What Are Structural Chromosome Rearrangements and How Frequently Are They Seen in the General Population?
As part of cytogenetic testing, the structure of each chromosome is assessed for (1) evidence of losses or gains of material; (2) internal rearrangements within the chromosomes; (3) exchange of material between members of the same chromosome pair; or (4) exchange of material between chromosomes of different pairs. Rearrangements are described based on microscopic examination of the chromosomes and the internal banding pattern within the rearranged chromosome(s).
Depending on the population studied and methods used, structural rearrangements of chromosomes are observed in 1:200–1:500 individuals. Unexpected rearrangements on prenatal chromosome tests occur at the higher end of this rate estimate.
How Are Structural Chromosome Rearrangements Categorized as Balanced or Unbalanced?
G-banded chromosome analysis is used to determine whether structural rearrangements appear balanced, when all of the genetic material is retained, even though a portion of chromosome material may be aligned in a different sequence within that chromosome or may be moved to another chromosome. The determination that a structural rearrangement is balanced is based on high-resolution banding and implies that on clinical evaluation the person with the rearrangement does not have dysmorphic features, structural malformations, or developmental delays consistent with aneuploidy.
Structural rearrangements may occur within a single chromosome, for example, a balanced inversion, or between members of two or more different chromosomes, for example, a balanced translocation. In contrast, unbalanced rearrangements are defined as having a loss or gain of a microscopically visible chromosome segment. Loss or gain of segments smaller than 5–10 Mb requires detection by other methods, particularly chromosomal microarray. Unbalanced rearrangements can occur within a single chromosome, for example, a terminal deletion at or near the tip of one arm of the chromosome or between members of different chromosome pairs in which there are both deleted and duplicated materials as can occur in unbalanced reciprocal translocations. Chromosomal microarray (CMA) offers more precision in delineation of unbalanced structural rearrangements and often enables detection of more complex rearrangements than is evident from traditional cytogenetic testing with high-resolution G-bands.
What Are De Novo Structural Rearrangements?
Balanced structural chromosome changes may be identified as de novo when they are not inherited from a parent. These occur due to breakage of chromosome(s) at one or more locations followed by rejoining of broken ends such that the alignment on the microscopic examination of the chromosome(s) is different than the usual sequence of bands. When portions of chromosome material are lost in the process, the structural rearrangement is unbalanced. It has been estimated that de novo rearrangements occur in approximately 1–2 per 1000 prenatal chromosome tests. Even when they appear balanced, further evaluation by microarray should be performed to identify small imbalances that may not be evident by G-banded karyotype. De novo structural rearrangements that appear to be balanced are sometimes found by microarray to be more complex than is evident by standard cytogenetics and may harbor clinically significant loss or gain of chromosome material or may result in disruption of a gene or regulatory region. Disruption of a gene or regulatory region may be detected by sequencing in some cases.
What Types of Structural Rearrangements Involve Only a Single Chromosome?
Terminal deletions of chromosomes may occur due to a single break in the chromosome with loss of the acentric fragment that is no longer attached to the centromere in subsequent cell division. Terminal deletions or duplications of the end of a chromosome may be recognizable cytogenetically because in addition to a change in banding pattern, they may result in a change in the ratio of the length of the short and long arms of the chromosome. Generally, small deletions are more compatible with survival. Examples of deletion syndromes that have been described based on cytogenetic detection are Wolf-Hirschhorn syndrome (4p deletion) and cri-du-chat syndrome (5p deletion). Cytogenetic diagnosis of deletions is infrequent when compared with detection by microarray.
What Are Ring Chromosomes?
Chromosomes with simultaneous breaks in the short arm and long arm may assume the configuration of a ring upon reunion of the broken ends ( Fig. 5.1 ). The diagnosis of a ring chromosome implies deletion of the acentric fragments that result from the breakage, and the ring typically includes both variable size segments of the terminal short-arm and long-arm material of that chromosome. Rings of the same chromosome present with a wide range of phenotypic findings. During mitosis, rings may be lost, duplicate, contract, or form duplicated interlocking rings. Mosaicism for a ring combined with variations in constitution of the ring in different percentages of cells make prediction of the phenotype difficult when a ring is found on prenatal cytogenetic diagnosis. Prenatal counseling is dependent not only on review of the literature but also on anatomic findings by imaging with ultrasound and/or MRI. Ring chromosomes initially diagnosed by prenatal microarray often result in highly unusual findings that may be best resolved by confirmatory cytogenetic visualization of the ring and the variant forms observed. Rings involve one member of a chromosome pair and are due to de novo events. They are not likely to recur except in the unusual event of gonadal mosaicism for the ring chromosome in one of the parents.

