Congenital Diaphragmatic Hernia and Eventration
The management and treatment of congenital diaphragmatic hernia (CDH) remains a challenge. Despite advances in prenatal diagnosis, operative management, and neonatal critical care, infants born with CDH still have a significant mortality and long-term disability. The relatively rare incidence of the disease and wide spectrum of disease severity results in clinical challenges for individual practitioners and institutions. The large majority of centers will treat less than 10 CDH infants per year.1 Thus, broad clinical experience, especially in cases of the most severe disease, is difficult to achieve.
The morbidity and mortality associated with CDH is largely due to pulmonary hypoplasia and pulmonary vascular hypertension (PHTN).2,3 Over the last 25 years, clinical efforts to avoid lung injury, including extracorporeal membrane oxygenation (ECMO), high-frequency oscillating ventilation (HFOV), and permissive hypercapnia, have improved overall survival, but significant variation remains amongst institutions.2,4 Reported survival ranges from 50–90%.3,5
Epidemiology
The incidence of CDH has been reported as high as 1 in 2000 births.6 In the USA, approximately 1,000 CDH infants are born with a prevalence of 2.4 per 10,000 live births.6,7 One-third of infants with CDH die as stillbirths, often associated with other congenital anomalies.8 However, the overall incidence of CDH may be underestimated. Proportions of fetuses are prenatally diagnosed with CDH and will die in utero or shortly after birth. Thus, many may never been seen or accounted for in a tertiary referral center. Presumed to be the most severe of all CDH infants, these patients contribute to the ‘hidden mortality’ of CDH.9–12
Infants with isolated CDH are typically male and one-third are associated with a major congenital anomaly.7 Approximately 80% are left sided. Bilateral defects are rare and are associated with other major anomalies.13 Although the exact etiology remains unknown, mothers that are thin or underweight may have an increased risk of bearing an infant with CDH.14
Genetics
CDH is no longer thought of as a sporadic isolated anomaly in all cases. A first-degree relative has an expected occurrence rate of approximately 2%.15 All types of structural chromosomal abnormalities including deletions, translocations, and trisomies have been identified.16–21 In addition, a gene distal to the 15q21 locus has been identified for the normal development of the diaphragm.17,18
CDH has been associated with over 70 syndromes.19 In some cases, the diaphragmatic malformation is the predominant defect, as in Fryns and Donnai–Barrow syndromes.20,21 In other syndromes, CDH only occurs in a small percentage, but still greater than the general population, as in Simpson–Golabi–Behmel and Beckwith–Wiedermann syndromes. The inheritance patterns for these syndromes include dominant and recessive as well as autosomal and X-linked variants.22 Identifying the patterns of non-hernia-related anomalies associated with CDH and recognizing genetic syndromes help determine the prognosis, treatments, counseling, and outcomes.16 If the antenatal diagnosis of CDH is made, amniocentesis with karyotype and chromosomal analysis is indicated.
Associated Anomalies
The posterolateral ‘Bochdalek’ hernia accounts for 90% of all diaphragmatic hernia cases.23 The remainder are the anterior ‘Morgagni’ hernia along with defects of the central septum transversum. The majority of posterolateral CDH are left sided (85%), with right sided (13%) and bilateral (2%) accounting for the rest.23–25
Approximately 50% of CDH are isolated defects with the others associated with anomalies of the cardiovascular (27.5%), urogenital (17.7%), musculoskeletal (15.7%), and central nervous (9.8%) systems (CNS).26 Many conditions, such as lung hypoplasia, intestinal malrotation, some cardiac malformations, and patent ductus arteriosus (PDA) are considered to be consequences of the diaphragmatic defect. Non-CDH-related defects are estimated to occur in 40–60% of cases and can involve the cardiovascular, CNS, gastrointestinal, and genitourinary systems, and may be a consequence of an underlying field defect of unknown etiology.27,28
The impact of associated anomalies on prognosis and outcome cannot be overstated. Ninety-five per cent of stillborn infants with CDH have an associated major anomaly.29 Greater than 60% of infants who do not survive the immediate neonatal period have associated anomalies.30 In contrast, infants that survive preoperative stabilization and come to operative repair have less than 10% additional anomalies.30 Although the severity of pulmonary hypoplasia and hypertension are the major determinants of overall survival, there is a significant survival advantage for infants with isolated CDH (43.7% vs 7.1%).27 Due to this dismal outcome, the emphasis on detailed and accurate prenatal diagnosis has influenced the management and treatment of CDH. Twenty per cent of prenatally diagnosed CDH infants have chromosomal anomalies, with 70% having an associated structural malformation. In contrast, only 35% of postnatally diagnosed CDH infants have an associated anomaly.27 This difference may be the result of lethal chromosomal anomalies and/or may reflect parental decisions for termination in high-risk infants with anomalies that portend significant morbidity.
