Introduction to Ventilators
A ventilator is defined as an automatic machine designed to provide all or part of the work required to generate enough ventilation to satisfy the body’s respiratory needs. Devices like resuscitation bags or a T-piece resuscitator are used to assist breathing but are not automatic and are not considered mechanical ventilators.
There was a time (the late 1970s) when textbooks describing ventilators emphasized individual mechanical components and pneumatic schematics of mechanical ventilators. Today, ventilators are incredibly complex mechanical devices controlled by multiple microprocessors running sophisticated software. Figure 25-1 shows a simplified pneumatic schematic diagram of a current-generation intensive care ventilator.

To keep this chapter practical, we restrict our description of ventilator design to a simplified discussion about general principles. From an engineering systems point of view, a ventilator can be viewed as having three main design characteristics: power inputs, power conversion and control, and power outputs. Beyond that, we need to understand some of the common design features of both the operator–ventilator interface (i.e., the control panels and displays) and the ventilator–patient interface (i.e., the tubing and adjunctive equipment that connects the ventilator to the patient).
Power Inputs
Work is force acting through a distance. For example, if you are asked to walk up a flight of steps, you do work in moving the mass of your body a certain distance above the earth. If you are asked to run up the steps, you may be disappointed to know that you are doing the same amount of work because it does not feel the same. What you are feeling is related to power, the rate of doing work. Similarly, it takes a certain amount of work as you inhale a breath. The larger the breaths and the faster you breathe, the more work you do per minute and the more power your body expends. Thus, power is a useful concept in understanding how ventilators are designed and operated.
Modern ventilators are powered by either electricity or compressed gas (early iron lung ventilators could actually be manually powered). Power is defined as the rate of doing work and is usually expressed in units of watts. Electrical power is calculated as the product of voltage and current required to operate the ventilator (watts = volts × amperes). Electricity, either from wall outlets (e.g., 110 to 220 V A/C, at 50/60 Hz) or from batteries (e.g., 10 to 30 V DC), is used to run compressors or blowers of various types. Batteries are used for transport or emergency power.
Alternatively, the power to expand the lungs is supplied by compressed gas. Pneumatic power in watts is equivalent to a flow of 1 L/s moving in response to a pressure gradient of 1 kPa. Compressed gas is usually supplied to ventilators from tanks or from wall outlets in the hospital (in the United States, hospitals supply about 50 psi from wall outlets). Some ventilators use compressed gas to power both lung inflation and the control circuitry, making them practical for transport and emergency use. Typically, ventilators are powered by separate sources of compressed air and compressed oxygen. This permits the control of oxygen concentrations between 21% (O 2 concentration in room air) and 100%. Although wall outlets supply air and oxygen at 50 psi, most ventilators have internal regulators to reduce this pressure to a lower level (e.g., 20 psi) to allow for safe operation in the case of fluctuating supply pressure. Compressed gas has all moisture removed. Otherwise, there could be condensed liquid in the tubing system, which could ruin ventilator pneumatic systems. Therefore, the gas delivered to the patient must be warmed and humidified to avoid drying out the lung tissue. A description of heating and humidification devices is beyond the scope of this chapter but is well described in current textbooks.
Power Conversion and Control
Input power must be converted (e.g., from electrical to pneumatic) to get the desired outputs of pressure, volume, and flow. Electrical power is converted to pneumatic power with either a compressor or a blower. A compressor generates a relatively low flow of gas at ambient pressure to a storage container at a higher level of pressure (e.g., 20 to 50 psi). Compressors are used to create stores of compressed air in hospitals. Smaller versions are also built into intensive care ventilators (avoiding the need for connection to the hospital supply). In contrast, a blower (also called a turbine) is smaller, consumes less electrical power, and generates relatively larger flows of gas directly to the ventilator output with a relatively moderate increase of pressure (e.g., 2 psi). Blowers are typically built into home care and transport ventilators, and more powerful versions are starting to appear in intensive care unit (ICU) ventilators.