What Are Isochromosomes?
Isochromosomes are chromosomes composed of mirror images of one of the arms of the chromosome. As a result, the opposite chromosome arm may be deleted and the cells only have a single copy of the genetic material in the arm present in the normal member of the homologous pair. The other homolog has both the normal short arm and normal long arm ( Fig. 5.2 ). As a result, the cells with an isochromosome have trisomy for the arm that is duplicated in the isochromosome and monosomy for the arm that is not present in the isochromosome. Several mechanisms have been postulated for the formation of isochromosomes, including misdivision of the centromere or sister chromatid breakage and reunion in an area adjacent to the centromere. Many isochromosomes have two centromeres and are dicentric.

An isochromosome of the long arm, the X chromosome, is found in 15%–18% of Turner syndrome cases, and the phenotype is due to having only a single copy of Xp (on the other, normal X). Isochromosomes of Yp are usually dicentric and loss of this structurally abnormal Y may result in mosaicism for a 45,X cell line (see page 34, chapter 4 ).
Unlike the isochromosome of the long arm of the X and the isodicentric Y, other isochromosomes encountered in prenatal diagnosis are often small supernumerary derivatives that are mosaic. Several of these were initially categorized as marker chromosomes until the source of the genetic material was identified and a syndrome described. Isochromosomes that may be encountered in prenatal diagnosis include (1) cat eye syndrome (CES) due to an isochromosome consisting of a fusion of the short-arm centromeres and proximal long arm of chromosome 22; (2) tetrasomy 15q due to an isochromosome consisting of the short arms, centromeres, and proximal long arm of 15q; (3) Pallister-Killian syndrome that is typically due to mosaicism for supernumerary isochromosome of 12p (tetrasomy 12p). Associated diaphragmatic hernia often leads to diagnosis by amniocentesis when the fetus has Pallister-Killian syndrome. An interesting feature of Pallister-Killian is tissue-limited mosaicism in which peripheral blood often does not demonstrate the finding by chromosome analysis.
What Are Paracentric Inversions ?
Two types of chromosome inversions are seen with very different effects on reproductive potential. Paracentric inversions may occur when there are two breaks on the same chromosome arm (i.e., on one side or the other of the centromere) and reunion occurs after the segment between the breaks flips 180 degrees ( Fig. 5.3A ). Balanced paracentric inversions may be inherited by familial transmission, and the only phenotypic effect is diminished reproductive potential. Depending on the size of the inversion, it is difficult for the inverted segment to align and recombine with the noninverted homolog during meiosis. If there are an even number of crossovers on the inverted segment during meiosis, a conception will have a 50% chance of inheriting either the balanced paracentric inversion or the normal homolog that is not inverted. If an odd number (e.g., 1,3,5) of crossovers occur in the inverted segment, daughter cells will inherit either an acentric chromosome derivative that will be lost in subsequent cell divisions or a large dicentric derivative chromosome with deletion of material ( Fig. 5.3B ). In both instances, participation in fertilization would expect to result in failure of early development or fetal loss.

What Are Pericentric Inversions?
Pericentric inversions are more common than paracentric inversions and occur when the breaks are on opposite arms or sides of the centromere ( Fig. 5.3A ). Pairing during meiosis requires the formation of an inversion loop. Similar to paracentric inversions, an even number of exchanges or crossovers in the inversion loop will result in normal gametes, with one-half inheriting the balanced pericentric inversion. In contrast, an odd number of crossovers will result in aneuploid gametes, which will have trisomy for the distal portion of the short arm and monosomy for the distal part of the long arm ( Fig. 5.3C ), or the derivative chromosomes can have monosomy of the distal portion of the short arm and trisomy of the distal part of the long arm. The combination of a monosomic region and a trisomic region might not be compatible with live birth unless the monosomy is small. The smaller the inverted segment, the larger the partial trisomy and partial monosomy and the higher the likelihood of early spontaneous loss. Thus, large pericentric inversions have a greater chance of resulting in the birth of a child with aneuploidy, whereas some small pericentric inversions are more likely to be associated with recurrent loss due to duplication and/or deletion of large chromosome segments.