Common cardiac defects include atrial septal defects (ASDs), ventricular septal defects (VSDs), and other outflow tract anomalies (transposition of the great vessels, tetralogy of Fallot, double-outlet right ventricle, and aortic coarctation).30–32 In a review of 4,268 infants with CDH, approximately 18% of infants had an associated cardiac defect. Major cardiac lesions (excluding patent foramen ovale, atrial septal defects, PDA) was 8% with an overall survival of 36% compared to infants with minor anomalies (67% survival) and those without cardiac defects (73% survival).33
Cost
Despite improvements in outcomes and advances in treatment, the cost of caring for CDH infants continues to increase. Data from the Kids’ Inpatient Database has projected the annual national costs to range between $264 and $400 million based on 60% overall survival.34 The utilization of ECMO was the highest single factor associated with cost with a 2.4-fold increase in expenditures from 1997 to 2006. The highest median cost were ECMO patients at $156,499.90/patient, constituting 28.5% of the total national costs.34 Single-center reports have documented expenses as high as $250,000 per CDH case with an estimated annual cost of $230 million, and estimated cost of $98,000 for non-ECMO survivors compared with $365,000 for ECMO survivors.35
Embryology
Diaphragm development and CDH pathogenesis
The development of the human diaphragm is a complex, multicellular, multi-tissue interaction that is poorly understood. Precursors to the diaphragm begin to form during the fourth week of gestation. Historically, the diaphragm was thought to develop from the fusion of four embryonic components: anteriorly by the septum transversum, dorsolaterally by the pleuroperitoneal folds (PPF), dorsally by the crura from the esophageal mesentery, and posteriorly by the body wall mesoderm (Fig. 24-1).36,37 As the embryo begins to form, the septum transversum migrates dorsally and separates the pleuro-pericardial cavity from the peritoneal cavity. At this point, the pleural and peritoneal cavities still communicate. The septum transversum interacts with the PPF and mesodermal tissue surrounding the developing esophagus and other foregut structures, resulting in the formation of primitive diaphragmatic structures. Bound by pericardial, pleural, and peritoneal folds, the paired PPFs now separate the pleuropericardial and peritoneal cavities. Eventually, the septum transversum develops into the central tendon.36,37 As the PPF develops during the sixth week of gestation, concurrently, the pleuroperitoneal membranes close and separate the pleural and abdominal cavities by the eighth week of gestation. Typically, the right side closes before the left. Ultimately, the phrenic axons and myogenic cells destined for neuromuscularization migrate to the PPF and form the mature diaphragm.38 The musculariztion of the primitive diaphragm is a separate, but inter-related process. Historically, the primitive fetal diaphragm is thought to muscularize from the inner thoracic musculature as the diaphragm closes.39 Others have implicated a progressive development of the pleuroperitoneal membrane40 or the posthepatic mesenchymal plate (PHMP) interacting with the PPF.41
FIGURE 24-1 Historically, the diaphragm has been thought to develop from fusion of its four embryologic components. According to this theory, the septum transversum fuses posteriorly with the mediastinal mesenchyme. The pleuroperitoneal canals (arrow) allow free communication between the pleural and peritoneal cavities. Closure of these canals is completed as the pleuroperitoneal membranes develop. The four embryologic components of the developing diaphragm are shown in cross section. (From Skandalakis IJ, Colborn GL, Skandalakis JE. In: Nyhus LM, Baker RJ, Fischer JE, editors. Mastery of Surgery. 3rd ed. Boston: Little, Brown; 1996.)