Flow Control Valves
Once pneumatic power is produced, it must be controlled to achieve the desired output of flow to the patient. Flow is manipulated in various ways to achieve predetermined patterns of patient–ventilator interaction called “modes of ventilation” (see Chapter 15 ). Inexpensive microprocessors became available in the 1980s and led to the development of digital flow control valves. Digital control allows a great deal of flexibility in shaping the ventilator’s output pressure, volume, and flow. Such valves are used in the current generation of intensive care ventilators used for neonates.
The output control valve works in coordination with another valve, called the expiratory valve or “exhalation manifold.” When inflation is triggered, the output control valve opens, the expiratory valve closes, and the only path left for gas is into the patient. When inflation is cycled off, the output valve closes, flow from the ventilator ceases, and the exhalation valve opens, allowing the patient to exhale to the atmosphere. An additional function of the exhalation valve is to adjust the instantaneous expiratory flow path resistance to control the level of positive end-expiratory pressure (PEEP). There is a complex interaction between the output flow control valve and the exhalation valve enabling many different pressures, volume, and flow waveforms to be generated.
Control Subsystems
The output flow valve and the exhalation valve behavior are coordinated by the ventilator’s control system. Most ventilators use electronic control circuits with microprocessors and complex software algorithms to manage monitoring (e.g., from pressure and flow sensors) and control functions. The differences among modes of ventilation (and the ventilators themselves) are due to the control system software as much as to the hardware. Software determines how the ventilator interacts with the patient, i.e., the modes available.
Power Outputs
As we have seen, the ventilator takes input power (e.g., electricity) and converts it to flow. Then it generates output as the power that supports the work of breathing for the patient. This output power is a function of the ventilator settings, such as frequency and preset inflation pressure, as are often used in the ventilation of infants.
The clinically relevant outputs of a mechanical ventilator are the pressure, volume, and flow waveforms it generates in supporting the patient’s work (or more accurately, power) of breathing along with the measured or calculated data it generates and displays to the operator. A “waveform” is simply a graphic representation of a variable as a function of time. Most modern ICU ventilators have graphic displays that plot the variables of interest (pressure, volume, or flow) on the vertical axis with time on the horizontal axis. The best way to understand this subject is to start with idealized waveforms, meaning the waveforms that would exist in an ideal world with perfect machines and no interferences from leaks or patient breathing efforts. These waveforms can be easily generated for educational purposes by using graphs of mathematical models using a spreadsheet program like Excel. Understanding idealized waveforms, one can more easily interpret real-world waveforms displayed on ventilators.
Idealized Pressure, Volume, and Flow Waveforms
Typical waveforms available on modern ventilators are illustrated in Figure 25-2 . These waveforms are defined by mathematical equations that characterize the ventilator’s control system. They do not show the minor deviations, or “noise,” caused by extraneous factors such as vibration, flow turbulence, or the patient’s spontaneous breathing efforts. Remember that most ventilators have manual or automatic scaling of the horizontal and vertical axes, and this can dramatically affect the appearance of waveforms. Interpretation of ventilator waveforms requires an in-depth understanding of a number of underlying concepts (see UNDERSTANDING MODES OF VENTILATION below).

Ventilator Alarm Systems
Ventilators used for neonates in the hospital environment have a wide range of alarms. The most important alarms cover events that are life-threatening, like loss of input power or microprocessor malfunction. Other alarms cover events that can lead to life-threatening situations if not corrected quickly. These include things like high or low airway pressure, tidal volume, and minute ventilation or unusual ventilator settings such as an I:E ratio greater than 1:1. The ventilator may also provide alarms for external monitors such as pulse oximeters and capnometers.
Operator–Ventilator Interface: Displays
The operator interface allows the operator to adjust settings and to monitor the status of the ventilator and patient. Interface designs vary widely, ranging from just a few hardware knobs and gauges to digital touch screen “virtual displays” and hybrids that have hardware knobs and buttons combined with digital displays. Obviously, the more complex the ventilator the more complex the operator interface (e.g., a simple transport ventilator compared to an ICU ventilator). Yet among the complex ventilators there is still a wide range of “user friendliness” of interface design, and there is a need for standardization.
