Diabetes Mellitus
Arlan L. Rosenbloom
CLASSIFICATION AND DIAGNOSIS
The diagnosis of diabetes includes a wide variety of diseases characterized by hyperglycemia. Because insulin is the only physiologically important hypoglycemic hormone, hyperglycemia is the result of either impaired secretion of insulin from the beta cells of the pancreas (type 1 diabetes) or resistance to the effect of insulin in the liver, muscle, and fat cells exceeding a limited capacity of the pancreas to compensate (type 2 diabetes). Criteria for the diagnosis of diabetes were revised in 2005 and prediabetes categories of impaired glucose tolerance and impaired fasting glucose added; this change reflected the recognition that these preclinical glucose intolerance states are associated with increased cardiovascular morbidity.4 The information in Table 544-1 is based on the current recommendations of the American Diabetes Association.4
ETIOLOGIC CLASSIFICATION OF DIABETES
Contemporary understanding of the pathogenesis of various forms of diabetes has made previous classification based on treatment inappropriate (non–insulin-dependent diabetes or NIDDM, insulin-dependent diabetes or IDDM for the principal forms).5Table 544-2 is adapted from the classification published by the American Diabetes Association expert committee first in 1997, then revised based on etiology in 1999.4
Type 1 Diabetes
Diabetes that occurs in childhood is predominantly immune-mediated type 1 diabetes (T1D) associated with histocompatibility locus (HLA) specificities. Idiopathic T1D may be difficult to distinguish from the immune-mediated form. Many, if not most, African American patients who have T1D without evidence of autoimmunity have what has been termed atypical diabetes mellitus or Flatbush diabetes.6,7 This condition has its onset throughout childhood. and rarely past age 40, and is not associated with HLA specificities. Further characteristics are described in Table 544-3.
Type 2 Diabetes
Type 2 diabetes (T2D) in childhood and adolescence accounts for 10% to 50% or more of new patients with diabetes in the 10- to 19-year-old age group, depending on the ethnic/racial mix of the population served.3,8
Monogenic Diabetes
Monogenic diabetes, formerly known as maturity onset diabetes of youth or MODY, is a stable form of youth-onset (under 25 years of age) diabetes inherited in an autosomal dominant fashion and affecting almost exclusively those of European ancestry (Table 544-3). Six molecular defects have been identified, affecting cell function. Its frequency among diabetes patients is reported to vary from less than 0.2% to 5% in various populations.9
Mitochondrial Mutations
Diabetes and deafness may be associated as a result of mutations in mitochondrial DNA.10-12
Genetic Defects in Insulin Action
Genetically determined abnormalities of insulin action as a cause of diabetes are very rare; the range of effects is from mild hyperinsulinemia with modest hyperglycemia to severe diabetes requiring massive amounts of insulin or treatment with recombinant insulin-like growth factor 1.13 In the insulin resistance of lipoatrophic diabetes, function of the insulin receptor is intact, indicating that the defect lies in postreceptor transduction.14
Table 544-1. Criteria for the Diagnosis of Diabetes
Symptoms plus casual* plasma glucose concentration ≥ 200 mg/dL (11 mmol/L), or |
Fasting plasma glucose ≥ 126 mg/dL (7 mmol/L), or |
2-hour plasma glucose ≥ 200 mg/dL (11 mmol/L) during an oral glucose tolerance test (OGTT). The test should be performed using a glucose load containing the equivalent of 75-g anhydrous glucose dissolved in water for those weighing > 43 kg and 1.75 g/kg for those weighing < 43 kg.** |
In the absence of marked hyperglycemia with decompensation, these criteria should be confirmed by repeat testing on a different day. OGTT is not recommended for routine clinical use. |
Impaired glucose tolerance = 2-hour plasma glucose 140 – 200 mg/dL |
Impaired fasting glucose = 100 – 125 mg/dL |
*Casual is defined as any time of day without regard to the time of the last meal.
**The oral glucose tolerance test should be done following 2–3 days of a high carbohydrate diet. In practice, however, obtaining a plasma glucose 2 hours after a high-carbohydrate breakfast may be more practical and as informative. The oral glucose tolerance test is recommended only for research purposes.
Diseases of the Exocrine Pancreas
Injury to the pancreas rarely results in diabetes because of the small volume occupied by the β cells, with the exception of carcinoma involving the pancreas or hemochromatosis (bronze diabetes), problems not seen in pediatrics. With improved survival, increasing numbers of patients with cystic fibrosis are developing diabetes.15
Endocrinopathies
Endocrine excess syndromes including acromegaly, Cushing syndrome, glucagonoma, and pheochromocytoma have been associated with diabetes in adults.16 There is concern about the use of pharmacologic doses of growth hormone in children who do not have growth hormone deficiency, which may be increasing the risk for type 2 diabetes.17,18
Drug- or Chemical-Induced Diabetes
Drugs frequently used to treat children can impair insulin secretion, increase gluconeogenesis, or increase insulin resistance, with resultant hyperglycemia or the precipitation of diabetes.19,20 The rat poison Vacor as well as pentamidine (given intravenously), can permanently destroy β cells.21-24 Atypical antipsychotic drugs (clozapine, olanzapine, quetiapine, risperidone) have been associated with precipitation of diabetes and ketoacidosis, often with clearing of the diabetes after the drug is stopped.25,26 Exact mechanisms are uncertain, but β cell toxicity and weight gain with insulin resistance have been implicated. Other drugs can impair insulin action, probably the most common being glucocorticoids.19,20
Table 544-2. Etiologic Classification of Diabetes Mellitus
Infections
Numerous viruses have been implicated in the induction of diabetes; the strongest evidence of a direct causation comes from the experience with congenital rubella,27 fortunately now rarely seen.
DIFFERENTIAL DIAGNOSIS
Table 544-3 describes the characteristic features of the common types of diabetes seen in childhood, and Table 544-4 summarizes clinical characteristics helpful in distinguishing type 1 diabetes (T1D) and type 2 diabetes (T2D) in children and adolescents. In those with acute onset who are not obese and not African American, T1D is highly likely, and further testing is not necessary. Non-obese African American youngsters with acute onset diabetes who have a 3-generation family history of diabetes indicative of autosomal dominant transmission and who do not have islet autoimmunity markers are very likely to have atypical diabetes mellitus. Islet cell autoimmunity testing should be considered in obese patients having acute onset diabetes; if this is not practical or if the patient has acanthosis nigri-cans, the ability to reduce and stop the acutely required insulin over the first several months, with weight reduction, exercise, and oral hypoglycemic therapy as necessary, will clarify the diagnosis.
Obese individuals with insidious onset can be considered to have T2D. If the patient is lean, islet autoantibody testing will be helpful and, if positive, indicate early detection of T1D. Negative islet cell autoimmunity in the nonobese patient may indicate monogenic diabetes, in which case testing of family members will be productive. Unless apparently unaffected family members are tested, the pattern of autosomal dominant transmission may be missed. Fasting C-peptide or insulin measurements can be of value, with elevated levels indicative of T2D. Normal levels, however, may also occur during the honeymoon period of T1D and suppressed levels can be the result of glucose toxicity. Repeated testing after 1 year or more may be needed. A patient with persistent normal C-peptide levels can be treated as with T2D, regardless of the presence or absence of diabetes-related autoimmunity.
A study of 700 newly diagnosed 5- to 19-year-old patients from 3 university centers in Florida over a 5-year period indicated that 3% of those initially classified as T1D (17/605) were later classified as T2D and that 8% of those initially diagnosed as T2D were subsequently reclassified as having T1D (6/77).28 In this relatively sophisticated clinical setting, the proportion of patients in whom classification may be problematic was less than 5% of newly diagnosed children and youth.
The distinctions indicated by the typical features in Tables 544-3 and 544-4 are not always as certain as one would like. There are a number of reasons for classification difficulty. With the increased frequency of obesity in childhood, a substantial number of newly diagnosed T1D and atypical diabetes mellitus patients will be obese, suggesting the possibility of T2D. Insulin or C-peptide measurements at the onset of diabetes may not be helpful because there may be reasonable β-cell function during the recovery phase (“honeymoon”) of autoimmune diabetes, and conversely, glucose toxicity/lipotoxicity in non–autoimmune diabetes will impair insulin secretion at the time of testing. Because some T2D patients and almost all atypical diabetes mellitus patients have ketoacidosis, this feature is not helpful to distinguish non–T1D from T1D.
Despite uncertainties in our classification of diabetes, the clinician dealing with the individual patient should always base treatment on individual characteristics rather than absolute diagnostic classification.
TYPE 1 DIABETES
EPIDEMIOLOGY
eFigure 544.1 shows the incidence of T1D diabetes in various countries worldwide. Incidence rates in European countries have steadily increased from the 1950s.43-45 Similar increases worldwide as countries undergo development emphasizes the influence of environment on T1D.
Table 544-3. Classification of the Types of Diabetes Seen in Children
The average age of onset of diabetes is thought to be decreasing.48-50 Modest seasonality has been noted, with a peak in winter months and a summertime dip in both the northern and southern hemispheres.44,51,52
Table 544-4. Differentiating Type 1 from Type 2 Diabetes in Children and Adolescents
ETIOLOGY AND PREVENTION
Observations that type 1 diabetes (T1D) had a long latency period began almost 4 decades ago.53,54 Subsequent identification of genetic markers (HLA haplotypes), autoimmune markers reflecting islet cell destruction, and early biochemical abnormalities preceding frank diabetes have greatly improved predictability of T1D. These studies have reinforced the long-held view that T1D develops in genetically predisposed individuals who are exposed to one or more environmental influences resulting in autoimmune destruction of β cells by autoreactive T cells (Fig. 544-1).35,55 The injury to the β cells releases antigens perceived as foreign proteins by the immune system, which produces antibodies against them. These autoantibodies can be assayed as a reflection of the damage, but they are not the cause of it. Insulin autoantibodies (IAA), islet cell antibodies (ICA), glutamic acid decarboxylase (GAD65) antibodies, and antibodies to tyrosine phosphatase (IA-2 or ICA512) are indicators of ongoing autoimmune disease and may be present for many years before the development of overt diabetes. GAD65 antibodies can be detected in 75% of newly diagnosed diabetes patients56 and IA-2 in 50% to 60% of patients.57 The number of different autoantibodies and their titers are independent predictors of T1D risk, which also depends on the age at which they are detected.
FIGURE 544-1. Modern model of the pathogenesis and natural history of type 1 diabetes. The modern model expands and updates the traditional model by inclusion of information gained through an improved understanding of the roles for genetics, immunology, and environment in the natural history of type 1 diabetes. FPIR, first phase insulin response; GADA, glutamic acid decarboxylase autoantibodies; IAA, insulin autoantibodies; ICA, islet cell autoantibodies; ICA512A, autoantibodies against the islet tyrosine phosphatase; IVGTT, intravenous glucose tolerance test. (Source: Adapted from Atkinson MA, Eisenbarth GS. Type 1 diabetes: new perspectives on disease pathogenesis and treatment. Lancet. 2001;358(9277):221-229, with permission.)
Outside of the research setting and for the development of accurate type 1 diabetes (T1D) prediction models, diabetes-specific autoanti-body testing is seldom necessary in diabetes practice. Such testing may be helpful in differentiating new onset T1D from T2D in the obese individual with characteristics of T1D, although even in this situation, individualized treatment is based on the clinical status rather than the specific diagnosis. Antibody testing may also be helpful for determining diabetes risk in individuals with stress- or drug-induced hyperglycemia.