Another theory for CDH development is a failure of muscularization of the future diaphragm prior to complete closure of the canal (Fig. 24-2).42 Inadequate closure of the pleuroperitoneal canal allows the abdominal viscera to enter the thoracic cavity when it returns from the extraembryonic coelom as well as the liver to herniate into the chest. Consequently, the limited intrathoracic space, due to the visceral herniation, results in pulmonary hypoplasia.
FIGURE 24-2 This schematic depicts a different embryologic pathway for diaphragmatic development and CDH formation than seen in Figure 24-1. On the left side, (1–3) is the proposed normal pathway for diaphragm development. On the right side, (4) is the pathway for CDH formation. MPC, muscle precursor cells; PPF, pleuroperitoneal fold. (From Clugston RD, Greer JJ. Diaphragm development and congenital diaphragmatic hernia. Semin Pediatr Surg 2007;16:94–100.)
Although traditional theories suggest that the lung hypoplasia is secondary to the diaphragmatic malformation, others have postulated that the primary disturbance may be abnormal lung development that causes the diaphragmatic defect.36 According to this theory, disturbances in lung bud formation subsequently impair the PHMP development, and results in failure of diaphragm fusion/muscularization.
The nitrofen murine model has led to improved understanding of abnormal pulmonary development in CDH.43 Nitrofen (2,4-dichloro-phenyl-p-nitrophenyl ether) is an environmental teratogen that is relatively harmless to adult mice. However, if given during pregnancy, it can cause pulmonary, cardiac, skeletal, and diaphragmatic abnormalities.44 Diaphragmatic defects resulting from the administration of nitrofen in mice are very similar to the diaphragmatic defects seen in babies with CDH in regards to size, location, and herniation of abdominal viscera.45,46 The side of the CDH depends on the time of nitrofen exposure during gestation. In nitrofen-exposed fetal mice, a defect is clearly seen in the posterolateral portions of the PPF.47 In addition, nitrofen exposure appears to affect muscularization of the PPF (see Fig. 24-2).48 Finally, the offspring will exhibit features of pulmonary hypoplasia including reduced airway branching, surfactant deficiency, pulmonary vascular abnormalities, and respiratory failure at birth.49,50
Other teratogens structurally similar to nitrofen have been shown to induce CDH in animal models as well. Although the exact etiology of CDH is unknown, these teratogens commonly affect the retinoic acid synthesis pathway by inhibiting retinol dehydrogenase-2 and causing similar diaphragmatic defects.51 Several clinical observations and molecular studies have supported the importance of the retinoic acid pathways in CDH development. Vitamin A-deficient rodents will produce offspring with CDH of variable severity.52 Retinoic acid receptor knockout mice produce fetuses with CDH.53 Failure to convert retinoic acid to retinaldehyde following administration of nitrofen produces posterolateral diaphragmatic defects in rats.51 Lower plasma levels of retinoic acid and retinol binding protein in infants with CDH have been found compared to controls.54
Lung Development and Pulmonary Hypoplasia
Lung development is recognized as a complex programmed event regulated by genetic signals, transcription factors, growth factors, and hormones. These events control the temporal and spatial interactions between epithelium and endothelium. Early transcription signals, such as thyroid transcription factor-1 and hepatocyte nuclear factor-3β, regulate pulmonary development from the primitive foregut mesenchyme. Other pathways of pulmonary development include sonic hedgehog, transforming growth factor-β, Notch-delta pathway, and Wingless-Int.22,55 In addition, glucocorticoids, thyroid hormone, and retinoic acid have all been shown to regulate pulmonary organogenesis.50
Fetal lung development is divided into five overlapping stages.56 (1) The embryonic stage begins during the third week of gestation as a caudal diverticulum from the laryngotracheal groove. The primary lung buds and trachea form from this diverticulum by the fourth week and lobar structures are seen by the sixth week. (2) The pseudoglandular stage occurs between the fifth and 17th weeks of gestation with the formation of formal lung buds as well as the main and terminal bronchi. (3) During the canalicular stage, the pulmonary vessels, respiratory bronchioles, and alveolar ducts develop between weeks 16 and 25 weeks with the appearance of type 1 pneumocytes and type 2 pneumocyte precursors. At this stage, functional gas exchange is possible. (4) The saccular stage continues from 24 weeks to term with the maturation of alveolar sacs. Airway dimensions and surfactant synthesis capabilities continue to mature as well. (5) Finally, the alveolar stage begins after birth with a continued increase and development of functional alveoli.