As far as monitored patient data are concerned, there are four basic ways to present the information: as numbers or text, as waveforms, as trend lines, and in the form of abstract graphic symbols.
Alphanumeric Values
Data represented in numeric form include both settings and measured values such as fraction of inspired oxygen (FiO 2 ), peak, plateau, mean and baseline airway pressures, inhaled/exhaled tidal volume, minute ventilation, and frequency. A wide range of calculated parameters may also be displayed, including resistance, compliance, time constant, percentage leak, I:E ratio, and peak inspiratory/expiratory flow, to name just a few. Alarms and alerts are commonly displayed as text messages. Some ventilators also present brief instructions to the operator about settings and alarms, and there may even be excerpts from the operator’s manual. See the sections below describing specific ventilators to see examples of the operator’s interface.
Trends
Aside from the current values of ventilator settings and measured values, we are often interested in how parameters related to mechanical support change over time. Therefore, many ventilators provide trend graphs of just about any parameter they measure or calculate. These graphs show how the monitored parameters change over variable periods of time ( Fig. 25-3 ). Significant events or gradual changes in patient condition can be easily identified. In addition, ventilators often provide an alarm log. This is usually a text-based list documenting such things as the date, time, alarm type, urgency level, and events associated with alarms including when activated and when canceled. Such a log could be invaluable in the event of a ventilator failure leading to a legal investigation.

Waveforms and Loops
Most ventilators display graphical depictions of pressure, volume, and flow waveforms (see Chapter 12 ). These waveforms are quite useful for adjusting ventilator settings or evaluating respiratory system mechanics. They are essential for assessing sources of patient–ventilator asynchrony such as missed triggers, flow asynchrony, and delayed/premature cycling and making appropriate corrections. Sometimes it is more useful to plot one variable against another as an x–y or “loop” display. Pressure–volume loop displays are useful for identifying optimum PEEP levels (to avoid atelectrauma) and optimum tidal volume (to avoid volutrauma). Ideally, loop displays for such usage should be made with patients who are paralyzed or heavily sedated (to avoid errors due to patient effort effects) and with very slow inflations (i.e., quasi-static curve), but that is rarely possible in newborn infants. Caution must be exercised because ventilators display loops under any ventilating circumstances, and hence the display may be meaningless when the patient is actively breathing. An example of a composite display showing numeric values, waveforms, and loops is shown in Figure 25-4 .

Patient–Ventilator Interface: Circuits
The ventilator output is connected to the patient input (i.e., the airway opening) by means of the patient circuit . There are three basic configurations ( Fig. 25-5 ). Home care and transport ventilators often use only one tube, called a single limb circuit, with a pneumatically controlled exhalation valve ( Fig. 25-5 , top) rather than having the exhalation valve built into the ventilator. The exhalation valve is controlled by a pressure signal from the ventilator, conveyed through small-bore tubing. This signal determines the timing of flow into and out of the patient for mandatory inflations and also may control the PEEP level.

Intensive care ventilators usually have the exhalation valve built into the ventilator and are connected to the patient with a double-limb circuit ( Fig. 25-5 , middle).
Noninvasive ventilation may use a mask instead of an artificial airway. Ventilators designed specifically for mask ventilation often have single limb circuits that are used without an exhalation valve ( Fig. 25-5 , bottom). In this case, the circuit or the mask has a carefully sized opening or port. The port provides a known leak. The relationship between circuit pressure and flow through the leak is programmed into the ventilator’s microcontroller. Thus, the ventilator can estimate the flow and hence the volume delivered to the patient by measuring the pressure in the circuit, calculating the leak flow, and deducting that from the total flow delivered by the blower.
Some intensive care ventilators measure flow at the airway opening using a small, usually disposable sensor. There are two basic types of flow sensors used with ventilators ( Fig. 25-6 ). One is called a pneumotachometer . It has a flow-resistive element such as a screen or plastic flap in the flow path. The pressure on both sides of the resistor is conducted to pressure sensors in the ventilator through small-diameter stiff tubing. The difference between the two pressures is proportional to flow. The second type of flow sensor is called a hot-wire anemometer . Very thin wires are placed in the flow path and heated. Gas flow passing over the wires carries away the heat. Therefore, the amount of energy required to maintain a stable temperature in the wires is proportional to flow.