The role of environmental factors in the development of T1D is supported by the finding that 30% of identical monozygotic twins are concordant for the disease67 and by the rising incidence with decreasing mean age of onset.37,47,49,50 Various environmental factors that have been implicated include congenital rubella infection,68 enteroviral infection,69,70 reduced exposure to early infection,71 and vitamin D deficiency.72 There is no evidence to support the notion that standard vaccination increases risk for T1D.73
An influence of cow’s milk protein on the development of T1D is based on the structural similarity of a 17-amino acid fragment (ABBOS) of bovine serum albumin to an islet antigen (ICA 69).74 The Diabetes Autoimmunity Study of the Young (DAISY) found no association between islet autoimmunity and early cow’s milk exposure.75 A more robust double-blind, controlled study is underway that will determine if avoidance of nutritional cow’s milk proteins for at least the first 6 months of life will reduce the incidence of diabetes.77 Investigators in Sweden and Finland found IgM antibodies to enterovirus more frequently in pregnant women whose children developed T1D than in control women.78,79 There is molecular mimicry between the P2-D protein of coxsackie virus and the GAD protein. Other viruses implicated include chick-enpox, mumps, measles, ECHO, rotavirus, and maternal rubella.78,80 The evidence is most convincing for congenital rubella, with 20% of infected children islet cell antibodies-positive.68
CLINICAL FEATURES
Type 1 diabetes (T1D) is usually diagnosed in a child with polydipsia, polyuria, weight loss, and decreased energy. Polyphagia is often absent, as ketosis can cause anorexia. With insulin deficiency, glucose utilization is impaired, resulting in elevated blood glucose concentrations that exceed the renal threshold for reabsorption (∼ 180 mg/dL), producing osmotic diuresis and glycosuria. The tissues perceive starvation because of absence of intracellular glucose, causing the release of triglycerides from fat stores and protein from muscle. These substrates are processed by the liver to yield ketones and more glucose. The tissue breakdown leads to weight loss and glycosuria to polyuria and polydipsia. Insulin deficiency, dehydration, and ketosis will progress to diabetic ketoacidosis before diagnosis in 20% to 40% of all newly diagnosed T1D.81
The primary care physician is likely to see an child who appears to be ill for evaluation of weight loss and fatigue or nonspecific symptoms of dehydration, including constipation, headache, and abdominal pain. Diagnosis is often missed in emergency rooms or doctors’ offices because the symptoms are attributed to a viral syndrome or gastroenteritis if there is vomiting. Children who are vomiting but do not have diarrhea and are maintaining high urine output despite dehydration have T1D. Other causes for this combination of symptoms are rare (adrenal insufficiency, renal disease). Enuresis in a previously toilet-trained child, nocturia, pyogenic skin infections, recurrent candida diaper rash in babies and toddlers, and monilial vaginitis in teenagers also require consideration of diabetes.
Children in whom diabetes must be considered in addition to those who have typical signs and symptoms include those who have glucosuria without hyperglycemia and those with transient hyperglycemia.
Renal glucosuria may be an isolated congenital disorder, a feature of the Fanconi syndrome and other renal tubular disorders, due to severe heavy metal intoxication, due to ingestion of certain drugs (eg, outdated tetracycline), or result from inborn errors of metabolism such as cystinosis. Starvation ketosis may occur in these conditions with gastrointestinal disturbance, but the absence of hyperglycemia eliminates the possibility of diabetes. Stress of illness, usually acute asthma exacerbation or severe infection, especially in toddlers, may be associated with moderate to severe hyperglycemia and ketosis.82,83 A similar clinical picture can be seen with glucocorticoid administration.84,85 The presence of islet cell antibodies, IA-2, insulin autoantibodies, or GAD antibodies in those with stress or glucocorticoid-induced hyperglycemia may indicate limited β cell reserve and risk for future development of diabetes.
DIABETIC KETOACIDOSIS
Complications of diabetic ketoacidosis account for most of the diabetes-related morbidity and mortality in childhood T1D81 diabetic ketoacidosis (DKA) is defined by venous pH less than 7.3 or serum bicarbonate concentration less than 15 mmol/L, with serum glucose concentration greater than 200 mg/dL (11 mmol/L) and is typically associated with glucosuria, ketonuria, and ketonemia.86 Rarely, DKA may occur with normal circulating glucose concentrations if there has been partial treatment or with pregnancy.87,88
PATHOPHYSIOLOGY
See Figure 544-2. Diabetic ketoacidosis (DKA) occurs when there is a critical net effective deficit of insulin, resulting in intracellular starvation, stimulating the release of the counterregulatory hormones glucagon, catecholamines, cortisol, and growth hormone. The counterregulatory hormonal responses stimulate hepatic and renal glucose production and reduce glucose utilization in insulin-dependent peripheral tissues (muscle, liver, adipose), which results in hyper-glycemia, release of free fatty acids into the circulation from adipose tissue (lipolysis), and unrestrained hepatic fatty acid oxidation to ke-tone bodies.100
MORBIDITY AND MORTALITY
Similar mortality risks for each episode of DKA have been reported from the United States (0.15%),101 Canada (0.18–0.25%),102,103 and the United Kingdom (0.31%).104 Cerebral edema accounts for 60% to 100% of this mortality.105-110 Other causes of death or disability with DKA in addition to cerebral edema include hypokalemia, hypophosphatemia, hypoglycemia, other intracerebral complications, peripheral venous thrombosis, mucormycosis, rhabdomyolysis, acute pancreatitis, acute renal failure, sepsis, aspiration pneumonia, and other pulmonary complications.81
CEREBRAL EDEMA IN DIABETIC KETOACIDOSIS 
The North American multicenter study reported a cerebral edema incidence of 0.9%.107 There were 13 verified cases of cerebral edema in a prospective surveillance study in Canada of patients younger than 16 years of age with DKA, for a rate of 0.51% of episodes of DKA.103
In 1990 the first extensive series, analyzing 69 cases, including 40 from the literature, found a case fatality rate of 64% overall, with 14% surviving without disability, 9% with disability that did not preclude independence, and 13% with severe disability or vegetative state. The case fatality was only 30% in those who were treated before respiratory arrest, even if this was at the gasping stage, with 30% surviving without disability, 26% with disability not precluding independence, and 13% with severe disability or vegetative state.105 Although the intervention in these cases was often after severe neurologic compromise and inadequate, the outcome with treatment is similar to those in contemporary population-based studies (eTable 544.1 ). A recent report from Canada documented that 19% of cases of cerebral edema were present before treatment was undertaken.103,105-107
Risk Factors
Young age, especially younger than 5 years, carries an increased risk for the development of cerebral edema. This may reflect the greater severity at presentation in this age group due to delay in diagnosis because of the nonspecificity of symptoms. The younger brain might also be more susceptible to metabolic and vascular changes associated with diabetic ketoacidosis. Severity of acidosis, degree of hypocapnia, and elevated serum urea nitrogen, indicators of severity of ketoacidosis and dehydration, have been noted to be risk factors.81 Infusion of sodium bicarbonate has been a significant association with the development of cerebral edema in 1 population-based case-control study.107 It is uncertain if this finding was due to a greater degree of severity of ketoacidosis or was an effect of the bicarbonate itself.133,134
Cerebral edema associated with diabetic ketoacidosis is peculiar to the pediatric age group and is almost nonexistent in the adult population.131 In adult patients with hyperglycemic hyperosmolar state, this is true despite rapid rehydration and restoration of normal glycemia.132
In several studies increased risk for cerebral edema has been associated with an inadequate increase in serum sodium concentration during treatment for diabetic ketoacidosis. This may reflect dysregulation of antidiuretic hormone secretion as a result of changes in the brain in those who are in the early, presymptomatic, stages of cerebral edema.107,133 The absence of evidence of associations between volume or tonicity of fluids or the rate of change in serum glucose and risk of cerebral edema indicates that this might be the result of altered renal sodium handling due to cerebral injury, rather than the type or rate of fluid administration.81,103,105,128,133
In the 61 children with cerebral edema from the North American multicenter study,107 poor outcome was associated with greater neurologic depression at the time of diagnosis of their cerebral edema, and with initial serum urea nitrogen concentration. Intubation with hyperventilation to a Pco2 less than 22 mm Hg was also a risk factor, demanding caution in hyperventilating affected patients.81,134
FIGURE 544-2. Mechanism of development of diabetic ketoacidosis.
Mechanisms of Cerebral Edema
Brain edema refers to an increase of cerebral tissue water causing an increase of tissue volume.135 The edema may be vasogenic, due to breakdown of the blood-brain barrier as around a tumor or with trauma; cytotoxic, from poisoning or metabolic derangement; or osmotic, as occurs with hyponatremia. Neither the cause nor the location of the fluid in the swollen brains of children with diabetic ketoacidosis–related or hyperglycemic hyperosmolar state–related cerebral edema is known. The mechanism is likely to be complex, and it may not be the same in all affected individuals, as reflected in the time of onset and the brain-imaging findings. The time of onset is distributed in a bimodal fashion, with approximately 2/3 of patients developing signs and symptoms in the first 6 to 7 hours and the rest from 10 to 24 hours after the start of treatment, with the early-onset individuals tending to be younger.107,133,134
A wide variety of imaging findings was reported in an analysis of initial brain computerized tomography (CT) scans done 2 to 44 hours after establishment of the diagnosis of cerebral edema; 39% had no acute abnormalities visible, and this did not differ significantly between early- and late-onset subjects; 26% had diffuse edema, which was also similar between early and late onset. Three of the 8 with diffuse edema also had hemorrhages, and 17% of the entire group had only subarachnoid or intraventricular hemorrhage. Focal brain injury in the mesial basal ganglia and thalamus, the periaqueductal gray matter, and the dorsal pontine nuclei was seen in 5 subjects (22%), and these localized injuries occurred only in the early-onset patients. These findings were not an artifact of the time that the studies were performed and thus truly reflect what appear to be widely varying pathology in the brain leading to the syndrome that is referred to as idiopathic cerebral edema.133 The observation that approximately 40% of initial brain-imaging studies in children who have cerebral edema are normal emphasizes that cerebral edema is a clinical diagnosis that requires treatment be started before imaging studies are undertaken.
Alterations in the brain with hyperglycemia and with diabetic ketoacidosis (DKA) have been explored using functional brain imaging. The functional imaging studies139 suggest that cerebral hypoperfusion before DKA treatment,136 followed by reperfusion, might lead to cerebral edema. The possibility remains that the initial and typical central nervous system (CNS) response to DKA is vasogenic edema, while symptomatic cerebral edema might require prolonged hypoxia or further insult (inappropriate vasopressin release, fluid overload, central hypoxia from rapid correction of peripheral acidosis with bicarbonate administration) leading to the superimposition of cellular edema.
Monitoring
Because treatment modification has not prevented cerebral edema and with the strong evidence that early administration of mannitol prevents brain damage and death from this complication, Muir et al. developed a model for early detection.133 The system allowed 92% sensitivity and 96% specificity for the recognition of cerebral edema early enough for intervention, using 1 diagnostic criterion, 2 major criteria, or 1 major plus 2 minor criteria (Table 544-5). This means that 5 youngsters will be treated for cerebral edema to prevent brain damage or death in 1 patient, a reasonable proposition considering the alternative of waiting for more stringent criteria to be met.
