Key Points
- •
This chapter describes the novel concept of expanded carrier screening (ECS), whereby individuals are simultaneously screened for up to 200 genetic conditions.
- •
Different laboratory techniques are used in ECS and include targeted genotyping and next-generation sequencing approaches.
- •
The choice of conditions to be included in ECS varies among laboratories, and no consensus presently exists.
- •
Various screening strategies exist, including premarital carrier screening, cascade screening, stepwise screening and couple screening.
- •
An important aspect in offering ECS is patient education.
Basic Principles of Carrier Screening
In 1968, the World Health Organization (WHO) proposed guidelines for establishment of screening programs ( Box 26.1 ). These initial guidelines provided general recommendations regarding the types of disorders appropriate for screening. These recommendations predated the advent of genetic testing. Thus, in 1998, the WHO proposed modified guidelines that would specifically apply to genetic screening ( Box 26.2 ).
- 1.
Is the disease an important health problem?
- 2.
Is there a recognisable latent or early symptomatic stage?
- 3.
Do we know the natural history of disease?
- 4.
Is there an effective treatment for patients with recognised disease?
- 5.
Is there a suitable test that will identify the disease in its early stages?
- 6.
Is the test acceptable to the population?
- 7.
Do we agree on who treats the disease?
- 8.
Are facilities for diagnosis and treatment available?
- 9.
Case finding should be ongoing.
- 10.
The cost of case finding (including diagnosis and treatment) should be economically balanced in relation to possible expenditures on medical care as a whole.
- •
Genetic screening should be voluntary, not mandatory.
- •
Genetic screening should be preceded by adequate information about the purpose and possible outcomes of the screen or test and potential choices to be made.
- •
Anonymous screening for epidemiologic purposes may be conducted after notification of the population to be screened.
- •
Results should not be disclosed to employers, insurers, schools or others without the individual’s consent to avoid possible discrimination.
- •
In rare cases in which disclosure may be in the best interests of the individual or of public safety, the health provider may work with the individual towards a decision by him or her.
- •
Test results should be followed by genetic counselling, particularly when they are unfavourable.
- •
If treatment or prevention exists or is available, this should be offered with a minimum of delay.
- •
Newborn screening should be mandatory and free of charge if early diagnosis and treatment will benefit the newborn.
In 2006, the WHO stated that genetic prevention services are needed to effectively reduce the burden of congenital and genetic disorders. Yet in many countries, the level of genetic services is currently inadequate and insufficient. Frequently, these services are available only to the wealthy and well-educated. Indeed, the provision of genetic services must be weighed responsibly and fairly against the competing health requirements in each country. However, epidemiologic data regarding the prevalence of congenital and genetic disorders and cost-effectiveness analyses of screening programs indicate that many countries would actually benefit from incorporating preventive genetic approaches into their health services. Unfortunately, despite the growing number of genetic technologies that hold great promise, these are underused in clinical practice and fail to reduce the burden of inherited diseases. It is at this juncture that the introduction of expanded carrier screening (ECS) may provide a feasible universal solution to these shortcomings.
The Evolution of Carrier Screening
Screening for Tay-Sachs Disease Using Enzyme Analysis
One of the first successful programs has been the community-wide screening for Tay-Sachs disease (TSD) carriers, established in the 1970s among Eastern European (Ashkenazi) Jews. TSD is caused by deficiency of β-hexosaminidase A activity, which is inherited in an autosomal recessive (AR) manner ( Fig. 26.1 ). Unaffected heterozygote carriers may be ascertained by detection of reduced enzymatic activity in serum and blood lymphocytes. Wide-scale voluntary screening programs for TSD were initiated among Ashkenazi Jews first in the United States, Canada and Israel but were eventually adopted in many countries worldwide. In the first 30 years after the implementation of these programs, more than 1.4 million individuals were screened (mostly from North America and Israel), and more than 1400 couples have been identified to be at risk for offspring affected with TSD. These programs resulted in a significant reduction in the incidence of TSD among Ashkenazi Jews, with the majority of affected individuals being from other unscreened ethnicities.

Screening for β-Thalassemia Using Red Blood Cell Indices
β-Thalassemia is a relatively common condition among individuals of Middle Eastern and Mediterranean origin. It is characterised by deficient synthesis of β-haemoglobin chains, resulting in severe anaemia. Heterozygote carriers have mild anaemia and reduced red blood cell indices. Screening is based on the results of complete blood count (CBC) showing a low mean corpuscular volume (MCV) and low mean corpuscular haemoglobin (MCH). Haemoglobin electrophoresis is used to differentiate carriers from those with iron deficiency, with high sensitivity and relatively good correlation with phenotype. Because the CBC is relatively inexpensive, easy and widely available, screening programs for β-thalassemia are widely available. For a detailed description, see Chapter 27 .
