Key Points
- •
The heart is largely derived from mesoderm.
- •
A single heart tube forms with venous and arterial connections. It elongates by addition of cells at either end from the surrounding mesenchyme.
- •
The tube folds to the right and lies in the pericardial cavity.
- •
The atria and ventricles form by ballooning from the tube; septation of the atria and ventricles is largely achieved by this method.
- •
The endocardial cushions at the atrioventricular (AV) junction and outflow tract complete septation of the AV junction and outflow tracts and give rise to the AV and arterial valves.
- •
The epicardium gives rise to the mesenchyme of the heart, the veins and most of wall of the coronary arteries.
- •
The epicardial coronary arteries grow into the aortic root.
- •
The conduction tissue is derived from primitive myocardium of the original heart tube.
- •
A complex series of venous precursors contribute to the abdominal veins.
- •
Aortic arches 3, 4 and 6 give rises to the vessels of the head, neck and thorax.
Introduction
The heart is an organ largely derived from mesoderm, with a contribution from ectodermally derived neural crest cells. Its development is critically dependent upon a cascade of events tightly regulated in time and space. The first recognisable sign of heart development in the embryo is the formation of the heart tube within the lateral splanchnic mesoderm late in the third week. Invisible to the classical morphologist are a series of preceding genetic steps on which the morphological changes are critically dependent. These include the specification of precursor cells in the bilaminar embryonic disc, their migration to the heart fields and the specification of cell types within the developing heart. By the end of the seventh week, the heart, although tiny, is essentially fully formed. It does not develop in isolation but is intimately related to surrounding structures such as the pharynx, and heart development is both influenced by and influences these surrounding structures. Unsurprisingly, defects in the heart are associated with defects in these associated structures. From very early in its development, the heart is a beating structure and contains a flowing liquid, so mechanical forces are also important in shaping its development.
Heart development is contributed to by multiple genes, many of which have multiple functions in development, with considerable overlap. If a defect develops, it is likely to be modified by growth and subsequent alterations in haemodynamics. Because heart development is a sequential and complex process, no single gene defect leads to a single specific heart defect. There has been a proliferation of information on heart development in recent decades, with genetic experiments in mouse, chick and zebrafish embryos having determined details of cell lineage. Although much remains unknown, the genetic mechanisms regulating heart development are beginning to be understood.
Brief Overview of Heart Development
The bulk of the work on early cardiac development comes from studies in fish, chick and mouse embryos.
Within the cardiac crescent (the group of mesodermal cells lying in the splanchnic mesoderm anterior to the buccopharyngeal membrane and that show patterns of gene expression committing them to cardiogenesis), paired endothelial-lined tubes develop with their long axis in the long axis of the embryonic disc ( Fig. 10.1 ).

With folding of the embryo, these paired tubes fuse medially over part of their length to form the primitive single heart tube. The surrounding mesoderm of the cardiac crescent differentiates to provide an investing sleeve of myocardium, both layers separated by extracellular matrix termed cardiac jelly . The inflow is caudal, a continuation of the primitive veins, and the outflow cranial and connected to the paired dorsal aortae.
The pericardial cavity develops initially on the dorsal surface of the developing heart as part of the intraembryonic coelom. With folding of the embryo and formation of the single heart tube, the pericardial cavity comes to lie ventral to the heart tube, which is connected dorsally to the mesenchyme by the dorsal mesocardium. The septum transversum grows into the intraembryonic coelom partially to divide the pericardial cavity from the peritoneal cavity. The pericardial cavity is further divided by ingrowth of tissue from the lateral body walls, the pleuropericardial folds, that fuse with each other and the foregut mesenchyme to completely enclose the pericardial cavity and create two pleural cavities still joined to the peritoneal cavity. The dorsal mesenchyme breaks down to leave the heart connected to the body wall only at the arterial and venous poles. The space thus created is the transverse pericardial sinus.
The primitive myocardium of this primary heart tube shows regular contractions by the third week (embryonic age). This heart tube elongates by addition of mesodermal cells at its two poles (and from the dorsal mesocardium until that structure involutes) and undergoes rightward looping ( Fig. 10.2 ). The primitive heart tube is the scaffold to which the atrial and ventricular chambers are added by ballooning from its myocardium. Septa are formed, dividing the right and left sides of the atria, ventricles and great arteries.