Treatment
While there can never be a controlled study of intervention, the response to rapid treatment with mannitol and associated measures as soon as cerebral edema is suspected, especially before respiratory arrest, has been repeatedly observed.81,105,117,128 Intervention measures include reduction in the rate of fluid administration, elevation of the head of the bed, and administration of intravenous mannitol in a dosage of 0.25 to 1.0 g/kg over 20 minutes, repeated as necessary in 1 to 2 hours. Although early intervention with mannitol treatment has improved outcome, it is difficult to determine to what degree, because increased recognition of the problem has undoubtedly led to less stringent case definition. The application of criteria developed by Muir et al. from the most severe cases, when applied to a series of 69 consecutive cases thought to be uncomplicated and experiencing full recovery, yielded 3 (4.3%) who would have been appropriately treated with mannitol according to these criteria.133 Indeed, this is the approximate percentage of diabetic ketoacidosis patients treated as cerebral edema in a recent report of 18 years’ experience.144
The effects of mannitol include lowering hematocrit and blood viscosity, which improves cerebral blood flow (CBF) and oxygenation, improvement in red cell deformability, vasoconstriction in areas of the brain with intact autoregulation or improved autoregulation from the effects of the mannitol on CBF and oxygenation, and direct osmotic effects with reduction in extracellular free water. Intensivists frequently express concern about the use of mannitol because of their experience with the risk of rebound edema and renal failure when mannitol is used over an extended period. There have been no reports, however, of any complications of mannitol use in this acute situation.
Hypertonic saline has been used to treat acute intracranial hypertension in head injury animal models and in patients undergoing surgical procedures in the supratentorial portion of the brain; in these circumstances it has been considered at least as effective as mannitol.145-151 The use of 3% saline in patients who have not responded adequately to mannitol infusion of a dose of 1.0 g/kg appears justified.
Table 544-5. Bedside Evaluation of Neurologic State of Children with Diabetic Ketoacidosis
Diagnostic Criteria for Cerebral Edema |
Abnormal motor or verbal response to pain |
Decorticate or decerebrate posture |
Cranial nerve palsy (especially III, IV, VI) |
Abnormal neurogenic respiratory pattern (eg, grunting, tachypnea, Cheyne-Stokes, apneustis) |
Diagnosis Also Suspected with Presence of 2 Major Criteria, or 1 Major Plus 2 Minor Criteria |
Major Criteria |
Altered mentation/fluctuating level of consciousness |
Sustained heart rate deceleration (decline more than 20 per minute) not attributable to improved intravascular volume or sleep state |
Age-inappropriate incontinence |
Minor Criteria |
Vomiting following initial treatment and its cessation, if present at admission |
Headache (recurrent and more severe than on admission) |
Lethargy or not easily aroused from sleep |
Diastolic blood pressure greater than 90 mm Hg |
Age less than 5 years old |
Adapted from Muir AB, Quisling RG, Yang MCK, Rosen-bloom AL. Cerebral edema in childhood diabetic ketoacidosis. Natural history, radiographic findings, and early identification. Diabetes Care. 2004;27:1541-1546.
Intubation is obviously necessary for those who have progressed to respiratory compromise, but should not otherwise be undertaken simply because of the diagnosis of cerebral edema. Aggressive hyperventilation was a significant risk factor for poor outcome in the study of Marcin et al.,134 similar to detrimental effects reported in head trauma and high-altitude exposure.153-154
OTHER COMPLICATIONS OF DIABETIC KETOACIDOSIS
Rhabdomyolysis
Rhabdomyolisis occurs with diabetic ketoacidosis (DKA) and is of uncertain etiology. Risk factors include severe hyperglycemia, high osmolality, and hypophosphatemia.
Mucormycosis
Mucormycosis is an acute, rapidly progressing, and often fatal facultative fungal infection that occurs in young patients with diabetes who have poor glycemic control and acute ketosis. The principal infection locus may be rhinocerebral, pulmonary, cutaneous, gastrointestinal, or in the central nervous system, and may be disseminated. In 1 study of 5 patients, which included only 1 survivor who had severe neurologic disability, predictors of increased susceptibility to infection with mucormycosis were African American and had a history of poor compliance, as evidenced by poor clinic attendance, risk-taking behaviors, and high rate of hospital admission.111 Impaired neutrophil function, vascular insufficiency, and, in DKA, the acid environment and high blood glucose concentration favoring fungal growth, contribute to the predisposition to mucor infection in patients with diabetes.
Acute Pancreatitis
Pancreatitis is a well-recognized complication of DKA in adults but is unusual in childhood type 1 diabetes (T1D). Elevation of serum levels of amylase and lipase was common in 50 children with DKA at onset of T1D. The elevation of serum lipase was directly associated with degree of acidosis. Acute pancreatitis was demonstrated by abdominal computerized tomography scan in 1 patient with persistent abdominal pain.112 Acute pancreatitis must be considered with abdominal pain that does not resolve with correction of acidosis.
Deep Venous Thrombosis
Deep venous thrombosis (DVT) has been reported in 4 of 8 children with DKA who required femoral central venous catheter (CVC) placement113. Coagulation factor abnormalities have not been demonstrated in children with diabetes in the absence of DKA115 but von Willebrand factor activity remains elevated at 120 hours following admission for DKA.116 This suggests that DKA and its treatment may result in a prothrombotic state and activation of vascular endothelium, predisposing to thrombosis.
Intracerebral Complications Other Than Idiopathic Cerebral Edema
Neurologic complications occur in an estimated 10% of all instances of neurologic collapse during ketoacidosis.81,119-121 The causes include subarachnoid hemorrhage,105 basilar artery thrombosis,105 cerebral venous thrombosis,119-121 meningoencephalitis,122 and disseminated intravascular coagulation.123,124
MANAGEMENT OF DIABETIC KETOACIDOSIS
The International Society for Pediatric and Adolescent Diabetes has recently completed consensus treatment guidelines.81 Children with established diabetes, who are not vomiting or severely ill and whose caregiver has been trained in sick day management, can be managed at home or an outpatient facility with appropriate supervision and follow-up.155-158 Children requiring intravenous rehydration over an extended period need to be admitted to a unit in which neurologic status and vital signs can be monitored frequently and blood glucose levels measured hourly.81,158 Nursing staff trained in monitoring and management of diabetic ketoacidosis (DKA) should be available, and there should be written treatment guidelines.81,158 Those with severe DKA (long duration of symptoms, compromised circulation, depressed level of consciousness) or with other factors increasing the risk for cerebral edema (ie, age under 5 years, low Pco2, high serum urea nitrogen) should be in an intensive care unit, preferably pediatric, or an equivalent facility.81,158
Evaluation
Differential diagnosis should include other causes of coma in children that might be present instead of or in addition to DKA (see Chapter 111). A body weight should be obtained for calculation purposes. The degree of dehydration can be estimated as 5% with reduced skin elasticity, dry mucous membranes, tachycardia, and deep breathing and up to 10% with capillary refill time greater than 3 seconds and sunken eyes. It is typical for calculations of fluid deficit to be based on 10% dehydration, which is a modest overestimate that does not appear to have clinical significance.159 The level of consciousness should be assessed using the Glasgow coma scale (Table 104-1).160 An initial blood sample should be tested for glucose, electrolytes, pH, urea nitrogen, creatinine, osmolality, ketones or β-hydroxybutyrate, hemoglobin and hematocrit or complete blood count, keeping in mind that diabetic ketoacidosis is associated with leukocytosis that does not correlate with the presence or absence of infection. As a substitute for measured osmolality, calculated osmolality may be determined as: 2×[Na + K] + glucose in millimoles per liter (or glucose in milligrams per deciliter divided by 18). A urine specimen should be checked for ketones. Appropriate culture specimens should be obtained as indicated. If there is any possibility of delay in obtaining a serum potassium result, electrocardiographic monitoring should be done for potassium status.161,162 Continuous electrocardiographic monitoring using standard lead II should be done for severely ill patients or if the initial potassium level is less than or equal to 3 mEq/L or more than or equal to 6 mEq/L; depressed T waves indicate hypokalemia, and peaked T waves hyperkalemia.163
Determination of glycohemoglobin (HbA1c) concentration will provide insight into the severity and duration of hyperglycemia in new patients and is useful for comparison to the previous determination in an established patient. It may also be useful to obtain a blood sample for measurement of free insulin to confirm an impression of failure to administer insulin as the cause of the diabetic ketoacidosis before administering any insulin.164
The severely obtunded child will require continuous nasogastric suction for the prevention of pulmonary aspiration and may require oxygen. Bladder catheterization should be performed only in individuals who are unable to void on demand; older patients may have atonic bladder with urinary retention requiring initial catheterization, but not indwelling catheterization.
Successful management and early intervention for complications require close monitoring. A flow chart should be maintained with as frequent entries as indicated by the patient’s condition (Table 544-6).165 Monitoring frequency recommendations are in Table 544-7.165
Fluid and Insulin Replacement
The goals of treatment with fluid and insulin are to (1) restore perfusion, which will increase glucose use in the periphery, restore glomerular filtration, and reverse the progressive acidosis; (2) arrest ketogenesis with insulin administration, reversing proteolysis and lipolysis while stimulating glucose uptake and processing, thereby normalizing blood glucose concentration; (3) replace electrolyte losses; and (4) avoid, insofar as possible, the complications of treatment or to intervene rapidly when they occur.
Fluid Therapy
• Dehydration can be assumed to be 5% to 10% (50–100 mL/kg). As noted above, clinical evaluation usually overestimates the level of dehydration. Serum urea nitrogen and hematocrit may be useful for determining severity of extracellular fluid (ECF) contraction. Serum sodium concentration is not reliable for determining ECF deficit because of the osmotic effect of hyperglycemia-induced dilutional hyponatremia166,167 and the low-sodium content of the elevated lipid fraction of the serum in diabetic ketoacidosis. Corrected sodium, that is, for normal glucose level, can be estimated by adding 1.6 mEq to the measured value for each 100 mg/dL blood glucose above normal.166
• NaCl (0.9%) at 20 mL/kg should be provided in the first 1 to 2 hours to restore peripheral perfusion.
• Maintenance can be calculated as 1000 mL for the first 10 kg body weight + 500 mL for the next 10 kg + 20 mL/kg over 20 kg.
Table 544-6. Diabetic Ketoacidosis/Hyperglycemic Hyperosmolar State Flow Sheet
• The remainder of replacement after the loading dose based on 5% to 10% dehydration, and maintenance, can be distributed over the subsequent 22 to 23 hours. Some prefer to provide half of replacement in the first 8 hours. It is important to consider fluids that have recently been administered orally at home (if not vomited) and parenteral fluids provided in the emergency room or referring institution.
• If osmolality (calculated or measured) is greater than 320 mosm/L, replacement can be calculated for correction in 36 hours, and if greater than 340 mosm/L, in 48 hours. Except for severely ill and very young individuals, oral intake will begin before 24 hours.
• While urinary output should be carefully documented, urinary losses should not be added to fluid requirements.81
• After initial 0.9% NaCl bolus, rehydration/maintenance should be continued with 0.45% NaCl. During rehydration, the measured Na can increase to the level of the corrected Na as glycemia declines and then decline to normal levels if the corrected level was elevated.
