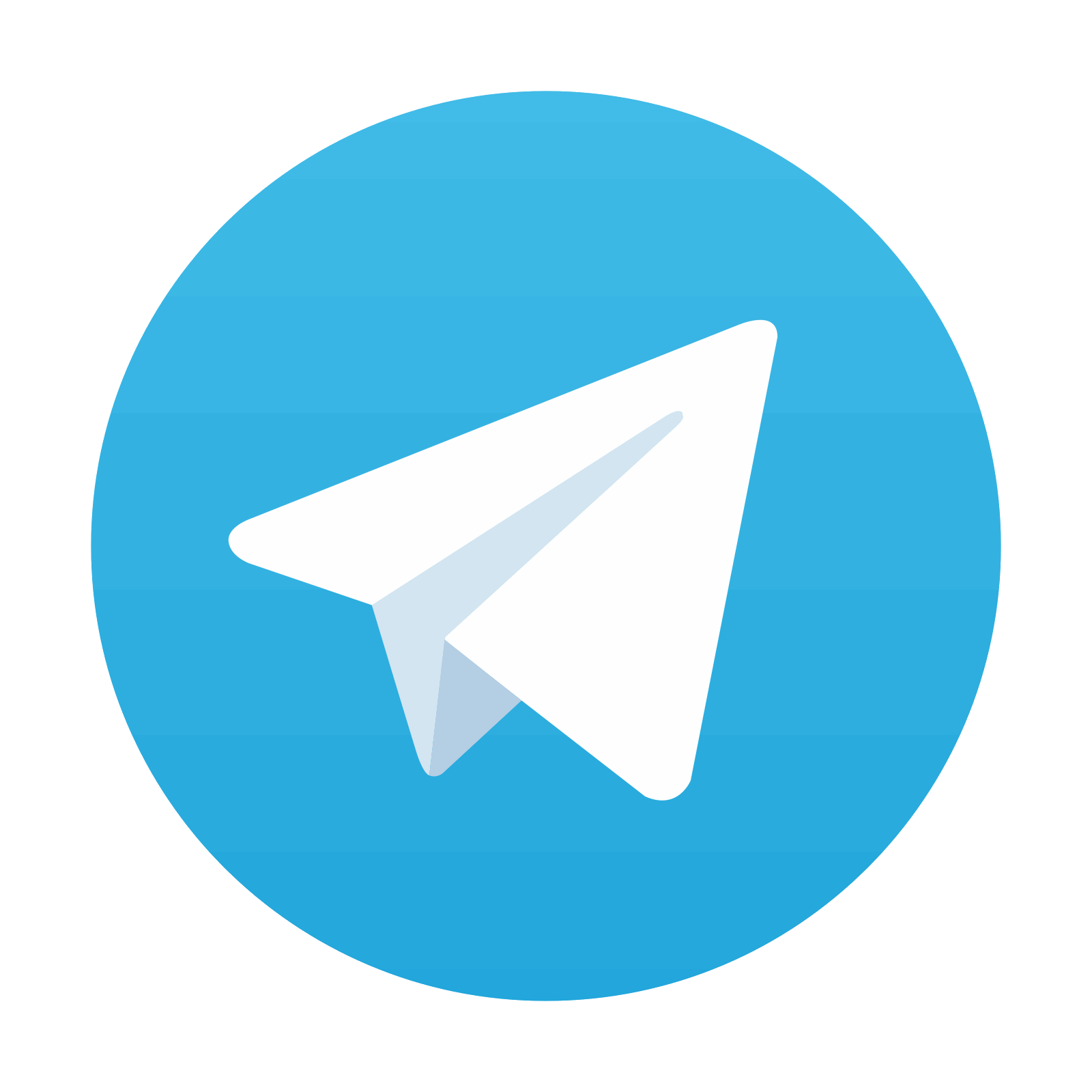
Stay updated, free articles. Join our Telegram channel

Full access? Get Clinical Tree
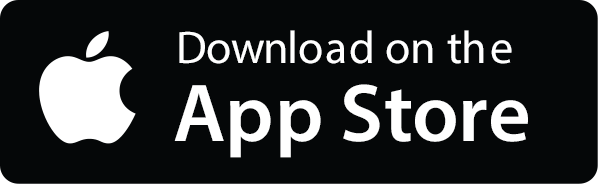
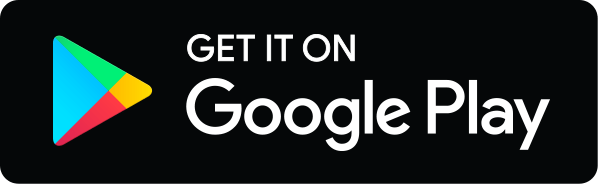