Heart formation is completed with the development of valves, the conduction system and the formation of the coronary arteries by the ingrowth of extracardiac tissues derived from the neural crest and from the proepicardium situated in the septum transversum.
The Heart Fields
Heart progenitor cells are located in two small patches, one on either side of the midline in the epiblast of the bilaminar embryonic disc, close to the cranial part of the primitive streak. The earliest known committed cardiac precursors express the T-box transcription factor Eomesodermin\Tbr2, which activates another transcription factor MESP1 . These progenitor cells migrate together through the primitive streak and form two plates of lateral mesoderm cells positioned anteriorly. Specification of cardiogenic mesoderm has already started during this cellular migration. The progenitor cells migrate such that the medial-lateral arrangement of these cells will become the cranial-caudal axis of a linear heart tube.
With the formation of the embryonic coelom, they occupy the splanchnic mesoderm and fuse in the midline cranially to form the cardiac crescent. The cells of this cardiac mesoderm express the cardiac specific transcription regulator genes NKX2.5 and GATA-4 .
The cells of the splanchnic mesoderm, one on each side of the body, interact with adjacent tissues ( Fig. 10.3 ). The splanchnic mesoderm is positioned adjacent to the foregut endoderm (see Fig. 10.1 ), which is thought to provide inductive signals to begin myocardial differentiation. Endoderm also has a mechanical role in assembly of the heart tube. By its active contraction, it pulls the bilateral fields of cardiogenic mesoderm towards the midline, permitting them to fuse and form a single heart tube.

The primitive, single heart tube initially functions not so much to support the embryonic circulation as to provide a scaffold into which the cells from the second heart field migrate to effect chamber formation. The second heart field cells are first located medial to the cardiac crescent and subsequently lie in mesoderm underlying the pharynx before they are added to the heart. The cranial part of the second heart field, the anterior heart field, which is identified by FGF10 expression, contributes to the formation of right ventricular and outflow tract myocardium. Cells in the posterior second heart field express Isl1, but not anterior heart field markers, contribute to atrial myocardium.
The nomenclature of the heart fields may be confusing because not all authors use exactly the same terms, and the heart fields are dynamic, changing in position and with time.
The first and second heart fields develop sequentially, and the anterior and posterior heart fields describe anatomical positions.
It appears that the overall cellular environment is critical for this process because cells taken from a different location or at a different developmental time point can contribute to the heart when placed in the appropriate location.
The Heart Tube
Within the cardiac crescent, the primitive heart tube forms. The horseshoe-shaped cardiac crescent forms a tube with two caudolateral inlets, or venous pole, and one craniomedial outlet, or arterial pole. The process starts with the formation of endothelial-lined channels in the mesenchyme that form a plexus that eventually coalesces to form paired endothelial tubes. Folding of the embryo then brings these paired tubes together in the midline where they fuse to form a single endothelial-lined tube with a surrounding cuff of mesenchyme, separated from it by extracellular matrix termed cardiac jelly. The endocardium develops simultaneously with the myocardium and is a specialised endothelial cell type derived from the splanchnic mesoderm that, via an unknown mechanism, differs from the myocardial precursors.
The linear heart tube subsequently grows by addition of cells from a proliferating pool of precursors located external to it in the heart fields and not by division of myocytes in situ. Being added to the heart, their fate is not fixed, and their identity depends on their eventual location.
The cardiac precursors are therefore initially situated cranial and lateral to the future mouth but caudal to the mesoderm that forms the transverse septum and contributes to the formation of the diaphragm. During the process of folding, the cardiac precursors end up between the mouth on the cranial aspect, the diaphragm at the caudal aspect, and ventral to the foregut, as in the adult situation.
By folding of the embryo, the lateral parts of the cardiac mesoderm are brought together, forming the ventral part of the heart tube. The inner curvature of the cardiac crescent forms the dorsal side of the tube and is contiguous with the dorsal mesocardium, the attachment of the heart to the body wall.
The peripheral part of the cardiac crescent will eventually face the transverse septum and form the venous pole of the heart, and the central part of the crescent, which forms the outflow tract, is contiguous with the pharyngeal mesenchyme. This intimate association of the cardiac and facial region during development explains the high incidence of combined cardiac and facial malformations in syndromic conditions.
Retinoic acid appears critical in specifying these posterior precursor cells to become the inflow, or venous parts of the heart, the sinus venosus and atria,
Contraction
The myocardium shows regular contractions by the third week, meaning that the structural requirements for contraction, namely contractile proteins, sarcoplasmic reticulum and gap junctions, are already present in the myocardial cells of the primitive heart tube. It would appear that the initial contractions, at least in the chick embryo, are not essential for tissue oxygen and nutrient supply but likely have an important function providing mechanical stimuli for further development of the heart.
Looping of the Heart Tube
The myocardium of the primitive heart tube demonstrates TBX2 and TBX3 expression. Traditionally, five consecutive segments of the tube are recognised: venous, atrial, left ventricular, right ventricular and arterial. Between each of the segments is a transition zone – the sinoatrial ring, the atrioventricular (AV) canal, the primary heart fold and the ventricular outflow tract. It is important to recognise that the cardiac chambers develop as outgrowths from these segments and that the original segments form the connections of the cardiac chambers rather than the chambers themselves.
Looping of the straight heart tube follows activation of a gene cascade that determines right–left symmetry. Left–right differentiation has already started at the time of gastrulation with the formation of the Hensen node. Nodal cilia beat in one direction and cause a gradient of molecules across the bilaminar embryo. These genes are already expressed in the cardiac mesoderm before formation of the heart tube and include NODAL , LEFTY and PITX2, of which PITX2 is the effector gene. Furthermore, the direction of looping is not random but is controlled by genes not yet understood and is not under the control of PITX2 .
The tube loops to the right (see Fig. 10.2 ), which causes the transition zones to be brought into close proximity on the inner curvature of the loop. This is absolutely essential for establishing the correct connections of the chambers. Actin polymerisation-driven myocardial cell shape changes have been found to contribute to the bending of the heart tube, but the torsional component of looping is largely caused by forces from its encapsulating membrane, the splanchnopleure.
The looped heart tube has unidirectional blood flow, which was originally thought to be peristaltic but is now regarded as functioning as a Liebau pump (unidirectional and pulsatile flow resulting when an elastic tube containing fluid is periodically squeezed ). At this stage, the AV cushions function as primitive valves to permit unidirectional flow of blood in the looped heart tube.
Development of the Chambers and Outflows
Both atria and ventricles develop by ballooning growth from the heart tube ( Fig. 10.4 ), the atria growing from the dorsal aspect and the ventricles from the ventral aspect. Atrial segment growth is bilateral and in parallel; hence, it is possible to develop isomerism ( Fig. 10.5 ). Ventricular segment growth is unilateral and in sequence; therefore development of isomerism is not possible.