• The use of large amounts of 0.9% saline may result in hyperchloremic metabolic acidosis.168,169
• To prevent unduly rapid decline in plasma glucose concentration and hypoglycemia, 5% glucose should be added to the IV fluid when the plasma glucose falls to 300 mg/dL (17 mmol/L). An efficient method of providing glucose as needed without long delays entailed by the changing of IV solutions is to have 2 IV fluid bags connected, 1 containing 10% dextrose and the appropriate sodium and potassium concentrations and the other with the same salt concentrations but no dextrose, the so-called 2-bag system.170
• Potassium (20–40 mEq/L or up to 80 mEq/L as needed) can be provided as half KCl, half KPO4 (to replenish low phosphate levels and to decrease the risk of hyperchloremia) or as half KPO4 and half K acetate (which, like lactate, is converted to bicarbonate to help correct acidosis) after serum K is reported as less than 6 mmol/L or urine flow is established. Serum potassium concentration increases by approximately 0.6 mEq/L for every 0.1 decrease in pH, so that the serum potassium level does not reflect the large deficit from diuresis and vomiting. Both potassium and phosphate shift markedly from intracellular to extracellular compartments with acidosis and reenter cells rapidly with insulin-induced glucose uptake and rehydration.163,171
• Bicarbonate is very rarely indicated. There is no evidence that bicarbonate facilitates metabolic recovery. Restoring circulatory volume will improve tissue perfusion and renal function, increasing organic acid excretion and reversing lactic acidosis. The administration of insulin stops further synthesis of ketoacids and reactivates the Krebs cycle, permitting their metabolism and regeneration of bicarbonate. Bicarbonate therapy may cause paradoxical central nervous system (CNS) acidosis: Rapid correction of acidosis can cause hypokalemia, the additional sodium can add to hyperosmolality, and alkali therapy can increase hepatic ketone production, potentially slowing recovery from ketosis.81 The extremely rare circumstance that may benefit from cautious alkali therapy is severe acidemia, in which tachypneic response is inadequate, or persistent compromised perfusion following initial fluid expansion, which suggests a decreased cardiac contractility and peripheral vasodilatation.81 To avoid the risk of hypokalemia, safe administration is 1 to 2 mEq/kg body weight or 80 mEq/m2 body surface area over 2 hours. The NaCl concentration in the fluids should be reduced to allow for added Na ion.
Table 544-7. Monitoring Treatment of Diabetic Ketoacidosis or Hyperglycemic Hyperosmolar State in Children
Insulin
• Insulin should be started after the initial fluid expansion is completed for a more realistic starting glucose level.
• The most widely used system is 0.1 U/kg hourly as a continuous infusion, using a pump. Use 50 U of regular insulin diluted in 50 mL normal saline to provide 1 U/mL.
• A bolus dose is not necessary and may increase the risk of cerebral edema.172
• It may be more convenient in some settings to administer insulin subcutaneously. In adults, there did not seem to be any difference whether insulin was administered intravenously, intramuscularly, or subcutaneously after the first couple of hours of treatment.173-176 The first study of subcutaneous insulin in children with diabetic ketoacidosis using rapid-acting insulin analog (lispro) provided a dose of 0.15 U/kg every 2 hours, finding no essential differences from children randomized to receive 0.1 U/kg per hour intravenously.177 The administration of 0.1 U/kg subcutaneously every hour may be preferable and can be adjusted to maintain blood glucose concentration at approximately 250 mg/dL (11 mmol/L).
• During initial fluid expansion, a high blood glucose level may drop 10 to 15 mM/L, even without insulin infusion. High blood glucose levels should drop 50 to 150 mg/dL/hour (but not > 200 mg/dL/hour), and if they do not, the dose of insulin should be increased. This is rarely necessary.
• If the blood glucose level falls below 150 mg/dL with 10% dextrose solution running, the insulin dose should be reduced to 0.05 U/kg/hour.
• Insulin should not be stopped or reduced below 0.05 U/kg/hour, for a continuous supply of insulin is needed to prevent ketosis and permit continued anabolism.
• Persistent acidosis, defined as bicarbonate value less than 10 mmol/L after 8 to 10 hours of treatment, is usually caused by inadequate insulin effect, indicated by persistent hyperglycemia. Insulin dilution and rate of administration should be checked and a fresh preparation made if it is older than 6 to 8 hours. Too dilute a solution may enhance absorption to the tubing. If insulin is being given by subcutaneous injection, inadequate absorption may be occurring or there may be resistance due to unusually high counterregulatory hormones, as with concomitant febrile illness.165 Extremely rare causes of persistent acidosis are lactic acidosis due to an episode of hypotension or apnea or inadequate renal competency in the handling of hydrogen ion as a result of an episode of renal hypoperfusion.165
Transition
• IV fluids can be stopped l to 2 hours after substantial consumption of oral fluids without vomiting.
• Subcutaneous insulin injection can be started when the patient no longer needs IV fluids. It is most convenient to wait until the presupper or prebreakfast time to restart intermediate- or long-acting insulin. Until then, regular insulin 0.25 U/kg subcutaneously can be given approximately every 6 hours and the insulin infusion stopped 60 to 120 minutes after the first subcutaneous dose of regular insulin or 15 to 30 minutes after rapid-acting insulin analog.
• Patients should not be kept in the hospital simply to adjust insulin dosage, because food, activity, and psychosocial environment are not normal.
Prevention
The prevention of diabetic ketoacidosis (DKA) in children and adolescents requires a diligent health care system. The prevention of DKA at onset is possible when early diagnosis is made through genetic and immunologic screening of high-risk children.89,97 For the larger population, an example has been provided by the Italian school and physician awareness program directed at 6 to 14 year olds, which reduced the rates of DKA from 78% to near 0% over 6 years.92 Recurrent DKA was demonstrated to be dramatically reduced 30 years ago by a comprehensive program involving outreach clinics, frequent routine and emergency telephone contact, and a camping program supported by a state program for children with special health care needs. Patients with compliance problems resulting in recurrent DKA account for a disproportionate number of recurrent DKA episodes. While psychosocial intervention is of importance, the necessity for assuring administration of insulin by responsible adults is paramount.
CHRONIC MANAGEMENT OF TYPE 1 DIABETES
Type 1 diabetes (T1D) management poses a daunting challenge to children, families, and health professionals. Not only must the condition be constantly considered in every activity or plan a person makes, but numerous less-than-pleasant tasks must be performed daily, and unexplainable variations occur nonetheless. If complications ensue, after years of being told that good control will prevent them, the person with T1D must deal with guilt and self-recrimination. The fundamental problems in controlling glycemia remain, although services, tools, and insulin have improved the ability to achieve control. The basic problem is the inability to deliver insulin in the physiologic manner, that is, into the portal vein in immediate response to food intake, and turn it off as rapidly. Newer forms of insulin and delivery systems (slow- and fast-acting analogs, pens, pumps) have made the approximation better, but with the requirement that more attention be paid to the diabetes. The Diabetes Control and Complications Trial demonstrated that the general level of control reached in this circumstance was inadequate to forestall microvascular complications, and the ability of patients to monitor their own blood glucose and physicians to estimate average control was by then standard of care.183
The final and perhaps most vexing limitation is the inability to control human behavior and social circumstances, which have a profound effect on compliance and metabolism. Nonetheless, progress has been made with this limitation, as well. The patient and family are recognized as being the major players on the treatment team, entitled to extensive diabetes and nutrition education, 24-hour access to expert assistance, psychological support as needed (enhanced by extensive psychosocial research in this area), and special programs such as camping.184-186 In sum, contemporary management of T1D is far more demanding of children, their families, and the health care system than before the modern technology era, but infinitely more satisfying for all members of the team.
THE FIVE ES
The five Es of diabetes control are education, emotions, eating, exercise, and enthusiasm/engagement/earnestness. Knowledge is power, but only when the knowledge is used. Each of the factors influences every other. For example, a child who is engaged in sports or otherwise exercising regularly, is likely to be eating better, feel better about herself, be less anxious, and be more enthusiastic about taking care of her diabetes.
STARTING TREATMENT
Learning basic diabetes physiology and management can give the family a sense of control and confidence to cope with the anxiety and despair that accompanies the diagnosis. This is preferably carried out in the outpatient setting, which is much more comfortable for education than is the emotionally highly charged hospital environment, and outpatient management greatly reduces costs.98 Additionally, often the family obtains and reports glucose measurements and immediately follows through on recommendations conveyed over the telephone than occurs in hospital settings. Nevertheless, some children who are not in diabetic ketoacidosis may need to be admitted to the hospital because outpatient management is not available or the social situation is problematic. In such situations, referral to a pediatric diabetes center should be the strategy of choice.
The initial education efforts should be concise and simple, addressing only the most critical needs of checking blood sugar levels, mixing insulin, giving insulin injections, identifying and treating hypoglycemia, and testing for and treating ketonuria. This effort must be accompanied by reassurance that any questions that arise are appropriate for calling in. Over subsequent visits, more information about diabetes and advanced management techniques and goals for glycemic control are progressively discussed.
Treatment usually begins with a combination of neutral protamine Hagedorn insulin and a rapid-acting analog such as aspart or lispro. Most children will require a total insulin dose of 1 U/kg/day. Adolescents are less insulin sensitive and may require 1 to 1.5 U/kg/day. The daily insulin dosage is typically divided into two thirds of the total in the morning and one third in the evening. The beginning morning dose is two thirds neutral protamine Hagedorn insulin and one third rapid-acting analog and the presupper dose one half neutral protamine Hagedorn insulin and one half rapid-acting analog.
The long-acting nonpeak analogs (glargine, determir) are seldom used at the time of diagnosis, except in families with other members having type 1 diabetes. Newly diagnosed children and their families need time to adjust to the treatment, monitoring, and nutritional demands of the basal-bolus regimen and to using twice-daily insulin before being taught to keep count of carbohydrate intake and take 3 or 4 injections daily. Nevertheless, pump administration of insulin and glargine-based regimens are generally superior to neutral protamine Hagedorn use.
MULTIPLE DAILY INJECTION AND CONTINUOUS SUBCUTANEOUS INSULIN INJECTION
Multiple daily injection (MDI) using glargine and mealtime rapid acting insulin analogs or continuous subcutaneous insulin injection (CSII) using insulin pumps are offered to children who have already demonstrated the ability to manage their diabetes somewhat independently and to younger children who have parents who are committed to their care. Several studies have demonstrated the ability of CSII and MDI to improve metabolic control and reduce hypoglycemia frequency in young children and toddlers. Both of these approaches require more frequent blood glucose testing and insulin administration than do fixed doses given 2 to 3 times per day, but many children and parents accept the increased work required because of the lifestyle flexibility and improved control. All regimens will fail unless the child is committed to performing the diabetes tasks necessary to succeed. Thus, before changing therapy, children interested in MDI or CSII should attend a carbohydrate-counting or pump class, demonstrate that they will monitor their blood glucose levels 4 times per day, call weekly with blood glucose results, and record their carbohydrate intake and “virtual” rapid-acting insulin boluses based on a prescribed insulin-to-carbohydrate ratio and correction factor.