Understanding Modes of Ventilation
A detailed description of the classification of ventilation modes can be found in Chapter 15 . Here, we briefly define some basic terms related to mechanical ventilation in general, with specific applications to neonatal ventilation highlighted along the way. The classification of modes is based on 10 basic technological concepts (maxims) that underlie all modes of ventilation. These concepts are each fairly simple and intuitively obvious. But taken together, they result in a classification system applicable to any mode on any ventilator.
Defining a Breath/Inflation
A breath/inflation is defined as one cycle of positive flow (inspiration) and negative flow (expiration) defined in terms of the flow–time curve ( Fig. 25-7 ). A breath is a spontaneous breath. An inflation (followed by exhalation) is a ventilator-generated “breath.” For the purpose of the proposed classification, a “spontaneous breath” (inflation) is one for which inflation is started (triggered) and stopped (cycled) by the patient. A mandatory inflation is one for which inflation is either started or stopped (or both) by the ventilator independent of the patient.

Assistance with Volume or Pressure Control
A ventilator assists breathing using either “pressure control” or “volume control” based on the equation of motion for the respiratory system:
P ( t ) = E V ( t ) + R V ˙ ( t )
This equation relates pressure ( P ), volume ( V ), and flow <SPAN role=presentation tabIndex=0 id=MathJax-Element-2-Frame class=MathJax style="POSITION: relative" data-mathml='(V˙)’>(V˙)(V˙)
( V ˙ )
as continuous functions of time ( t ) with the parameters of elastance ( E ) and resistance ( R ). If any one of the functions ( P , V , or <SPAN role=presentation tabIndex=0 id=MathJax-Element-3-Frame class=MathJax style="POSITION: relative" data-mathml='V˙’>V˙V˙
V ˙
) is predetermined, the other two are derived. The term control variable refers to the function that is controlled (predetermined or preset) during a ventilator cycle. This form of the equation assumes that the patient makes no inspiratory effort and that expiration is complete (no auto-PEEP). Volume control (VC) means that both volume and flow (variables on the right-hand side of the equation) are preset. In the literature, the following terms are often used interchangeably to mean VC: volume targeted, volume limited , and volume preset . Pressure control (PC) means that inflation pressure (the variable on the left-hand side of the equation) is preset. In practice, this means one of two things: (1) the peak inflation pressure is preset (i.e., airway pressure rises to some target value and remains there until inflation time is complete) or (2) inflation pressure is controlled by the ventilator so that it is proportional to the patient’s inspiratory effort. In the literature PC is often referred to as pressure targeted, pressure-limited , and pressure preset . Characteristic waveforms for VC and PC are shown in Figure 25-8 .

At this point we should point out that some ventilator designers consider the mode of ventilation to be simply the breath sequence. For example, the Covidien Puritan Bennett (PB) 840 presents the operator with the option of first setting the “mode” as Assist/Control (A/C), Synchronized Intermittent Mandatory Ventilation (SIMV), Bilevel, or Spont (being continuous mandatory ventilation (CMV), intermittent mandatory ventilation (IMV), and continuous spontaneous ventilation (CSV), respectively). Then the operator selects a combination of the control variable and targeting scheme for mandatory inflations and spontaneous breaths ( Tables 25-1 and 25-2 ). While this is a logical paradigm for selecting settings on an individual ventilator, it is not a good paradigm for a general mode classification system. All manufacturers have (understandably) tended to see the problem of describing modes from the narrow vision of their particular product rather than from the larger issue of understanding, classifying, and comparing modes in general. While the system proposed in Chapter 15 will probably never be embraced by all manufacturers, leading to an industry-wide set of standards for ventilator design, we can still hope that they at least describe modes the same way in their operator manuals. And even if that never happens, end users are now free to apply this knowledge on their own to understand and use the available technology.