The initially formed atrial myocardium only gives rise to the trabeculated, atrial appendages in the formed heart. All smooth-walled myocardium found in the full-grown heart is added later during development from cells of the posterior heart field. Histologically, chamber formation becomes evident when the extensive extracellular matrix between endocardium and myocardium, known as cardiac jelly, disappears and trabeculations become evident in both atria and ventricles.
Numerous genes are involved in this process, the primitive myocardium of the primary heart tube expressing TBX2 and TBX3 , and the myocardium that forms the chambers shows specific gene expression ( ANF, CX40, CX43 ) but not TBX2 and TBX3 . The early markers of chamber formation remain restricted to the original trabeculated myocardium, with NOTCH, ERBB, and Ephrin playing roles in trabeculation. The compact ventricular layer does not express ANF and CX40 , but NOTCH, bone morphogenetic protein (BMP), and fibroblast growth factor play roles in compact myocardium development.
Myocardial ‘compaction’ is a slightly misleading term because it is not a process by which the previously trabeculated myocardium becomes compact but rather one in which the myocardium of the epicardial side of the ventricular wall proliferates to form the compact layer; when the compact outer layer starts to form, proliferation in the ventricular trabeculations ceases. This process may be related to mechanical strain because there is a gradient of strain across the ventricular wall, greatest on the inner surface and least on the outer surface. The curvature of the heart therefore depends on cell shape changes at the cellular level caused by a complex interplay on haemodynamic shear stress, contractive wall strain and electrical activity.
It remains uncertain precisely how the left and right ventricles achieve their different morphologies, although there are differences in gene expression between the left and right ventricles, with the cardiac transcription factor TBX5 expressed in a gradient tapering off toward the right ventricle.
Distal to the ventricle is the bulbus cordis. It is divided into three components, the proximal part forming the trabeculated part of the right ventricle, the midpart (conus cordis) forming the outflow tract of both ventricles and the distal third the truncus arteriosus. The bulbus cordis is demarcated externally from the ventricle by the bulboventricular sulcus, and internally this is the site of the interventricular foramen.
Septation
Septation of the ventricles occurs between 5 and 7.5 weeks of gestation. Initially, because the atrial segment is connected to the left ventricular segment, there is no direct connection with the right ventricle, but blood can flow from the atria to the right ventricle during diastole via the interventricular foramen ( Fig. 10.6 ). Similarly, the outlet is connected initially solely to the right ventricle, but blood flows from the left ventricle to the outlet during systole via the interventricular foramen. The ventricular septum grows from the apex of the heart loop between the left and right ventricular segments, and its growth is largely appositional caused by ballooning of both ventricles. The interventricular foramen lies above the interventricular septum.