Initial glargine doses are calculated as 70% of the total intermediate-acting insulin previously given. Similarly, initial pump rates are usually 70% to 80% of the previous total daily amount of subcutaneous insulin, with 60% of that given as a basal dose divided over 24 hours. Meal coverage is determined by using insulin (units)-to-carbohydrate (grams) ratios. Ratios in children may vary from 1:30 for young children to 1:5 for adolescents at the peak of the physiologic insulin resistance of puberty.
BLOOD GLUCOSE MONITORING
Blood glucose levels should be monitored at least 4 times per day, before breakfast, lunch, and dinner, and at bedtime. Intensive insulin therapy (multiple daily injections, insulin pump) also requires checking before snacks so that appropriate doses of rapid-acting insulin can be taken. More frequent monitoring throughout the day and during the night may also be needed when starting pump use or at times of other major management changes. In order to determine if the rapid-acting insulin dose is correct, 2-hour postprandial blood glucose levels are encouraged to assess the effectiveness of the insulin-to-carbohydrate ratio. If a child is waking up with high blood glucose levels, the caregiver needs to obtain a 2 or 3 am blood glucose level before adjusting the evening insulin dose upward to be sure that the child is not becoming hypoglycemic in the middle of the night and having rebound hyperglycemia in the morning: In this case the dose would need to be reduced instead of increased. It is also necessary to check the blood glucose levels more frequently during illness.
Patients and caregivers are encouraged to keep a logbook with blood glucose levels, insulin doses, and notes detailing events that may affect blood glucose readings, and to bring both the logbook and the meter for download to the clinic visit. Unless a daily or weekly record is kept, it is unlikely that patients and caregivers will recognize developing blood glucose patterns.
BLOOD GLUCOSE AND HBA1C GOALS
HbA1c is the hemoglobin fraction that is nonenzymatically glycated and therefore reflective of average blood glucose over the average half-life of the red cell hemoglobin, that is, 60 days. HbA1c goals must be age adjusted in children and adolescents to minimize the danger of serious hypoglycemia; fortunately, the risk for long-term complications is much less related to duration of diabetes before 15 years of age than after.187 Children who have recurrent hypoglycemia at an early age (< 5 years old) may be at increased risk for long-term neuropsychological impairment.188,189 Glycemic goals for infants and toddlers are 100 to 180 mg/dL (5.6–10 mmol/L) before meals, 110 to 200 mg/dL (6–11 mmol/L) overnight, and the HbA1c goal between 7.5% and 8.5%. School-aged children (6–12 years) should achieve better control, with HbA1c less than or equal to 8%.
In adolescents, the problems of adolescent rebellion and independence require a more individualized approach. Premeal blood glucose levels should be 90 to 130 mg/dL (5–7.2 mmol/L), and the goal should be to maintain a HbA1c value of less than 7%. This goal is difficult for most teens to achieve, however, and the ADA has recommended a HbA1c goal of 7.5% for this age group, with an ideal HbA1c value of less than or equal to 7% if achievable without hypoglycemia.
NUTRITION
Children with diabetes should be encouraged to maintain a normal, healthy, age-appropriate diet, which includes avoiding concentrated sweets and excess calories. Daily caloric requirements for children can be estimated by using the formula 1000 kcal plus 100 kcal per year of age. Most children should obtain their caloric intake from a balanced diet containing 50% to 60% carbohydrates, 30% fat, and 10% to 20% protein. Fat should be limited to less than 30% of caloric intake if the child is overweight, with saturated fat less than 10% of daily calories and less than 7% of calories if the child has elevated low-density lipoprotein (LDL) cholesterol. Finally, cholesterol intake should be limited to 300 mg/day; if the child has elevated LDL cholesterol, this should be less than 200 mg/day.
Carbohydrate counting is important for children using multiple daily injection or continuous subcutaneous insulin injection. They may require simple carbohydrate ratios to minimize confusion, and many children require postprandial glucose monitoring to determine carbohydrate ratios for particular meals. Food should never be used to reward or punish children, especially those with diabetes.
AGE-SPECIFIC MANAGEMENT ISSUES
Infants
Caring for an infant with diabetes can induce considerable emotional distress. It is difficult to traumatize infants and young children with multiple blood glucose level checks and insulin injections because the parent is unable to explain the reasons for the finger sticks and injections. Fortunately, infants adapt quickly to glucose monitoring and insulin administration. In addition to the anxiety of having an infant with diabetes, parents also have to cope with unpredictable eating and play activity level. They may need to give insulin after meals, based on intake, and this is now practical with the rapid-acting analogs.
Toddlers
Toddlers are able to scream, kick, bite, or make hurtful statements to caretakers who are giving insulin or checking blood glucose levels. As are infants, they remain incapable of understanding the need for these procedures. Thus, the parent or caregiver must carry out diabetes care tasks in a matter-of-fact, nonemotional way and provide enthusiastic praise when the toddler is cooperative. The combination of positive reinforcement and a matter-of-fact delivery of diabetes care will teach children that their cooperation is rewarded. Consistency and firmness are critical in teaching the child that diabetes care needs are non-negotiable and are not subject to argument.
Elementary School Age Children
In the elementary school-age child, school absenteeism as the result of manipulative use of diabetes-related complaints can become a serious problem. Parents often worry about sending their child with diabetes to school when the child may actually be ill. Children should not be allowed to miss school unless there is good evidence of an illness, such as fever or vomiting.
As diabetes care has become more intensive, with blood glucose monitoring and insulin administration required during the school day, it is essential that school personnel be trained to handle diabetes-related tasks. Many schools do not have a nurse on site at all times, and educators and other school personnel often do not feel comfortable or competent dealing with the medical needs of the child. Nevertheless, parents and physicians must advocate and help educate school officials, and in cases in which a nurse is not available, teachers or coaches can be taught to provide basic diabetes care. Older children—those in middle and high schools—are usually developmentally able to check their blood glucose levels and administer insulin boluses independently. Diabetes should not keep children from participating in any school activities, including after-school sports. Conversely, school should not be a barrier for children’s ability to care for their diabetes.
Children with diabetes are covered under Section 504 of the Rehabilitation Act of 1973, the Individuals with Disabilities Education Act of 1991, and the Americans with Disabilities Act. Public schools, day care centers, and all schools that receive federal funding fall under the jurisdiction of these acts,190 requiring that the institutions provide adequate accommodation for children with diabetes. Parochial schools are exempt. (A sample 504 plan and an excellent book about diabetes in the schools are available on the National Diabetes Education Program (http://www.ndep.nih.gov/resources/resources.htm) and American Diabetes Association (http://www.diabetes.org/uedocuments/ad-504-adanasndredf-2007.pdf. and http://www.diabetes.org/advocacy-and-legalresources/discrimination/school/schooltraining.jsp) web sites.
The increasing number of children using continuous subcutaneous insulin injection and multiple daily injection requires that school nurses or the diabetes liaison oversee lunchtime insulin injections. Parents and physicians must be sure that nurses or diabetes liaisons are aware of the child’s specific blood glucose goals and are appropriately trained to either administer or oversee insulin injections. Physical fitness is important for children with diabetes to maintain cardiovascular health and improve metabolic control. Ready access to fluids and carbohydrates is essential. If physical education is expected to last more than 30 minutes, a 10% to 20% reduction in the insulin dose or a juice box or small snack can be used to avoid hypoglycemia. Glucose testing should be made available at the site and should be allowed any time the child feels symptoms of hypoglycemia. The symptoms of hypoglycemia are clinically difficult to distinguish from those of stressful exercise, so it is better to err on the side of unnecessarily treating the symptoms as hypoglycemia, with carbohydrate ingestion, than run the risk of worsening hypoglycemia if glucose measurement cannot be immediately done. Full participation in physical education should be expected. Creating an exercise program for children with diabetes that provides for appropriate monitoring and the expectation that they participate fully teaches children that diabetes should not exclude them from any activities.
School-aged children begin to understand diabetes care and may want more independence in managing their diabetes. Although they may handle diabetes management tasks well, they still require supervision by an adults.
Adolescence
Poor adherence is a major problem for adolescents, who are usually managing their own care, during a period of development characterized by rebellion, rejection, and denial of vulnerability. Not all adolescents are ready for the responsibility of self-care, however, and all require some degree of supervision. Risk-taking behaviors such as smoking, alcohol and other substance use, sexual activity, and careless driving need to be addressed. Menstrual irregularities may be a sign of poor glycemic control. Discussion of contraception and pregnancy risks should be included in every adolescent office visit. Oral contraceptives have clinically insignificant effects on glucose tolerance, so that there is no reason to avoid their use in girls with type 1 diabetes.
The characteristic stresses of adolescence associated with social pressures, schoolwork, and puberty add an uncontrollable variable to the management of diabetes. The physiologic insulin resistance of adolescence, likely the result of increased growth hormone secretion, may be further intensified by elevations of cortisol, catecholamines, and sex steroids, resulting in increased insulin requirements (up to 1.5 to 2 U/kg/day) and extended periods of hyperglycemia and elevated HbA1c values during periods of stress and menses. Some pediatric endocrinologists use metformin as an adjunct therapy in adolescents with type 1 diabetes to enhance insulin sensitivity.
SICK DAY MANAGEMENT
Sick day management, designed to prevent or treat early ketoacidosis (diabetic ketoacidosis) during illness or stress, requires repeated reinforcement with caregivers and adolescents. Provision of written instructions is important because of the infrequency of the situation. A common mistake is to skip insulin injection if the child refuses to eat or vomiting is present. The notion of continuing to take insulin despite no food intake is counterintuitive for many parents; in fact, the insulin requirements are typically increased because of the stress of illness, resulting in counterregulatory hormone-induced gluconeogenesis and keto-genesis. Blood glucose levels should be checked more frequently than usual, at least every 2 to 4 hours. Urine ketones should be checked often, as well. If the child is sick, ketones should be monitored even if the blood glucose level is low or normal. If vomiting occurs, ketones should be checked each time the child urinates, with repeated testing at least every 2 to 3 hours. Extra insulin may be necessary for the insulin resistance accompanying the illness or if ketones are present.
For negative, trace, or small ketonuria, increasing water intake, exercise, or insulin supplementation can be tried. For moderate or large ketonuria, extra insulin should be administered based on the total daily dose of insulin.
For children using multiple daily injection, temporary adjustments of the insulin/carbohydrate ratio, target blood glucose levels, and doses of the long-acting and/or rapid-acting insulins may be necessary.
Those using continuous subcutaneous insulin injection can program a temporary basal rate, usually a 20% to 30% increase for 3 to 4 hours.
While most children become hyperglycemic with illness despite not eating, some will have a decrease in glycemia under these conditions. We provide the following guide to our patients for appropriate fluids for various blood glucose ranges.
• If the blood sugar is below 120 mg/dL:
• Fluids need to contain extra glucose, such as soda or a sport drink with sugar added. The child should continue with glucose-containing drinks until the blood sugar is above 120 mg/dL.
• If the blood sugar is between 120 and 180 mg/dL:
• Fluids need to contain approximately 15 g of carbohydrates, such as one half cup of ginger-ale or cola, one half cup of regular Jell-O®, one half cup of juice, or one half of a popsicle.
• Foods need to have at least 15 g of carbohydrates per serving. If the child cannot eat normal meals, possible “sick day” foods include: one half cup of ice cream or sherbet, 1 slice of toast, or 6 saltines.
• If blood sugars are above 180 mg/dL:
• Fluids need to have no calories, such as diet soda, unsweetened tea or water.