Genetic Screening Using Molecular Techniques
Screening methods for both TSD and β-thalassemia were initially based on nonmolecular testing (enzymatic testing and CBC, respectively). Currently, however, most carrier screening programs are based on molecular methodologies. Screening for cystic fibrosis (CF) has become the prototype model, following the discovery of the causative role of the CFTR gene in 1989. CF is an AR disorder characterised by progressive lung disease, pancreatic dysfunction and male infertility. It is one of the most common severe genetic diseases in caucasians, with a carrier frequency of about 1 in 25. Although more than 2000 mutations have been described in the CFTR gene, each ethnic group may have a unique set of mutations, with the delF508 mutation being the most prevalent and accounting for about 70% of CF alleles. In 2001 the American College of Obstetricians and Gynecologists (ACOG) and the American College of Medical Genetics (ACMG) set guidelines for prenatal and preconception CF carrier screening. Initial guidelines recommended limiting screening to caucassian individuals or those with a family history of CF. However, in 2011, the guidelines were updated, stating that it has become increasingly difficult to classify individuals with CF into distinct ethnic categories. Thus the Committee agreed that offering CF screening to all couples planning a pregnancy is reasonable. For couples in whom both partners are carriers of mutations in the same AR gene, there is a reproductive risk of 25% for affected offspring. Knowing this before delivery provides an opportunity for genetic counselling during which reproductive options such as prenatal diagnosis by chorionic villus sampling or amniocentesis may be discussed. Knowing the carrier status before pregnancy also provides the option of preimplantation genetic diagnosis of embryos attained by in vitro fertilisation.
Panethnic Screening
Although many genetic disorders have an ethnic predilection, only a few are universally frequent in diverse populations. One such example is spinal muscular atrophy (SMA), a severe AR neuromuscular disease caused by degeneration of motor neurons in the spinal cord, resulting in progressive muscle weakness and paralysis. It is for this reason that the ACMG recommends that carrier screening be offered to all couples, regardless of race or ethnicity. The same is also true for some CF mutations (e.g. delF508 mutation), which account for approximately 70% of CF alleles worldwide. This mutation is thought to have occurred more than 52,000 years ago, in a population genetically distinct from any present European group. Panethnic screening for CF is currently recommended by ACOG and ACMG. Similarly, fragile X syndrome (FXS), a condition associated with intellectual disability (ID) and autistic behaviour, is prevalent in most populations. Indeed, it is the most common cause of inherited mental retardation. The prevalence of the fragile X syndrome is estimated to be about 1 in 4000 males and about 1 in 7000 females. The clinical phenotype in males includes varying degrees of ID with mild dysmorphic features such as relative macrocephaly, large ears, a prominent jaw and macro-orchidism after puberty. Affected females usually present with a less severe phenotype and may exhibit only subtle cognitive impairment. The disorder is caused by a CGG triplet repeat expansion mutation in the FMR1 gene on chromosome Xq27.3. Unaffected individuals usually have less than 55 CGG repeats. Individuals with the fragile X syndrome have more than 200 CGG repeats, a full mutation. Individuals with 55 to 200 CGG repeats are considered to have a premutation . Female premutation carriers are at risk for having offspring with further CGG expansion, which may ultimately result in a full mutation (>200 repeats). There is a strong correlation between the number of CGG repeats in the carrier mother and the risk for expansion to full mutation in the offspring. Population-based carrier screening for fragile X has been widely used in Israel since the mid-1990s. It is now included in most basic screening panels for all ethnicities.
Screening Panels for Multiple Disorders
In the 1990s and 2000s an increasing number of gene discoveries have led to a growing number of conditions available for screening. This has been particularly the case in the rather homogeneous Ashkenazi Jewish population in Israel and North America. These included Canavan disease, Gaucher disease, familial dysautonomia, Fanconi anaemia group C, Niemann-Pick disease type A, mucolipidosis type IV, Bloom syndrome and many others.