Name | Abbreviation | Description | Advantage | Disadvantage | Example Mode Name | Ventilator | Manufacturer |
---|---|---|---|---|---|---|---|
Set-point | s | Operator sets all parameters of pressure waveform (pressure control modes) or volume and flow waveforms (volume control modes) | Simplicity | Changing patient conditions may make settings inappropriate | Pressure Control A/C | Babylog VN500 | Dräger |
Servo | r | Output of the ventilator (pressure/volume/flow) automatically follows a varying input. Currently implemented as inspiratory pressure proportional to inspiratory effort. | Proportion of total work of breathing supported by the ventilator is constant regardless of inspiratory effort | Requires estimates of artificial airways and/or respiratory system mechanical properties | Proportional Assist Ventilation Plus | PB 840 | Covidien |
Adaptive | a | Ventilator automatically sets target(s) between breaths in response to varying patient conditions | Can maintain stable tidal volume delivery with pressure control for changing lung mechanics or patient inspiratory effort | Automatic adjustment may be inappropriate if algorithm assumptions are violated or they do not match physiology | Pressure-regulated Volume Control | SERVO-i | Maquet |
BREATH CATEGORY | ||
---|---|---|
Mode | Mandatory | Spontaneous |
A/C | VC | NA |
PC | ||
VC+ | ||
SIMV | VC | PS |
TC | ||
PC | PS | |
VS | ||
PA | ||
TC | ||
VC+ | PS | |
TC | ||
Bilevel | PC | PS |
TC | ||
Spont | NA | PS |
VS | ||
PA | ||
TC |
∗ The word “mode” in this case refers to the breath sequence (continuous mandatory ventilation, intermittent mandatory ventilation, and continuous spontaneous ventilation), which are given names (assist/control, synchronized intermittent mandatory ventilation, and spontaneous, respectively).
To make this chapter practical for the user, we provide only a brief description of the unique features of each device and the available modes. We provide the list of available modes and their classification in the accompanying tables. For a general discussion of mode classification and definitions of the modes, please see Chapter 15 .
Universal Intensive Care Ventilators Used for Neonatal Ventilation
Up until the 1980s, there was an unequivocal need to have separate adult and infant ventilators, mainly because of technological limitations related to delivering small volumes to children and newborns. Today, most of the high-end ICU ventilators used in the United States are able to ventilate the whole range of patients from premature infants (weighing a few hundred grams) up to obese adults (over 400 kg). This is an amazing technological feat but by necessity involves certain compromises. Because the adult market is much larger than the neonatal and pediatric market, these devices are primarily adult ventilators that extend their reach into neonatal-size patients but do not address some of the unique aspects of neonatal physiology, allow for precise tidal volume measurement at the airway opening or ventilation in the presence of large leaks due to the use of uncuffed endotracheal tubes. Thus some of these devices may not be optimal for the smallest preterm infants. The most common “universal” ventilators are described below (in alphabetical order).
Note that the tables showing the modes for each ventilator pair the arbitrary names created by the manufacturers with the standardized ventilator mode taxonomy described in Chapter 15 .
Modes commonly referred to as volume targeted in the pediatric literature include both modes classified as VC and those that are classified as PC with adaptive targeting of tidal volume (either of which may have any of the three breath sequences).
CareFusion AVEA
Description
The CareFusion AVEA ventilator ( Fig. 25-9 ) is designed for intensive care ventilation of adult, pediatric, and neonatal patients.

Operator Interface
The AVEA’s operator interface has a touch screen, buttons, and a control knob. Settings are entered by touching a virtual button on the screen to select the desired setting, turning the knob to select the setting value, and then pressing the ACCEPT button to finalize the setting. The real buttons provide various features related to menu navigation, alarm silencing, suctioning, temporary 100% oxygen delivery, manual breath trigger, inspiratory hold, and expiratory hold.
Modes
Modes are selected by pressing the virtual button with the desired mode name. There are 10 basic mode names ( Table 25-3 ). All modes can be flow or pressure triggered. In addition, there are “advanced settings” that can be used to modify the main modes ( Table 25-4 ). Some of these advanced settings actually change the mode, resulting in many more modes by classification ( Table 25-5 ).