Foods need to be small servings as tolerated. If the child is nauseated or vomiting, give small sips of clear fluids (such as ice chips, popsicles, clear broth or bouillon, gelatin, water, or tea). Avoid orange juice, milk, or milk products. Speak with your diabetes team about fluid replacement and advancing to foods. Nutritional needs and insulin doses can change hour by hour depending on blood glucose and urine ketone levels.
An over-the-counter product to control nausea, such as Emetrol, may prevent vomiting. Regular cola, ginger ale, or other soda with the bubbles shaken out may also be helpful. Vomiting is the signal that the diabetes team or primary physician needs to be called. If the child has not been ill for more than a few hours and has not been vomiting for more than couple of hours, a Tigan or Phenergan suppository may control the vomiting and permit oral fluid intake, thus avoiding admission to the emergency room or hospital for rehydration.
DIABETES CAMP
Diabetes camps provide a unique opportunity to educate and socialize children with diabetes. Children who are initially reluctant to attend a diabetes camp often leave camp with a new sense of acceptance of their diabetes and renewed interest in maintaining near-normal blood glucose control. Most diabetes camps are residential camps staffed by experienced pediatric diabetes nurses, psychologists, and physicians. Strict observation of blood glucose monitoring and insulin administration is required to assure maximum vigor for camp activities and prevent ketosis/ketoacidosis.191 Daily insulin adjustments must be made, as well, for most children are much more active at diabetes camp than at home, increasing the risk for hypoglycemia. Local Juvenile Diabetes Research Foundation and American Diabetes Association contacts typically have the best information on diabetes camps in the area.
LONG-TERM COMPLICATIONS
The Diabetes Care and Complications Trial and the longitudinal follow-up of these patients, the Epidemiology of Diabetes Interventions and Complications study, demonstrated that tight glycemic control is critical in reducing both microvascular and macrovascular complications of diabetes.183,192 The ability to normalize blood glucose is now limited only by the frequency and severity of hypoglycemia.193 Microvascular disease results in retinopathy, nephropathy, and neuropathy. Macrovascular complications affecting coronary, carotid, and other major vessels are now recognized as having their origins in childhood. Of particular interest in children are the effects of diabetes on growth and development and associated connective tissue complications.
Joint Problems
Limited joint mobility (LJM) is the earliest clinically apparent long-term complication of type 1 diabetes (T1D) in childhood and, following its initial description in 1974, was recognized to be a common feature of pediatric T1D.180 The initial description was of bilateral, painless, but obvious, contracture of the finger joints and large joints, with thick tight waxy skin and short stature in 3 older teenagers with long-standing diabetes and early microvascular complications.194 Milder expression was subsequently recognized as affecting approximately 30% of youngsters with diabetes, a third of whom had involvement of more than 2 fingers or 1 finger and 1 large joint bilaterally.180
LJM is painless and only mildly disabling when severe (eFig. 544.2 ). A simple examination method is to have the patient attempt to approximate palmar surfaces of the inter-phalangeal joints. Passive examination is essential to confirm that inability to do so is due to LJM. Attained age seems more important than duration of diabetes or age of onset of diabetes in the evolution of LJM. With rare exception, LJM appears after the age of 10 years. The biochemical basis for LJM is likely glycation of protein with the formation of advanced glycation end products. This results in increased stiffness of the periarticular and skin collagen, with decreased range of motion.
As might be expected, the presence of LJM is associated with increased risk for the development of microvascular disease. Attempts to correlate the development of LJM with diabetes control as measured by HbA1c levels were unsuccessful in cross-sectional studies, but a longitudinal study of average HbA1c from the first year after diagnosis demonstrated unequivocally that the appearance of this complication was significantly related to diabetes control.197
Both the prevalence and severity of LJM have decreased since the initial recognition in the 1970s, most likely the result of improved glucose control during this era.198 These study results are pertinent to the question of the importance of prepubertal duration and control of diabetes for the development of long-term microvascular complications.
Skin Problems
Necrobiosis lipoidica diabeticorum is a rare pretibial lesion that is characterized by round or oval indurated plaques, with central atrophy and eventual ulceration. Unless they become infected, the lesions are painless. Relationship to diabetes control is uncertain, but there is an association with smoking, proteinuria, and retinopathy.199,200 The lesions do not heal well and need to be protected from trauma and treated vigorously if infected.
Lipoatrophy, indentation, or atrophy of the subcutaneous tissue resulting from insulin injection has become uncommon with the generalized use of recombinant derived human insulin. It is presumed that the impurities in animal extract insulin or the foreign nature of that insulin led to the problem. When lipoatrophy develops, giving insulin injections around the edges of atrophic areas or using an alternative insulin analog may be helpful.204
Lipohypertrophy, in contrast to lipoatrophy, always occurs at the site of injection as a local reaction to repeated injection trauma, usually reflecting favorite site injection, with scarring and decreased sensitivity to pain.205 Unlike lipoatrophy, lipohypertrophy continues to be a frequent problem with improved insulin forms, affecting as many as half of patients under 20 years of age.206 Varying absorption of insulin from areas of hypertrophy can result in erratic glycemic control.206 Site rotation is also important in reducing the risk of developing lipohypertrophy. Once lipohypertrophy develops, injections into the area of induration should be discouraged. If injection of the affected area is avoided, most lipohypertrophy will resolve within 6 to 8 weeks. However, some areas of lipohypertrophy become permanent, even with dedicated avoidance of injections to the area.
Cataracts
Cataracts occur rarely during recovery from ketoacidosis, typically in the newly diagnosed patient; they usually disappear rapidly but may persist, requiring surgical removal.207 In contrast to these “sugar” cataracts, subcapsular juvenile cataracts, associated with chronic hyperglycemia and caused by sorbitol accumulation, do not regress and always require removal.
Growth Failure and Delayed Sexual Maturation
Insulin sufficiency is necessary for normal growth and development. During the nearly 6 decades between the discovery of insulin and the contemporary era of diabetes management, growth retardation and delayed puberty were frequently seen in children with diabetes. In twin pairs discordant for diabetes, the development of diabetes before the onset of puberty was associated with invariably shorter stature than that of the nonaffected twin.208 Nonetheless, despite the generally nonphysio-logic traditional means of treating diabetes, most children with diabetes did not have serious growth or maturational delay problems. Most children with diabetes now experience normal timing of puberty, with normal growth and final adult heights.209,210
The effects of insulin on normal growth and development are largely mediated through the anabolic pathway and the GH-IGF-1 axis. Deficiency of portal insulin delivery to the liver results in reduced circulating growth hormone (GH) binding protein (the extracellular domain of the cell surface receptor for GH), GH resistance, and diminished IGF-1 production.211,212 The deficiency in insulin also results in decreased production of IGFBP-3, the principal binding protein for circulating IGF-1, and increased production of IGFBP-1 and IGFBP-2.213 These latter binding proteins, unlike IGFBP-3, do not deliver their bound IGF-1 to tissues. Furthermore, increased proteolysis of IGFBP-3 decreases its viability in the circulation. In addition to the GH resistance, which is reflected in the lower concentrations of circulating GH binding protein, there may be postreceptor defects in GH action mediated by the insulin deficiency. Diminished circulating total and free IGF-1 levels are associated with GH hypersecretion because of the absence of negative feedback from IGF-1. This hypersomatotropism increases glycemia and decreases insulin sensitivity, but is reversible with adequate insulin replacement.214
The Mauriac syndrome consists of growth failure, maturational delay with hepatomegaly, and abdominal distention in insulin-treated children.215 There appear to be 2 forms of the Mauriac syndrome. In 1 form there is associated Cushingoid obesity and documented wide fluctuation between hyperglycemia and hypoglycemia, suggestive of a pattern of over- and underinsulinization, which would be expected with treatment using only soluble insulin, with secondary hyperadrenalism.217 More recent reports are of patients with Mauriac syndrome who do not have obesity and who are without a history of alternating hypoglycemia and ketoacidosis; these patients are unmistakably inadequately insulinized continuously.218,219
Eating disorders are also more common in children with diabetes than in the general population and should be considered in the assessment of any child who is not gaining weight or growing normally.220-223 Adolescent females with diabetes are twice as likely to have eating disorders than are those without diabetes. Mean HbA1c levels were higher in adolescents with diabetes who had an eating disorder than in those who did not.224
Autoimmune Disorders
Associated autoimmune problems, such as hypothyroidism and celiac disease, can result in growth failure in children with type 1 diabetes (T1D). Approximately 20% of children with T1D have antibodies indicative of autoimmune thyroid disease.220 Anti-thyroid autoantibodies should be tested for in all youngsters with T1D at diagnosis, and those who are positive should have annual determination of TSH levels. It has recently been recommended that all children with T1D have TSH testing every 1 to 2 years and any time thyromegaly is noted or clinical thyroid dysfunction is suspected.221 If TSH is abnormal, free T4 should be measured.
Because celiac disease has been described in 5% to 8% of children with T1D, tissue transglutaminase antibodies should be sought in children with growth failure without other obvious cause, even in the absence of diarrhea.221 Children should be tested soon after T1D diagnosis for celiac disease, using tissue transglutaminase antibodies and serum IgA levels. Retesting should be done if growth failure, failure to gain weight, weight loss, or gastrointestinal symptoms occur. Small bowel biopsy is required to confirm the diagnosis. Management is with a gluten-free diet.222
MICROVASCULAR COMPLICATIONS
Thickening of the capillary basement membrane with alterations in membrane permeability and occlusion of small blood vessels is the hallmark of diabetic microvascular disease. Although some of the long-term complications of T1D discussed above are likely partially due to microvascular disease, renal disease and retinopathy are more clearly linked. Several mechanisms have been proposed to explain the pathophysiology of these complications, all with the basic concept of hyperglycemia-induced tissue damage that appears to result from the increased production of reactive oxygen species.225
1. Glycemic control is directly related to the development and progression of microvascular disease.183 This suggests that excess glucose per se has deleterious effects on tissues. Some of these effects appear to be through binding to collagen by posttranslational nonenzymatic means, depending directly on glucose concentration.
2. Sorbitol is the alcohol of glucose that is formed from glucose by aldose reductase, the magnitude of the reaction driven by the concentration of blood sugar leading to sorbitol accumulation in a number of tissues, including lens and nerve, which leads to increased permeability and increased pressure and leakage in blood vessels and decreased vessel wall elasticity and microvascular hypertension.226
3. Intracellular hyperglycemia also increases the synthesis of diacylglycerol, a key activator of protein kinase C, activation of which has multiple effects on gene expression that result in inflammation, increased reactive oxygen species, and vascular dysfunction.225
4. As described above, diabetes is associated with increased fibrinogen levels and elevations in other antithrombotic factors that may result in increased platelet stickiness, resulting in increased red blood cell aggregation and blockage of small capillaries.227
Nephropathy
Microalbuminuria, defined as urinary albumin excretion rates of 20 to 200 μg/minute or 30 to 300 mg/24 hours, is predictive of renal and cardiovascular disease.228 Higher concentrations are considered to reflect overt diabetic nephropathy. HbA1c level, LDL cholesterol concentration, and body mass index were all risk factors for the development of microalbuminuria in 12.6% of 1,134 men and women with type 1 diabetes aged 15 to 60 years during the 7-year follow-up in the EURODIAB Prospective Complications Study.229
A study of the prevalence and progression of microalbuminuria and clinical proteinuria in 361 children with type 1 diabetes over the course of 12 years found a very low incidence of microalbuminuria. Single episodes of microalbuminuria increased between the ages of 10 and 18 years (30.9% of males and 40.4% of females), but persistent microalbuminuria and progression from microalbuminuria to macroalbuminuria was rare.230 Predictors of persistent albuminuria have included elevated HbA1c and high baseline albumin excretion rates.