These discoveries ushered in the introduction of what has become known as the Ashkenazi Jewish Genetic Panel (AJGP). The ACOG initially recommended that individuals of Eastern European Jewish (Ashkenazi) ancestry be offered carrier screening for TSD and CF as part of routine obstetric care. The current recommendations of ACOG and ACMG for the AJGP include only 4 or 9 diseases, respectively. However, the content of AJGPs in many laboratories has continued to expand, and some current AJGP offerings include several dozen conditions. Although many of the diseases included in the AJGP are individually rare, cumulatively, they do amount to a significant disease burden. A study conducted by Mount Sinai Laboratory demonstrated that additively, the risk for being a carrier of at least one condition on a 16-disease AJGP is at least 30%.
Although the AJGP has a high yield in the Ashkenazi Jewish population, with a few exceptions, it is not very effective among non-Ashkenazi Jews and even less so in non-Jewish populations. This is because most mutations in this panel are unique founder mutations, likely the result of genetic drift following a bottleneck of this ethnic group.
The increase in the number of genetic conditions amenable to screening, the complexity of designing ethnic-specific screening panels and dramatic technological innovations have highlighted the fact that current screening strategies are inadequate and may, in fact, become obsolete. This is further complicated by the fact that the rapid population fluxes characteristic of this era result in increasing ethnic admixtures to the point where individuals have become unaware of their precise ethnic background. These considerations have resulted in the paradigm shift that is ECS. In this approach, hundreds of conditions are screened for simultaneously using a uniform platform. The test is offered to all, regardless of stated ancestry. In this chapter, different aspects of ECS are discussed.
Expanded Carrier Screening
In 2005 the ACOG updated its recommendation to justify panethnic screening for specific disorders such as CF because ‘it is becoming increasingly difficult to assign a single ethnicity to individuals’. In fact, approximately 12% of newborns diagnosed with β-haemoglobinopathies by newborn screening during the 1990s in California were not from the groups allocated in the ACOG carrier screening guidelines. This reasoning called for extending the screening programs for haemoglobinopathies, and potentially other conditions, to more ethnically diverse populations.
It is estimated that 18% to 20% of infant hospitalisations and deaths can be attributed to Mendelian diseases. Offering screening for only a limited number of conditions, if at all, does little to reduce the overall burden of these Mendelian disorders, many of which could be averted by offering ECS to the entire population. The rapidly expanding list of disorders that can now be identified makes ECS a reasonable option, especially as novel sequencing technologies enable relatively inexpensive analysis of many AR diseases simultaneously.
These assumptions have already been confirmed in several studies. Lazarin and coworkers described a targeted mutation panel which includes 417 mutations associated with 108 genetic diseases. In their cohort of more than 23,000 patients of mixed ethnicities, a carrier status of at least one mutation was identified in 24% of cases. Despite the rarity of each individual disorder included in the panel, the overall risk for having an affected offspring was calculated at 1 in 280. The authors argue that this risk exceeds that of having an offspring with a neural tube defect (NTD), for which screening is universally accepted. Comparable findings were found in an even larger study analysing the results of 322,484 patients undergoing routine carrier testing by ECS using a panel of 88 severe or profound recessive diseases in which the frequency of affected pregnancies was approximately 1 in 550. They noted that the number of patients needed-to-screen to detect one affected case was lower with ECS compared with standard screening for Down syndrome or NTDs.
In 2017 ACOG reviewed the role of ECS and suggested ‘that ethnic-specific, panethnic, and ECS are acceptable strategies for prepregnancy and prenatal carrier screening’. Each obstetrician–gynaecologist or other health care provider or practice should establish a standard approach that is consistently offered to and discussed with each patient, ideally before pregnancy. After counselling, a patient may decline any or all carrier screening. If a patient requests a screening strategy other than the one used by the obstetrician–gynaecologist or other health care provider, the requested test should be made available to her after counselling on its limitations, benefits and alternatives.
The European Society of Human Genetics has developed and published recommendations regarding responsible implementation of ECS. It confirms that the primary goal of carrier screening is to facilitate informed reproductive decision making by identifying couples at risk for having an affected child with an (autosomal or X-linked) recessive disorder. ECS allows testing of all individuals regardless of ancestry or geographic origin (‘pan-ethnic’ or ‘universal’), which in this respect increases equity and reduces the chance of stigmatisation. The best time to offer screening is during the preconception period because identifying carrier couples before pregnancy allows the greatest number of options with more time to make an informed decision. However, responsible implementation of expanded genetic carrier screening raises many technical, ethical, legal and social questions.