Setting | VOL/AC | VOL SIMV | PRES A/C | PRES SIMV | PRVC A/C | PRVC SIMV | CPAP/PSV | APRV/BIPHASIC | TCPL A/C | TCPL SIMV |
---|---|---|---|---|---|---|---|---|---|---|
Frequency | x | x | x | x | x | x | x | x | ||
Volume | x | x | x | x | ||||||
Peak flow | x | x | x | x | ||||||
Inspiratory pressure | x | x | x | x | x | |||||
Inspiratory time | x | x | x | x | x | x | x |
Advanced Setting | Action |
---|---|
Volume limit | For pressure control modes, sets a volume cycle threshold. Note that volume cycling of a pressure support breath changes it from spontaneous to mandatory. |
Machine volume | For pressure control modes, allows a volume target and flow and activates dual targeting. The operator sets the target volume and the ventilator calculates the target flow as the volume divided by the set inspiratory time. If flow decays to this flow target and the volume has not been delivered, then inspiration switches to volume control with constant flow until the volume has been delivered. Inspiratory time remains constant. Machine volume overrides flow cycle setting if activated. |
Flow cycle | For pressure control modes, changes the cycle criterion from time to flow and sets the threshold for inspiratory flow termination as a percentage of peak flow. |
Demand flow | For volume control modes, sets a ventilator-determined pressure target and activates dual targeting. If inspiratory pressure decreases 2 cm H 2 O (because of patient inspiratory effort), volume control switches to pressure control. If the set volume is delivered and flow is equal to the set flow, inspiration is volume cycled. Otherwise, inspiration is flow cycled at 25% of peak flow. |
Vsync | Switches the mode from volume control to pressure control with adaptive targeting. Inspiratory pressure is automatically adjusted to maintain an average tidal volume equal to the set volume. |
MODE CLASSIFICATION | |||||
---|---|---|---|---|---|
Mode Name | Control Variable | Breath Sequence | Primary Breath Targeting Scheme | Secondary Breath Targeting Scheme | Tag |
Volume A/C | Volume | CMV | Set point | N/A | VC-CMVs |
Volume SIMV | Volume | IMV | Set point | Set point | VC-IMVs,s |
Volume SIMV with artificial airway compensation | Volume | IMV | Set point | Set point/servo | VC-IMVs,sr |