Concomittantly, fetal pulmonary vascular development occurs in concordance with the associated lung development and follows the pattern of airway and alveolar maturation. A functional unit known as the acinus consists of the alveolus, alveolar ducts, and respiratory bronchioles. The pulmonary vasculature develops as these acinar units multiply and evolve during the canalicular stage. The preacinar structures consist of the trachea, major bronchi, lobar bronchi, and terminal bronchioles. The pulmonary vascular development for the preacinus is typically completed by end of the pseudoglandular stage.57–59 In theory, any impedance to normal pulmonary development will concurrently hinder pulmonary vascular development.
Pulmonary hypoplasia is characterized by a decrease in bronchial divisions, bronchioles, and alveoli. The alveoli and terminal saccules exhibit abnormal septations that impair the air–capillary interface limiting gas exchange.60 At birth, the alveoli are thick-walled with intra-alveolar septations. These immature alveoli have increased glycogen content leading to thickened secretions which further limit gas exchange. Animal models of CDH have demonstrated pulmonary hypoplasia with decreased levels of total lung DNA and protein. In addition, the pulmonary vasculature appears to be less compliant with abnormally thick-walled arterioles.61 Surfactant levels are also decreased which may result in immature functioning lungs.62 Endogenous surfactant synthesis has been found to be decreased in CDH infants that required ECMO compared with non-CDH ventilated neonates without significant lung disease.63 Interestingly, the contralateral lung also exhibits the structural abnormalities of pulmonary hypoplasia.
Pulmonary Vascular Development and Pulmonary Hypertension
Normal fetal cardiopulmonary circulation transitions to it postnatal state rapidly with a tenfold increase in pulmonary blood flow shortly after birth. Fetal pulmonary blood flow is characterized as a low-flow, high-resistance circuit due to medial and adventitial hypertrophy of the vasculature.64 Normally, the pulmonary vascular resistance quickly decreases as the distal small pulmonary arteries and arterioles remodel over the first few months of life, resulting in a low-resistance, high-flow postnatal circulation.65,66 However, this process appears to be arrested in CDH newborns, and the fetal circulation persists resulting in PHTN. In fact, the abnormal fetal pulmonary circulation in CDH fetuses appears to occur in early gestation. The pulmonary arteries exhibit a decrease in density per unit of lung parenchyma as well as an increase in muscularization that extends to the vasculature at the acinar level.67 In fetal lamb models of surgically created CDH as well as human fetuses with CDH, there is a relative decrease in lung parenchyma.68,69 This impaired lung growth and development has been speculated to be related to impaired vascular development.70 As a result, PHTN appears to develop in utero, which may cause a reduction in pulmonary artery growth, proper alveolar development, and normal lung growth.71
Utilizing fetal Doppler ultrasound (US), fetuses with CDH exhibit a decrease in the overall pulmonary blood flow and vascularity.72 The modified McGoon Index, a ratio of the combined diameter of the proximal pulmonary arteries to the descending aorta, may help predict the degree of PHTN that correlates with death. A modified McGoon Index of ≤1.3 has an 85% sensitivity and 100% specificity for mortality.73 An index <1.25 and birth weight <2755 g has been shown to result in 80% mortality, with a sensitivity of 73% and a specificity of 78%.74 The arterial adventitia and medial wall thickness are increased in postmortem CDH infants compared to controls.75,76 In addition, the adventitial thickness and area were greater in CDH stillborns and newborns when compared to live born non-CDH infants, suggesting that increased muscularization of the pulmonary vasculature occurs in utero and fails to appropriately remodel after birth.
In a retrospective study, CDH infants who developed normal pulmonary artery pressures during the first 3 weeks of life were found to have a 100% survival rate.77 In this same study, an intermediate reduction in elevated pulmonary pressures after birth were seen in 34% of infants with a 75% survival. Mortality was 100% in CDH infants that failed to normalize their pulmonary pressures despite therapy. Although contemporary outcomes for infants with pulmonary hypertension have improved, these data underscore the importance of PHTN in CDH.