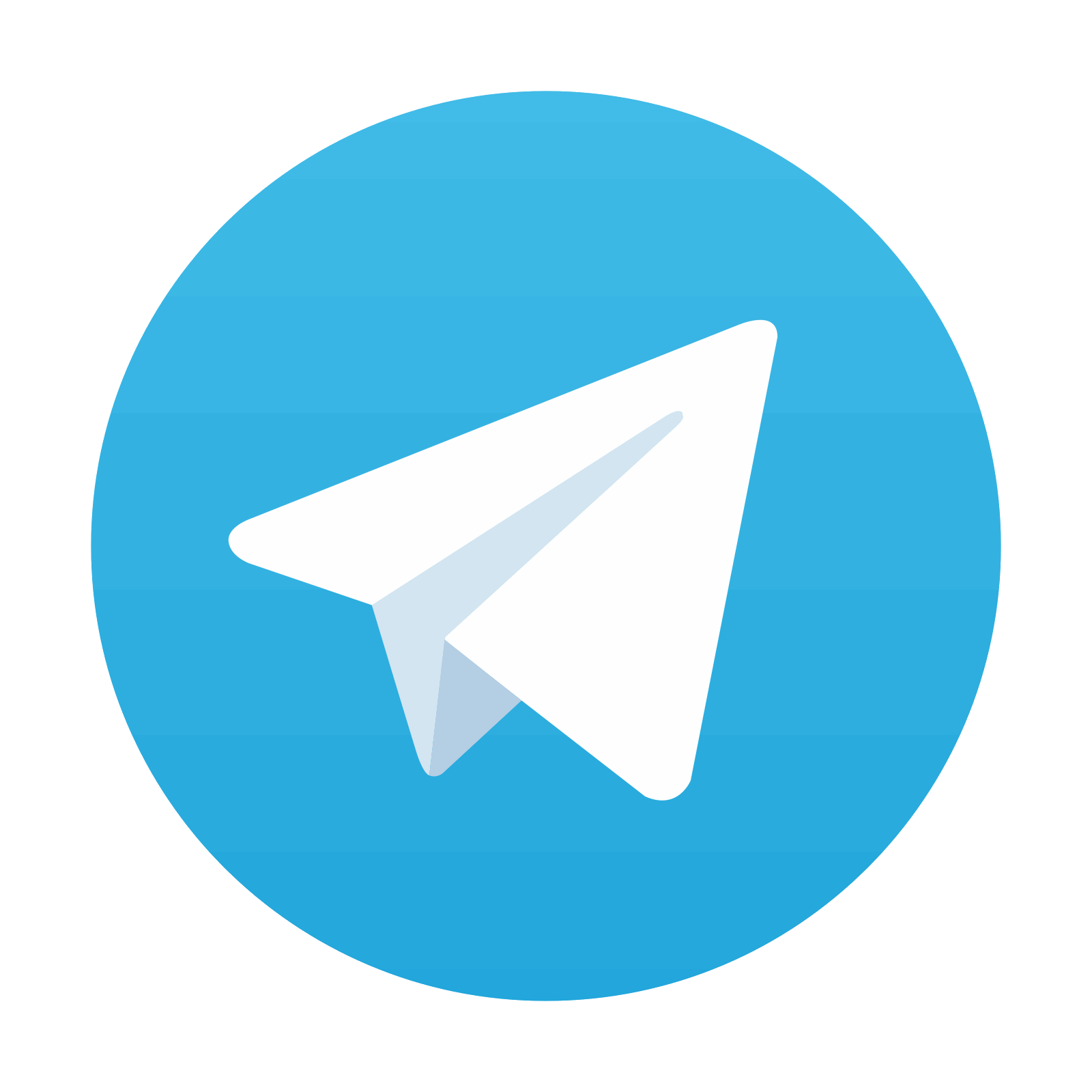
Stay updated, free articles. Join our Telegram channel

Full access? Get Clinical Tree
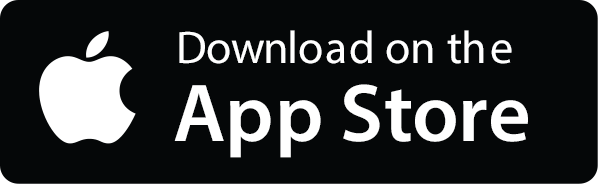
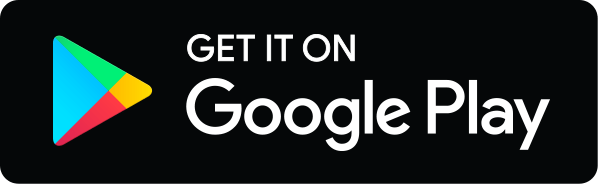