Volume A/C with demand flow | Volume | IMV | Dual | Dual | VC-IMVd,d |
Volume SIMV with demand flow | Volume | IMV | Dual | Set point | VC-IMVd,s |
Volume SIMV with demand flow and artificial airway compensation | Pressure | IMV | Dual | Set point/servo | VC-IMVd,sr |
Pressure A/C | Pressure | CMV | Set point | N/A | PC-CMVs |
Time-cycled pressure-limited A/C | Pressure | CMV | Set point | N/A | PC-CMVs |
Pressure A/C with machine volume | Pressure | CMV | Dual | N/A | PC-CMVd |
Pressure A/C with volume guarantee | Pressure | CMV | Adaptive | N/A | PC-CMVa |
Time-cycled pressure-limited A/C with volume guarantee | Pressure | CMV | Adaptive | N/A | PC-CMVa |
Volume A/C with Vsync | Pressure | CMV | Adaptive | N/A | PC-CMVa |
Regulated volume control A/C | Pressure | CMV | Adaptive | N/A | PC-CMVa |
Pressure A/C with flow cycle | Pressure | IMV | Set point | Set point | PC-IMVs,s |
Pressure A/C with flow cycle and artificial airway compensation | Pressure | IMV | Set point | Set point/servo | PC-IMVs,sr |
Pressure SIMV | Pressure | IMV | Set point | Set point | PC-IMVs,s |
Pressure SIMV with artificial airway compensation | Pressure | IMV | Set point | Set point/servo | PC-IMVs,sr |
CPAP/pressure support ventilation with volume limit | Pressure | IMV | Set point | Set point | PC-IMVs,s |
CPAP/pressure support ventilation with volume limit and artificial airway compensation | Pressure | IMV | Set point | Set point/servo | PC-IMVs,sr |
Infant nasal IMV | Pressure | IMV | Set point | Set point | PC-IMVs,s |
Infant nasal IMV with artificial airway compensation | Pressure | IMV | Set point | Set point/servo | PC-IMVs,sr |
Airway pressure release ventilation/biphasic | Pressure | IMV | Set point | Set point | PC-IMVs,s |
Time-cycled pressure-limited A/C with flow cycle | Pressure | IMV | Set point | Set point | PC-IMVs,s |
Time-cycled pressure-limited SIMV with artificial airway compensation | Pressure | IMV | Set point | Set point/servo | PC-IMVs,sr |
Time-cycled pressure-limited SIMV | Pressure | IMV | Dual | Set point | PC-IMVs,s |
Pressure SIMV with volume guarantee | Pressure | IMV | Adaptive | Set point | PC-IMVa,s |
Pressure SIMV with volume guarantee and artificial airway compensation | Pressure | IMV | Adaptive | Set point/servo | PC-IMVa,sr |