Diagnosis
Prenatal Diagnosis
Due to the wide discrepancy of disease severity and potential fetal therapies, accurate and timely prenatal diagnosis of CDH is important. The differential diagnosis for CDH include other pulmonary anomalies, such as congenital pulmonary airway malformations (CPAM), bronchogenic cysts, bronchial atresia, or bronchopulmonary sequestrations, as well as mediastinal lesions, including enteric, neuroenteric, or thymic cysts. In these conditions, the normal intra-abdominal anatomy is not disturbed. In addition, diaphragmatic eventration can be misinterpreted for CDH. Although this differentiation from CDH can be difficult, this distinction is important as diaphragmatic eventration portends a much better prognosis and requires different management. Eventration are typically isolated lesions, but may be associated with pleural and/or pericardial effusions.78
The diagnosis of CDH can be made by ultrasound as early as 11 weeks’ gestation, although most are not seen until after 16 weeks (Fig. 24-3).79 Although most CDH can be detected during the second trimester, some sonographic features may not manifest until later in pregnancy.80 Approximately 50–70% of CDH are diagnosed during pregnancy.79,81 However, for some tertiary centers, 90% of CDH newborns may have been diagnosed in utero.82 Fetal ultrasound features include polyhydramnios, bowel loops within the chest, an echogenic chest mass, and/or an intrathoracic stomach. Left-sided CDH typically feature mediastinal/cardiac shift to the right as well as herniation of stomach, intestines, and/or spleen. The liver may herniate but its echogeneity is often similar to the lung, and may be more difficult to differentiate. In right-sided CDH, the right lobe of the liver is herniated, with a left-sided mediastinal shift.
FIGURE 24-3 Fetal ultrasound image at the level of the four-chamber heart (dotted arrow). Gastric bubble (solid arrow) at the level of the four-chamber heart suggests CDH. This is the level used to calculate the lung-to-head ratio.
Ultrasound of the fetal chest is best performed in the axial plane. Fetal lung circumference, used to determine the lung-to-head ratio (LHR), is measured at the level of the four-chambered view of the heart (see Fig. 24-3). However, measurements of the fetal chest require an understanding of the dynamic growth of the fetal thorax which mostly occurs during the first trimester. During this time, the heart-to-thorax ratio is variable. However, after the first trimester, the heart-to-thorax ratio is generally 1 : 3 and remains unchanged thereafter.83 The fetal diaphragm can be seen as early as the first trimester as a thin hypoechogenic line in the sagittal view.
Although independent sonographic features of CDH have not been shown to be accurate predictors for a poor prognosis, severe or advanced CDH may exhibit an intrathoracic ‘liver up’ (liver in the chest), mediastinal shift to the contralateral thoracic cavity, or features consistent with hydrops fetalis.84 Two distinct ultrasound features have been commonly utilized to risk stratify: (1) LHR and (2) liver herniation into the chest. Predicting survival of CDH fetuses based on LHR has been statistically supported: 100% survival with LHR > 1.35, 61% with LHR between 1.35–0.6, and no survival with LHR < 0.6 in one series.85 Survival based on liver herniation alone is 56%, compared to 100% survival without liver herniation.86 The combination of liver herniation and low LHR (LHR < 1.0) has a 60% mortality in prenatally diagnosed CDH.87–89 Although fetal ultrasound is the most reliable modality for prognosis, its inconsistent utilization and inter-rater variability has created variable results.90,91
Although the heart-to-thorax ratio is consistent after the first trimester, the effects of gestational age can influence the LHR. The fetal lung increases 16-fold, compared to a fourfold increase in head circumference, between 12 and 32 weeks gestational age. This gestational age differential can be ameliorated by using the LHR as a function of observed or measured to the expected or mean of the same lung (O/E LHR). The definition of severe CDH as measured by O/E LHR is <25%. In one series, survival for severe CDH using this definition was approximately 10% with liver up and 25% with liver down. There were no survivors when O/E LHR was <15% with liver up.92 The total fetal lung volume (TFLV) as determined by three-dimensional ultrasound may be more accurate than O/E LHR as it includes the contralateral lung.93–95 Similar to the LHR, the effects of gestational age on fetal lung growth can be accounted for by using biometric measurements, total fetal body volume, or normal/expected total fetal lung volume, expressed as O/E TFLV.96–98 Early work suggests that O/E TLFV in utero correlates well with outcome.99,100