The Pittsburgh Epidemiology of Diabetes Complications study is an observational prospective study of 589 patients with onset of T1D before the age of 17 years. Baseline LDL levels greater than or equal to130 mg/dL (3.36 mmol/L), triglycerides greater than or equal to 150 mg/dL (1.7 mmol/L), systolic blood pressure greater than or equal to 130 mm Hg, and diastolic blood pressure greater than or equal to 85 mm Hg conferred relative risks of 2.2, 3.2, 2.3, and 2.5, respectively, for developing nephropathy over 10 years.236
Diabetes management improvements over the last 30 years have markedly decreased the incidence of severe nephropathy in adults who were diagnosed with T1D as children.237,238
Annual testing for albuminuria is recommended for children from age 10 who have had diabetes for 5 years.211 The most convenient and reliable way to do this is to measure urinary albumin-to-creatinine ratio in a urine sample. Microalbuminuria in a single specimen may not indicate disease. Repeat testing is required to confirm persistent microalbuminuria. Orthostatic proteinuria is common and must be distinguished from that due to diabetic renal disease. A first-void morning urine should not contain abnormal concentration of albumin, but a late afternoon urine specimen often will if the albuminuria is orthostatic.
Although the Diabetes Care and Complications Trial demonstrated that microalbuminuria and overt nephropathy can be delayed or prevented by intensive diabetes treatment,183,239 the United Kingdom Proscriptive Diabetes Study and other studies have shown that blood pressure control is at least as important as glycemic control in prevention of nephropathy and in decreasing the rate of decline of glomerular filtration rate in adults with T2D.240
Persistent microalbuminuria should be treated with an angiotensin-converting enzyme (ACE) inhibitor titrated to maintain normal albumin excretion. ACE inhibitors increase afferent vessel dilatation and decrease elevated intraglomerular pressures, resulting in decreased albumin excretion. Although children and adolescents with microalbuminuria have higher blood pressures than those with normal albuminuria,241-244 the beneficial effects of ACE inhibitors are observed whether or not the patient has hypertension.245 Many pediatric diabetologists start therapy with enalapril 5 to 10 mg daily and titrate upward to achieve normal albuminuria and blood pressure.
Although high protein intake should be avoided in children with microalbuminuria, protein restriction below 15% of total daily calories is not recommended because of the need for adequate dietary protein in the growing child.
Adolescents should be counseled to avoid smoking, as nicotine has profound vasoconstrictive effects that have been associated with progression of nephropathy in adults with T1D.248
Retinopathy is the most common microvascular disease seen in children and adolescents with T1D. Retinopathy is broadly defined as (1) background retinopathy, with the presence of microaneurysms only or microaneurysms and occasional dot-blot hemorrhages, and (2) proliferative retinopathy, with growth of new vessels, glia, and fibrous tissue. Fluoresce-in angiography can document severity of retinopathy by showing microaneurysms, areas of retinal ischemia, proliferation of new blood vessels, and permeability of the retinal vessels.
At diabetes diagnosis, there is increased blood flow through the retina that may be associated with leakage of injected fluorescein into the vitreous,249 which is reversed by improved metabolic control. In addition, rapidly improving metabolic control may be associated with an initial worsening of diabetic retinopathy with, however, long-term improvement.221 Anatomic disease results from increased vascular permeability and capillary and arteriolar occlusion within the retina. The increased permeability results in edema and the formation of hard exudates, whereas the occlusion results in formation of microaneurysms, hemorrhages, soft exudates and capillary dropout. The growth of new blood vessels, glia, and fibrous tissue in front of the retina can result in vitreous hemorrhage and retinal detachment from shrinkage of the proliferating tissue.
Although retinopathy has been reported to be present early in the course of childhood diabetes,250 it usually is not recognized before 5 to 10 years of diabetes duration and after the onset of puberty. By that time, 20% to 30% of patients will have background retinopathy, 30% to 50% after 10 to 15 years diabetes duration, and 70% to 80% after 15 years of diabetes.251
In the EURODIAB prospective complications study of 764 patients greater than 15 years of age, retinopathy incidence was 56% after 7 years of follow-up. Risk factors included high albumin excretion rate, elevated cholesterol and triglyceride levels, and increased waist-to-hip circumference ratio. In contrast to other studies, there was no association between retinopathy and blood pressure, cardiovascular disease, or smoking.252
The Pittsburgh Epidemiology of Diabetes Complications found that systolic blood pressure (BP) greater than or equal to 120 mm Hg conferred a relative risk of 1.6 for the development of proliferative retinopathy, and the relative risk was 2.7 if systolic BP was greater than or equal to 130. Diastolic BP greater than 79 mm Hg conferred a relative risk of 1.8, if diastolic BP was greater than or equal to 85 relative risk was 2.4, and if diastolic BP was greater than or equal to 90 mm Hg, the relative risk of proliferative retinopathy was 4.6.236
An ophthalmologic evaluation at the time of diagnosis and after stabilization may be useful to assure that no eye disease or uncorrected visual limitation is present. Annual ophthalmologic evaluation should begin after age 10 and following 3 to 5 years of diabetes.221 Recent studies indicate, however, that biennial screening may be adequate in well-controlled children.254 ACE inhibitors slow the progression of retinopathy in patients with and without hypertension; thus, retinopathy is an indication for ACE inhibition.255 ACE inhibitors are contraindicated in pregnancy.
Retinal photocoagulation has markedly improved the prognosis for vision in patients with proliferative retinopathy.256 The photo-coagulation destroys ischemic retinal tissue, thus removing the stimulus for neovascularization. Laser therapy is also used for macular edema by destroying leaking microaneurysms and dilated capillaries.
Neuropathy
Although symptomatic neuropathy is uncommonly seen in children and adolescents with diabetes, sensory and autonomic motor nerve impairment can be demonstrated in young people. Peripheral neuropathy, including motor and sensory disturbances, as well as autonomic neuropathies (gastrointestinal, cardiovascular, vasomotor instability, and hypoglycemia unawareness) can be seen in children and adolescents with type 1 diabetes. Peripheral sensory function testing should be done at each clinic visit by gently bending a 10-g monofilament against the great toe. Loss of ability to feel the monofilament requires further evaluation.
The pathogenesis of neuropathy in diabetes involves both neural and vascular components. The aldose reductase system in the lens and peripheral nerves is responsible for metabolism of glucose to sorbitol with elevated glucose levels accelerating the synthesis of sorbitol, which is not freely diffusible, exerting an osmolar affect within the nerve and causing swelling and eventual destruction. Myoinositol, a component of neuronal cell membranes involved in the control of neural transmission, is decreased in animals and humans with diabetes. The basement membrane of the Schwann cell is thickened in diabetes, and the vasa nervorum show capillary and arteriolar wall thickening as the result of basement membrane accumulation. This can be considered another microvascular complication of diabetes. Numerous thrombosed vessels, axonal loss, and a characteristic segmental demyelination are demonstrated on biopsy of affected nerves.
Symptoms of peripheral neuropathy include bilateral numbness and paresthesias, especially pain and burning in the lower extremities, which is much worse at night. A decrease in vibratory sense is the usual first clinical sign of neuropathy, followed by loss of ankle jerks and later by loss of pinprick sensation in a stocking distribution.257 The Pittsburgh Epidemiology of Diabetes Complications study found that 3% of 65 children under 18 years of age had neuropathy based on history and physical examination.258 Subclinical neuropathy with decreased motor nerve conduction velocity and sensory change is much more common, however, in as many as 50% to 72% of adolescents.259
Neuropathy has been correlated with hyperlipidemia, smoking,259 limited joint mobility,260 and albuminuria.261 Among 1172 patients followed for an average of 7.3 years, neuropathy, determined by clinical evaluation, quantitative sensory testing, and autonomic function tests, developed in 23.5% of patients. Risk markers were HbA1c, duration of diabetes, and modifiable cardiovascular risk factors such as LDL cholesterol, triglycerides, body mass index, smoking, and blood pressure.256
Improved metabolic control may result in resolution of sensory and motor nerve dysfunction as well as autonomic symptoms. The Diabetes Care and Complications Trial showed that intensive diabetes therapy decreased clinical neuropathy by 35% to 90%.183 Peripheral motor and sensory nerve conduction velocities were significantly faster after 5 years of intensive therapy compared to conventional treatment in the adolescent cohort.261
Manifestations of autonomic neuropathy include gastroparesis, cardiovascular reflex loss, and hypoglycemic unawareness. Diabetic gastroparesis is associated with decreased gastric motility and delayed emptying time. Affected patients often complain of bloating and feelings of satiety following intake of small amounts of food, and they may have anorexia and weight loss. Diagnosis is by study of gastric emptying. Gastroparesis also results from hyperglycemia, so improved metabolic control is important for management.262,263 Prokinetic agents including metoclopramide, domperidone, tegaserod, and erythromycin may alleviate symptoms.
Autonomic neuropathy of the cardiovascular system affects heart rate, typically with fixed heart rate, and defects in central and peripheral vascular dynamics. There is hypersensitivity to catecholamines and an inability to increase cardiac output with hypotension on standing or with exercise. Cardiovascular autonomic neuropathy also increases the risk for painless myocardial ischemia and fatal cardiovascular events.264
Hypoglycemia unawareness is the loss of the catecholamine-induced symptoms of sweating, tachycardia, nausea, anxiety, and tremulousness that normally accompany severe drops in blood glucose concentrations.
MACROVASCULAR DISEASE AND HYPERLIPIDEMIA
Diabetes confers a two- to fourfold increased risk for cardiovascular disease.265-268 Factors that further independently increase cardiovascular risk in people with diabetes include smoking, hypertension, dyslipidemia, renal dysfunction, and hyperglycemia.269
The increased cardiovascular risk in type 1 diabetes (T1D) may be due to a number of factors, including endothelial dysfunction, increased arterial stiffness, and loss of endothelial integrity.267,271,272 The endothelium controls vascular tone through the production of nitric oxide. Hyperglycemia increases reactive oxygen species that directly reduces the bioavail-ability of nitric oxide, resulting in endothelial dysfunction.273 Endothelial dysfunction is a precursor to atherosclerosis, and its measurement can be used to predict cardiovascular events.
Tight glycemic control may decrease cardiovascular event rates in patients with T1D and may provide the same “metabolic memory” effect demonstrated by the Diabetes Care and Complications Trial (DCCT) follow-up studies for microvascular complications. Mean HbA1c levels 1 year after conclusion of the DCCT were nearly identical between the former intensive and former conventional groups. While the Epidemiology of Diabetes Interventions and Complications cohort is still relatively young and the total event rates are still quite small, the intensive insulin therapy group demonstrated lower cardiovascular event rates than the conventional therapy group.277
Treatment of Hyperlipidemia
The American Diabetes Association (ADA) recommendation is that adults with diabetes maintain LDL cholesterol less than 100 mg/dL, triglycerides less than 150 mg/dL, and high-density lipoprotein (HDL) greater than 40 mg/dL.278 Even lower LDL levels may further decrease cardiovascular disease in high-risk patients.279 The ADA clinical practice recommendations now suggest an LDL goal of less than 70 mg/dL in high-risk patients with diabetes and cardiovascular disease.278 That fatty streaks and atherosclerotic plaques are present during childhood indicates that the process begins early, suggesting that it is reasonable to apply adult intervention goals in children who have T1D.