Atrial Septation
The atria incorporate the draining veins and form a pair of valves around the sinus venosus. Fusion of the anterior part of these valves creates the septum spurium, which contributes to closure of the atria. The primary atrial septum develops to the right of this and grows downwards towards the fused AV cushions ( Fig. 10.7 ) with the gap between its free edge of the primary septum and the AV cushions called the ostium primum. There is a mesenchymal cap on the edge of the primary septum that is continuous ventrally with the ventral AV cushion and dorsally with the dorsal mesenchymal protrusion and the dorsal AV cushion. Before obliteration of the ostium primum by fusion of the septum primum and the AV cushions, an opening forms by fenestration in the primary septum – the ostium secundum. The septum secundum then develops on the right side of the septum primum, beginning at about day 41, by infolding of the muscular wall of the atrium. The septum secundum is thicker and more muscular than the septum primum. It grows downwards, and its anterior part fuses with the endocardial cushions, but a defect remains – the oval fossa. The ostium primum is actually beneath the free edge of the septum primum and is obliterated when that edge fuses with the endocardial cushions at about day 38. The sinus venosus is incorporated into the right atrium, the coronary sinus representing its left horn. The right valve of the sinus venosus disappears in its upper part, but the lower part becomes the valve of the inferior caval vein (Eustachian valve) and the valve of the coronary sinus (thebesian valve) ( Fig. 10.8 ). Occasionally, a complete remnant of the right valve persists, termed a Chiari network ( Fig. 10.9 ). This may fill out like a sail and extend through the right AV valve. The left valve of the sinus venosus is incorporated into the developing septum secundum but occasionally remnants of it persist as thin threads attached to the right side of the septum.



The pulmonary vein enters the left part of the atrium and is incorporated into it. The exact site of development of the pulmonary vein remains controversial. The smooth wall of the body of the left atrium results from incorporation of the pulmonary veins into the atrium. Failure of connection of the pulmonary vein results in total anomalous pulmonary venous connection ( Fig. 10.10 ).

The atrial appendages hence represent the original parts of the primitive atrium. The smooth-walled part of the right atrium develops from incorporation of the sinus venosus and is termed the sinus venarum .
The Interventricular Septum
The greater part of the interventricular septum results from a process of apposition because of ballooning of the ventricular chambers, beginning at about day 26. The crest of the interventricular septum is at the site of the primary foramen and retains the genetic expression pattern of the primitive heart tube (expressing TBX3 and NOTCH1 ), fusing with the dorsal AV cushion.
The Atrioventricular Junction
The AV valves develop form the two endocardial cushions. During looping, the cardiac jelly is eliminated from much of the heart tube but persists at the site of the endocardial cushions at the AV junction as well as in the outflow tract. The cushions are originally acellular, but beginning on day 26, the epithelial-to-mesenchymal transition produces cells that populate the cushions. In the first stage of this process, a subset of endocardial cells lining the AV junction and the outflow tract transform into a mesenchymal phenotype mediated by BMP through transforming growth factor β (TGFβ) and Notch produced by the myocardium of the AV junction and invade the cardiac jelly. This invasive, proliferating mesenchyme progressively remodels the matrix, and the resultant cellular masses, now called cushions, continue to grow and extend into the lumen ( Fig. 10.11 ). In the AV canal, these cushions form superiorly and inferiorly and fuse in the midline to create right and left AV orifices, differential growth of the right AV canal having brought the right atrium into contact with the right ventricle. Fusion of the cushions with the developing interventricular muscular septum and the atrial primary septum completes septation of the atrial and ventricular chambers. This complex process may be defective and result in ventricular septal defects (perimembranous in this region) ( Fig. 10.12 ).