Laboratory Methodologies
Two main methodologies are used for ECS: targeted array-based genotyping and sequence analysis by next-generation sequencing (NGS). Each have specific advantages and disadvantages ( Table 26.1 ).
Targeted Array–Based Genotyping | Next-Generation Sequencing | |
---|---|---|
Pros |
|
|
Cons |
|
|
Targeted Array–Based Genotyping
Targeted genotyping is based on analysis of a preselected list of pathogenic variants (mutations), known to be causative of a particular disease. Such systems are often based on development of multiple fluorescently labelled sequences tags, each corresponding to a specific sequence variant. Most variants are straightforward biallelic single nucleotide polymorphisms (SNPs). However, specific modifications are also made to allow detection of more complex variants, such as triallelic SNPs, insertions and deletions, copy number variants and so on. Targeted array–based genotyping platforms enable the simultaneous analysis of hundreds of known pathogenic variants. Several validation studies, including those comparing a microarray-based platform to single-gene based methods, found similar analytical performance. Moreover, this method was found to be 100% concordant in a group of known mutation carriers, demonstrating that high-throughput targeted genotyping assays can accurately identify carriers in the tested population. Because targeted analysis includes only well-curated mutations with a known genotype–phenotype correlation, posttest counselling is relatively simple and uncomplicated for those detected as carriers. Conversely, targeted mutation analysis would miss rare pathogenic variants that have yet to be included in the panel. As a result, constant modifications may be required any time a novel pathogenic variant in a specific disease gene is discovered or when new genes need to be included in the panel. In addition, for many conditions, the pathogenic variants have been described only in a specific population. Thus targeted mutation analysis should be considered as comprehensive only for the ethnicities for which it was initially designed. It is for this reason that referral to targeted panels as ‘universal screening’ should be discouraged.
Next-Generation Sequencing
Next-generation sequencing is based on the ability to sequence, in parallel, millions of DNA fragments, and introduction of NGS technology has resulted in a dramatic increase in speed and content of sequencing at a fraction of the cost. Described briefly, first a DNA library is prepared from the patient’s sample by fragmentation, purification and amplification of the DNA sample. Individual fragments are then physically isolated by attachment to solid surfaces or small beads. The sequence of each of these fragments is resolved simultaneously by such techniques as sequencing by synthesis . The resulting sequence data are computationally aligned against a ‘normal reference’ genome. This enables the detection of many sequence alterations in a single reaction.
Next-generation sequencing–based screening has been shown to have high clinical sensitivity in the assayed genes. Mutation detection has been shown to have about 95% sensitivity and 100% specificity for a variety of alterations such as SNPs, insertions and deletions, splicing mutations and gross deletions. Proponents of NGS-based carrier screening claim that it shows high accuracy, precision, reproducibility and robustness for clinical use compared with the targeted mutation analysis. Because sequencing is performed throughout the genes of interest, unrecognised or rare pathogenic variants, not included in any targeted arrays, may be detected. This allows the implementation of carrier screening across a wider range of ethnically diverse populations, more closely approximating the term ‘universal’.
The cost of NGS based carrier screening, which in the past has been a major deterrent, is also gradually decreasing. Because most relevant sequence variants within a gene are detected, constant modifications are not required. Modelling a population of 1,000,000 couples that is representative of the US population would result in detection of 83,421 mutation carriers. It has been estimated that NGS-based screening would avert 21 additional affected births compared with screening by targeted genotyping. Cost saving would amount to approximately $13 million. Compared with no screening at all, NGS-based carrier screening would avert 223 additional affected births. The results are sensitive to assumptions regarding mutation detection rates and carrier frequencies in multiethnic populations.
Next-generation sequencing–based approaches have several shortcomings: some of the novel variants detected by NGS may have no clinical significance. For some variants, no clear genotype–phenotype correlation exists. Therefore this methodology requires robust bioinformatic capabilities that will allow accurate determination of the pathogenicity of each detected variant using a variety of in silico analyses as well as literature reviews. Laboratories usually limit their reports to include variants in classes 1 and 2 only, but for some variants, it may be difficult to make a call ( Table 26.2 ). This may put a strain on laboratory personnel, genetic counsellors and physicians alike. In such circumstances, the importance of pre- and posttesting counselling cannot be overemphasised.