If LDL cholesterol is borderline (100–129 mg/dL; 2.6–3.4 mmol), or elevated (≥ 130 mg/dL; 3.4 mmol), dietary intervention to decrease total and saturated fat in the diet should be initiated and a repeat lipid profile obtained in 6 months. If LDL cholesterol remains elevated after 6 months of attempting to improve blood glucose control and diet, pharmaco-therapy is warranted. Statins are as safe and effective in children as in adults and should be the first pharmacologic intervention.183,280,281 The usual starting dose of atorvastatin is 10 mg, after normal liver function is verified. Fasting lipid levels should be rechecked 6 weeks after starting therapy and the dose should be titrated upward at 10-mg increments to achieve the target level of LDL cholesterol. Adolescent females should be warned of the teratogenic potential of statins. Cholesterol absorption inhibition can be used as adjunct therapy, adding ezetimibe 10 mg daily if the LDL cholesterol goal is not reached with 20 mg atorvastatin. Triglyceride elevation should also be vigorously treated. Isolated hypertriglyceridemia may be seen in some patients unable to achieve optimal blood glucose levels. In those instances, fenofibrate (48 mg daily), should be considered. Tryglycerides should be rechecked 6 weeks after initiating therapy and the dose titrated upward to achieve normal triglyceridemia.
HYPERTENSION
The United Kingdom Prospective Diabetes Study showed that above a systolic blood pressure of 110 mm Hg, the higher the blood pressure, the greater the risk of cardiovascular disease.282
Treatment of Hypertension
Children with high-normal blood pressure, defined as average systolic or diastolic blood pressure greater than the 90th percentile for age, sex, and height, should reduce salt intake and increase exercise (30–60 minutes of daily exercise that raises heart rate). If blood pressure has not improved after 3 to 6 months of lifestyle interventions, pharmacologic treatment is indicated. Hypertension, defined as average systolic or diastolic blood pressure consistently greater than the 95th percentile for age, sex, and height on 3 separate occasions, should be treated with angiotensin-converting enzyme inhibitors.278,280
TYPE 2 DIABETES
T2D in children and adolescents is becoming an increasingly important public health concern throughout the world.8,28,284-301 In North America, T2D comprises 30% of all newly diagnosed diabetes in patients 10 to 20 years of age.302 Not surprisingly, this epidemic is closely associated with the increased prevalence of obesity among youth of all ethnic backgrounds, as increased visceral adipose tissue produces adipokines that increase insulin resistance.303 As in adult T2D, children with T2D typically have the insulin-resistance/metabolic syndrome, which includes abdominal obesity, disturbed glucose regulation, dyslipidemia, and hypertension.304
EPIDEMIOLOGY
Beginning around 1990, pediatric endocrinologists noticed a sharp increase in the numbers of patients with T2D.28,286,305-307 This was predominantly, but not exclusively, seen in the African American and Mexican Hispanic-American population, suggesting ethnic differences in background insulin sensitivity. Indeed, in Asian youth and adults, T2D occurs without overall obesity, but increase in intra-abdominal fat accumulation.308 In studies from Cincinnati, Arkansas, and Texas, African Americans accounted for 70% to 75% of pediatric diabetes.286,306,309 It is estimated that one third of Mexican American children and youth with diabetes in Southern California and over two thirds of those in South Texas have T2D mellitus.310,311
Reports from throughout the United States indicate that the percentage of patients with new-onset diabetes who have T2D increased from 2% to 4% in 1994 to 20% to 50% by 2000.301
The recognition of an epidemic of T2D is not unique to North America (eTable 544.2 ). In Japan 80% of all new cases of diabetes in children and adolescents in 2000 were T2D.
Other reports indicate that increasing rates of T2D with concurrent increases in obesity are also being observed in children in Thailand, China, India, New Zealand, Australia, and throughout Europe.301 The prevalence of T2D in England is estimated at 0.2%, and this problem is expected to be more prevalent with the epidemic of childhood obesity in that country.316
A constant in the emergence of type 2 diabetes (T2D) in young patients has been the association with increasing rates of obesity. The US National Health and Nutrition Examination Survey conducted between 1988 and 1994 found that 20% of children 12 to 17 years of age had body mass indexes above the 85th percentile for age, the definition of overweight, and that depending on ethnicity, 8% to 17% were obese, with a body mass index greater than the 95th percentile. Not only was there a doubling of the frequency of childhood obesity since 1980, but the severity of obesity was greater (see Chapter 32).317
ETIOLOGY
The recent increase in T2D prevalence in young patients has been so rapid that it can only be explained by changes in the environment. The most important of these is the increasing prevalence of obesity.318-324 Nonetheless, not all or even a majority of obese youngsters develop T2D, emphasizing the importance of underlying genetic predisposition. The thrifty genotype hypothesis, advanced nearly 40 years ago and recently updated,325,326 explains the insulin resistance and relative β-cell insufficiency that is associated with the development of obesity and T2D as an adaptation for conserving energy and surviving famine. Until the modern era of ready availability of high-calorie foods, such a genotype would have had great survival advantage.
Numerous studies have demonstrated that both insulin resistance and limited pancreatic β-cell capability to respond to the increased insulin requirements associated with obesity are the basic abnormalities in the development of T2D.327-333
Insulin resistance affects glucose, lipid, and protein metabolism. Normal glycemic control requires the sensing of glucose concentration by the cells, the synthesis and release of insulin, the coupling of insulin to its receptors, and postreceptor activation of specific signal transduction pathways. This results in increased glucose uptake by muscles, fat, and liver tissue, with decreased glucose production by the liver. In T2D, peripheral insulin resistance in muscle and fat tissue, taken together with decreased pancreatic insulin secretion and increased hepatic glucose output, result in hyperglycemia (Fig. 544-3).334
Low Birth Weight
The association of lower birth weight, smaller head circumference, and thinness at birth, with later development of insulin resistance and impaired glucose tolerance or T2D suggests in utero programming that limits β-cell capacity and induces insulin resistance in peripheral tissues.335,336 The maternal undernutrition that led to low birth weight is thought to impair development of the endocrine pancreas. The effect of fetal undernutrition on adult glucose tolerance has been confirmed in large studies in Sweden and the United States.337,338 Glucose intolerance was also found in adults who were the offspring of mothers who had starved during the last trimester of pregnancy during the Dutch famine near the end of World War II.339
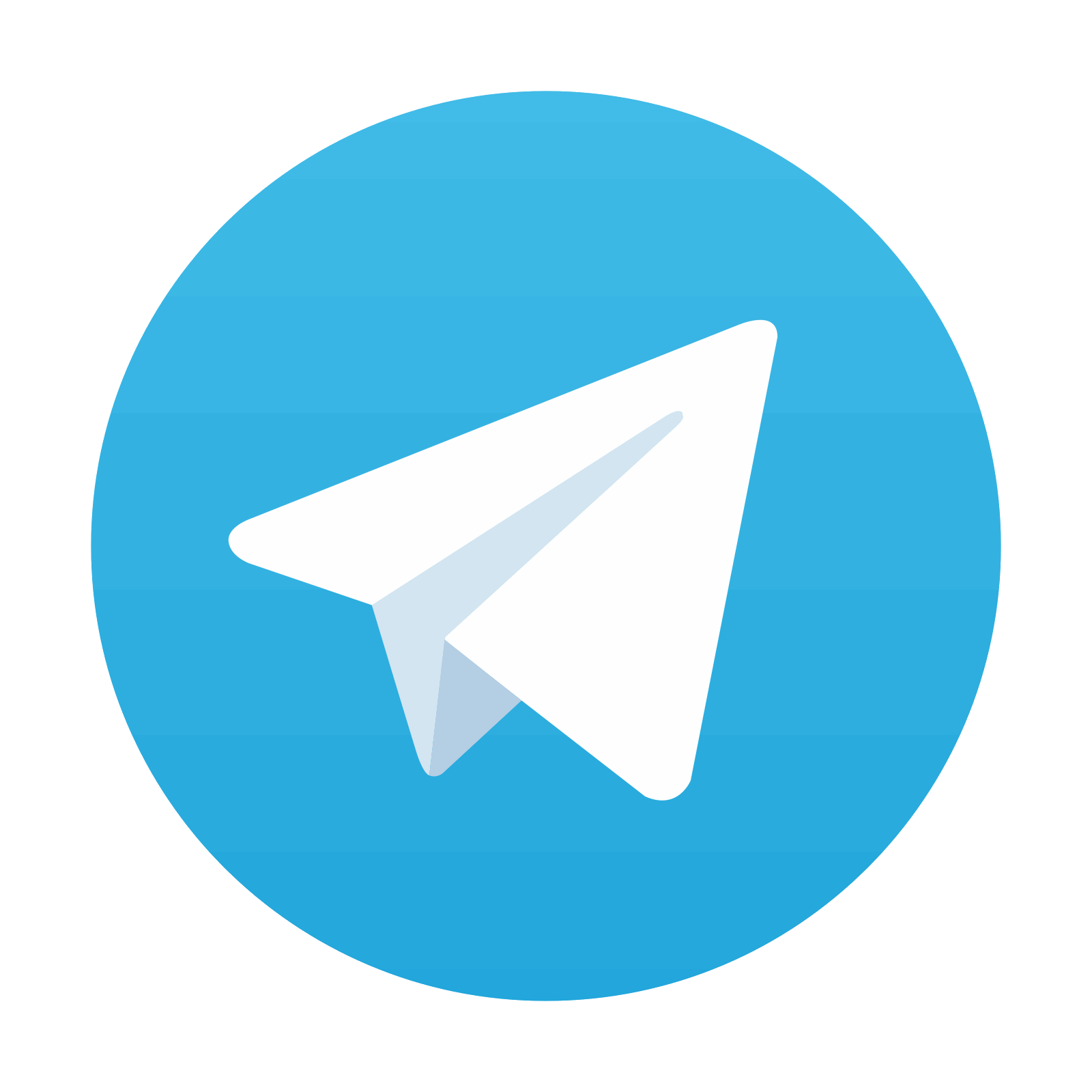
Stay updated, free articles. Join our Telegram channel

Full access? Get Clinical Tree
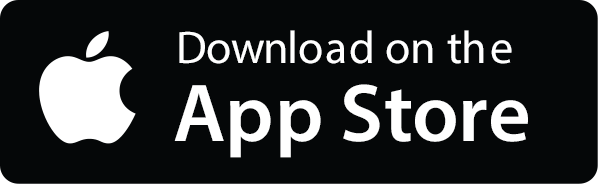
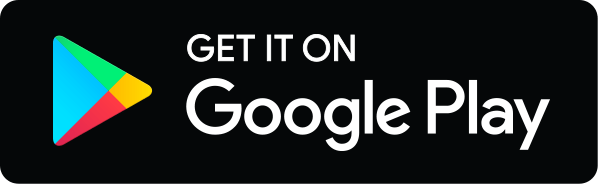