Lateral cushions also develop, attracting cells from the epicardium. The dorsal mesenchymal protrusion, also called the vestibular spine, is contiguous with the dorsal AV cushion and the mesenchymal cap of the primary atrial septum in the atria and is derived from extracardiac cells. Maldevelopment of the dorsal mesenchymal protrusion causes AV septal defect ( Figs. 10.13 and 10.14 ), and absence of the dorsal mesenchymal protrusion is seen in fetuses with Down syndrome ( Fig. 10.15 ).



Even before septation, laminar blood flow ensures separation of the right and left streams of blood. The primary foramen is that part of the primitive heart tube from which the ventricles balloon. It is sometimes referred to as the interventricular foramen but is not actually between the two ventricles but rather above them at the inner curvature of the heart (see Fig. 10.6 ). Blood flows through this foramen from the right atrium to the right ventricle during diastole and from the left ventricle to the aorta during systole. This foramen becomes septated by the membranous septum at about day 45. The AV canal develops ventral and dorsal cushions that separate the canal into right and left parts.
The outflow tract is separated by two ridges called the septal and parietal outflow tract ridges ( Figs. 10.16 and 10.17 ). In an adult heart, the membranous part of the ventricular septum is the remnant of the fused AV and outflow tract cushions. It has been proposed that the morphology of transposition of the great arteries is a defect of laterality akin to the heterotaxy syndromes ( Fig. 10.18 ).



Valves
The septal leaflet of the tricuspid valve and the aortic leaflet of the mitral valve arise from fusion of the dorsal and ventral AV cushions on the right and left sides, respectively. The septal leaflet detaches from the myocardium by cellular apoptosis. The mural leaflets of the two AV valves are formed from the lateral AV cushions, and the ventricular space grows behind these mural leaflets.
The semilunar valves form from the parietal and septal outflow tract cushions and two intercalated ridges. The parietal outflow tract cushion gives rise to the right aortic and pulmonary valve leaflets. The septal outflow tract cushion gives rise to the left aortic and pulmonary leaflets, and the right and left intercalated ridges give rise to the posterior aortic and anterior pulmonary leaflets. The cushion at the distal margin undergoes apoptosis to achieve a cusp. The AV and semilunar valves then mature by remodelling of their matrix.
Outflow Tract
The outflow tract connects the developing ventricles to the aortic sac that is connected to the symmetrical pharyngeal arch arteries. Septation starts at day 32 and occurs distally to proximally (see Figs. 10.16 and 10.17 ) with the cushions arranged in a spiral fashion. The outflow tract myocardium becomes incorporated into the developing right ventricle. The outflow tract is connected via the aortic sac to arch arteries 3, 4 and 6. A protrusion of pharyngeal mesoderm, the aortopulmonary septum, grows into the aortic sac and connects distally to the spiralling outflow tract ridges, which separates the sixth and fourth pharyngeal arch arteries.
The outflow tracts therefore have a complex origin, partly from the primary heart tube and partly from ingrowth of cells from two distinct sources, including the cardiac neural crest and the second heart field. The complex interaction of all these tissues gives rise to the ventricular outflow tracts, the arterial valves and the intrapericardial parts of the aorta and pulmonary trunk ( Fig. 10.19 ).

Coronary Circulation
The coronary arteries and veins develop by both vasculogenesis (the formation of vessels in situ) and angiogenesis (the formation of new vessels by sprouting from existing vessels) from cells that grow over the myocardium from the proepicardium ( Fig. 10.20 ). Both the endothelium and the medial smooth muscle of the coronary arteries derive from this source ( Fig. 10.21 ). These vessels link up and grow to join with the aorta ( Fig. 10.22 ).