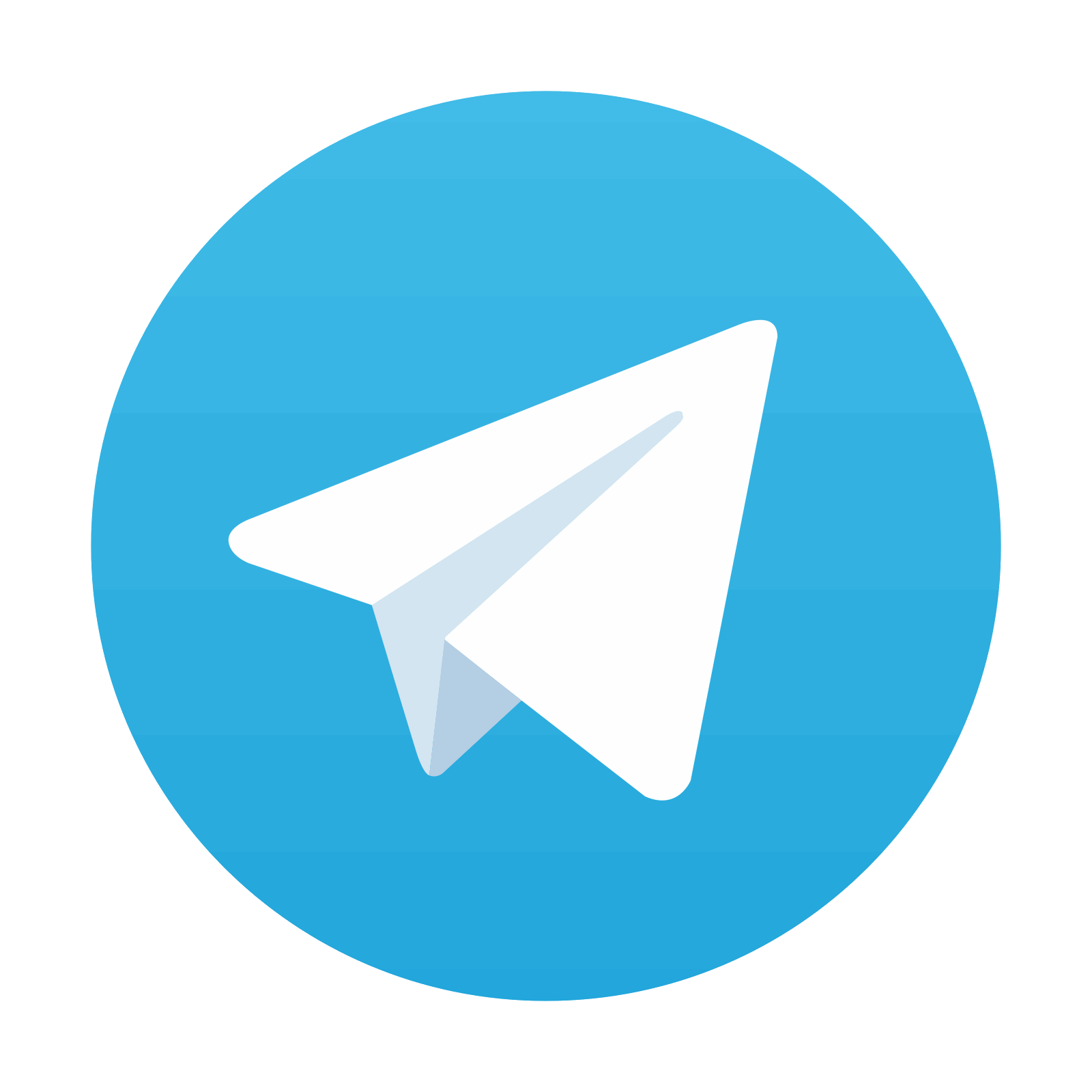
Stay updated, free articles. Join our Telegram channel

Full access? Get Clinical Tree
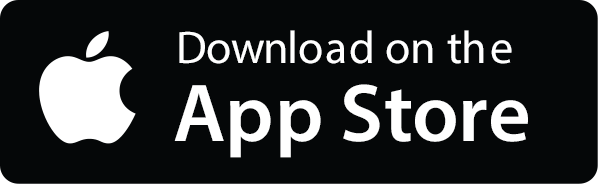
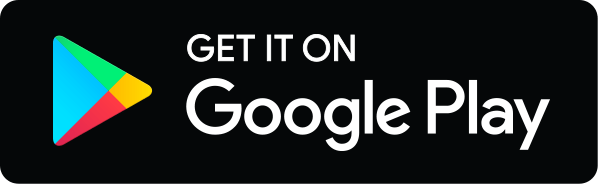