Time-cycled pressure-limited A/C with flow cycle and volume guarantee | Pressure | IMV | Adaptive | Set point | PC-IMVa,s |
Time-cycled pressure-limited SIMV with volume guarantee | Pressure | IMV | Adaptive | Set point | PC-IMVa,s |
Time-cycled pressure-limited SIMV with volume guarantee and artificial airway compensation | Pressure | IMV | Adaptive | Set point/servo | PC-IMVa,sr |
Volume A/C with Vsync and flow cycle | Pressure | IMV | Adaptive | Set point | PC-IMVa,s |
Volume SIMV with Vsync | Pressure | IMV | Adaptive | Set point | PC-IMVa,s |
Volume SIMV with Vsync and artificial airway compensation | Pressure | IMV | Adaptive | Set point/servo | PC-IMVa,sr |
Pressure-regulated volume control A/C with flow cycle | Pressure | IMV | Adaptive | Adaptive | PC-IMVa,s |
Pressure-regulated volume control SIMV with flow cycle | Pressure | IMV | Adaptive | Set point | PC-IMVa,a |
Pressure-regulated volume control SIMV | Pressure | IMV | Adaptive | Set point | PC-IMVa,s |
Pressure-regulated volume control SIMV with artificial airway compensation | Pressure | IMV | Adaptive | Set point/servo | PC-IMVas,s |
Time-cycled pressure-limited A/C with flow cycle, volume guarantee, and artificial airway compensation | Pressure | IMV | Adaptive/servo | Set point/servo | PC-IMVar,sr |
Volume A/C with Vsync, flow cycle, and artificial airway compensation | Pressure | IMV | Adaptive/servo | Set point/servo | PC-IMVar,sr |
Pressure-regulated volume control A/C with flow cycle and artificial airway compensation | Pressure | IMV | Adaptive/servo | Set point/servo | PC-IMVas,as |
Pressure-regulated volume control SIMV with flow cycle and artificial airway compensation | Pressure | IMV | Adaptive/servo | Set point/servo | PC-IMVar,sr |
Time-cycled pressure-limited A/C with flow cycle and artificial airway compensation | Pressure | IMV | Set point/servo | Set point/servo | PC-IMVsr,sr |
CPAP/pressure support ventilation | Pressure | CSV | Set point | N/A | PC-CSVs |
CPAP/pressure support ventilation and artificial airway compensation | Pressure | CSV | Set point/servo | N/A | PC-CSVsr |
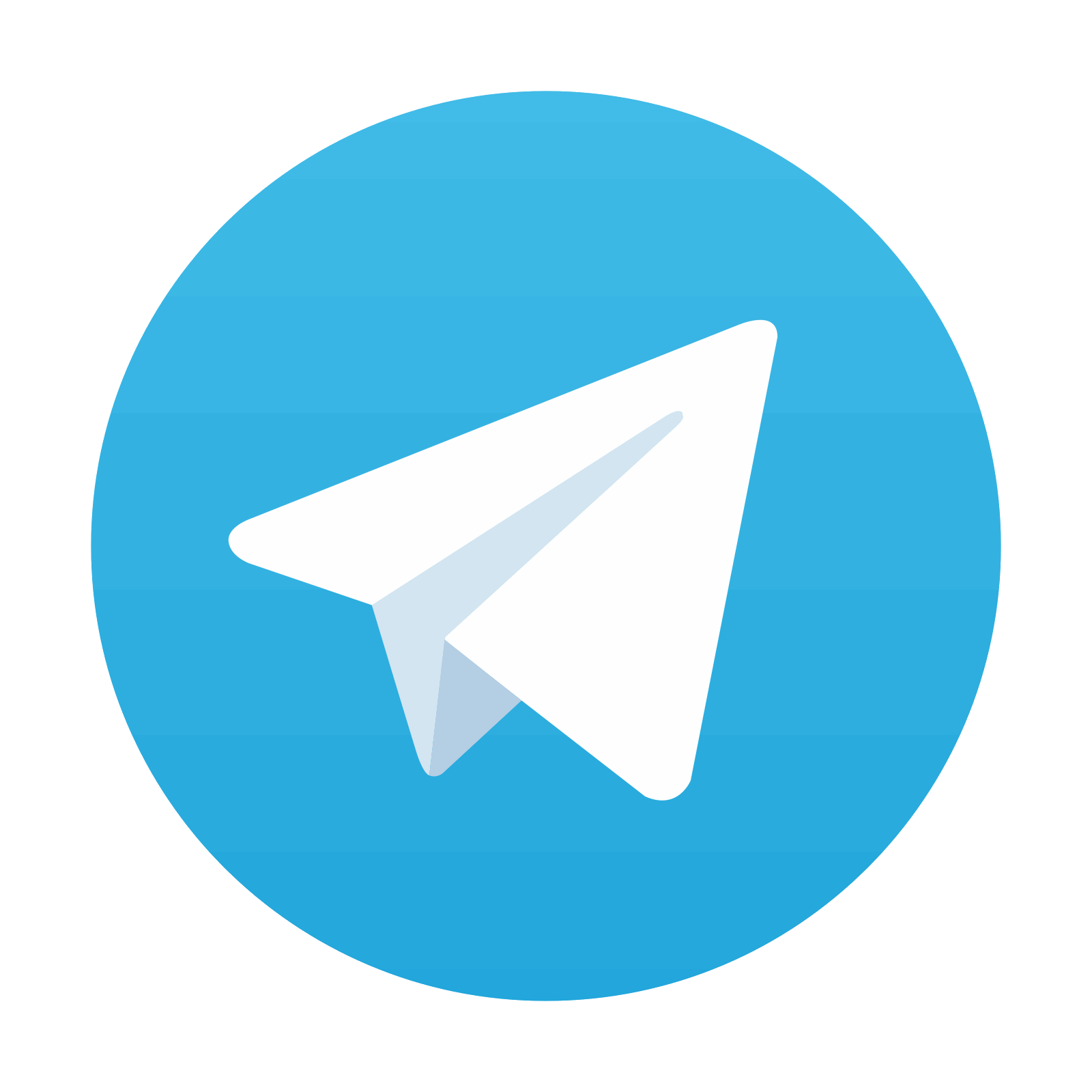
Stay updated, free articles. Join our Telegram channel

Full access? Get Clinical Tree
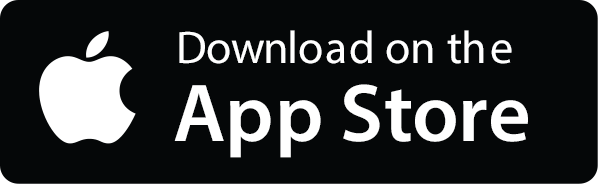
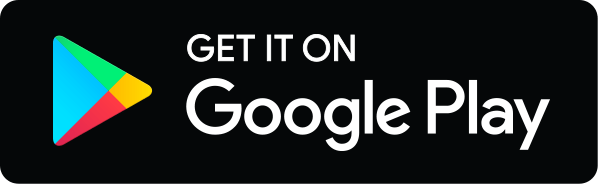