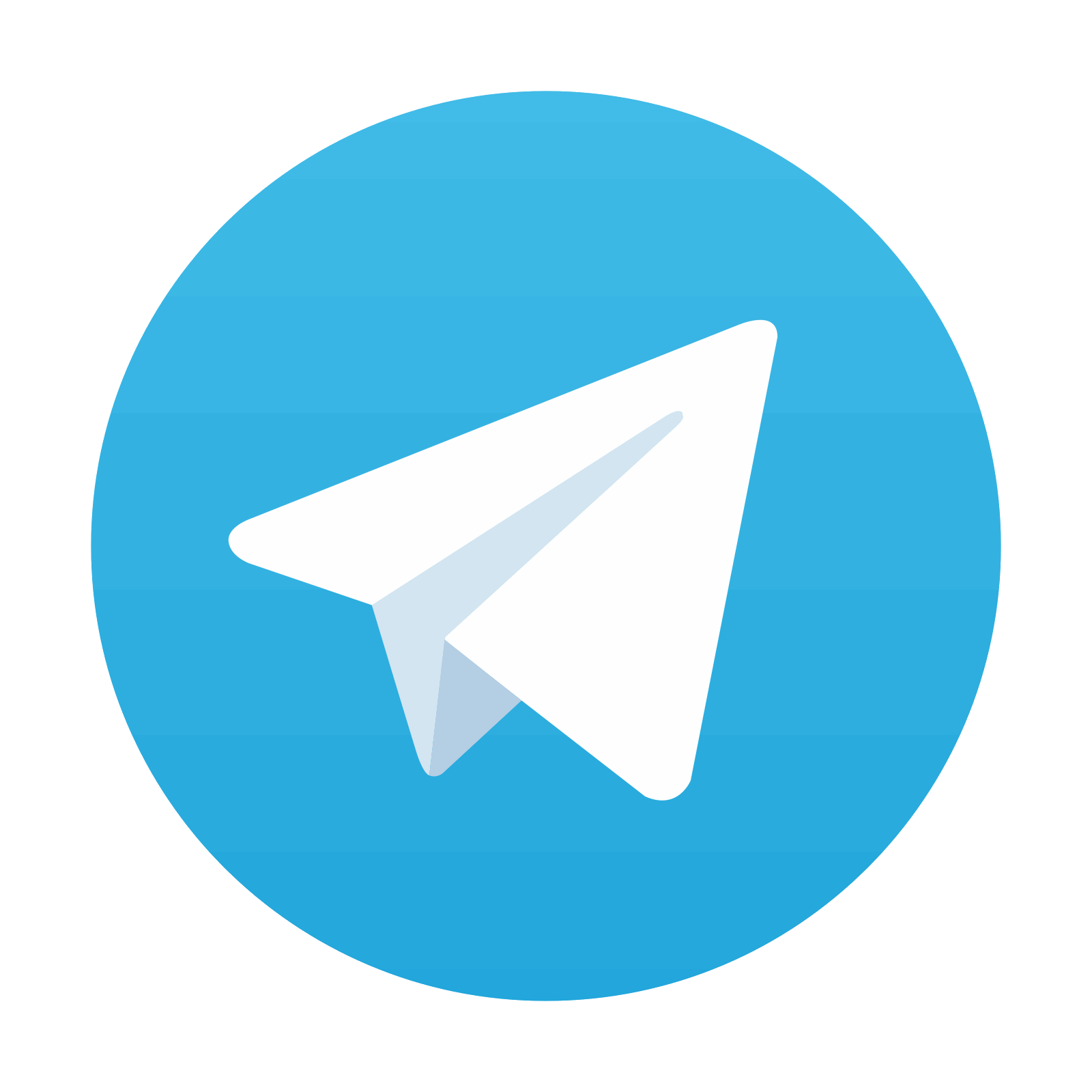
Stay updated, free articles. Join our Telegram channel

Full access? Get Clinical Tree
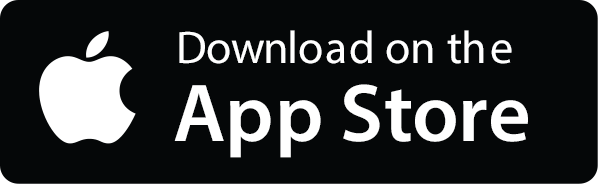
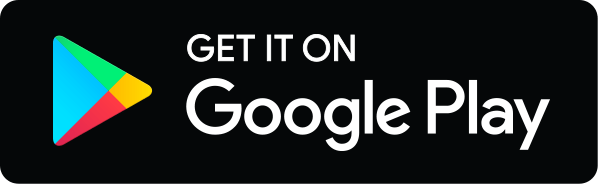