Fetal magnetic resonance imaging (MRI) has become widely utilized in the prenatal diagnosis of CDH (Fig. 24-4). Due to the high water content, fetal lungs exhibit high signal intensity on T2-weighted images which produces a stark contrast to the fetal chest and other organs. In addition, fetal MRI is an excellent modality for morphologic and volumetric measurements of the fetal lung. It is especially advantageous for oligohydramnios and maternal obesity. Utilizing an image thickness between 2–4 mm, the combination of T2– and T1-weighed images can help to differentiate and outline other organs, such as liver and intestine, as well as calculate MRI TFLV. The correlation between ultrasound and MRI-based measurements has been evaluated, but with limited results. The contralateral fetal lung volume (FLV) on ultrasound appears to correlate with TFLV measured by fetal MRI, irrespective of gestational age, side of defect, or liver herniation.101 One study showed that ultrasound O/E LHR correlated well with the O/E contralateral FLV and TFLV by MRI (R2 = 0.44, P < 0.001; R2 = 0.37, P < 0.001, respectively).102 When the MRI-based O/E TFLV is less than 25%, there is a significant decrease in postnatal survival.100
Clinical Presentation
Newborns with CDH typically present with respiratory distress. Clinical scenarios at birth range from immediate respiratory distress with associated low Apgar scores to an initial stable period and a delay in respiratory distress for 24 to 48 hours. Initial signs associated with respiratory distress include tachypnea, chest wall retractions, grunting, cyanosis, and pallor. On physical examination, infants will often have a scaphoid abdomen and an increased chest diameter. The point of maximal cardiac impulse is often displaced, suggesting mediastinal shift. Bowel sounds may be auscultated within the chest cavity with a decrease in breath sounds bilaterally. Chest excursion may be reduced, suggesting a lower tidal volume.
The diagnosis of CDH is typically confirmed by a chest radiograph demonstrating intestinal loops within the thorax (Fig. 24-5). The abdominal cavity may have minimal to no gas. Right-sided CDH is often more difficult to diagnosis (Fig. 24-6). Salient features, such as intestinal and gastric herniation, may not be seen. The herniated right lobe of the liver can be mistaken for a right diaphragmatic eventration. Occasionally, features of lung compression may be the only radiographic sign, which can cause confusion with CPAMs, pulmonary sequestrations, bronchopulmonary cysts, neurogenic cysts, or cystic teratomas.
FIGURE 24-5 (A) Anteroposterior chest radiograph in a neonate with a CDH demonstrating air-filled loops of bowel within the left chest. The heart and mediastinum are shifted to the right, and the hypoplastic left lung can be seen medially. (B) Postoperative radiograph demonstrating hyperexpansion of the right lung with shift of the mediastinum to the left. The edge of the severely hypoplastic left lung is again easily visualized (arrow).
Although most infants with CDH will be diagnosed within the first 24 hours of life, as many as 20% may present outside the neonatal period.103 These patients present with milder respiratory symptoms, chronic pulmonary infections, pleural effusions, pneumonias, feeding intolerance, or gastric volvulus.104 As CDH is invariably associated with abnormal intestinal rotation and fixation, some children may present with intestinal obstruction or volvulus. Occasionally, CDH may be completely asymptomatic and is only discovered incidentally.43,105 Older patients who present later in life have a much better prognosis due to milder or absent associated complications, such as pulmonary hypoplasia and hypertension.
Treatment
Prenatal Care
The prenatal diagnosis of CDH has improved with the increased use and refinement of fetal ultrasound examinations. After initial screening, an advanced ultrasound helps to determine discordant size and dates, associated anomalies (cardiovascular and neurological) as well as signs of fetal compromise (i.e., hydrops fetalis). Once diagnosed, chromosomal screening should be performed. Optimally, the mother and fetus should be referred to a tertiary perinatal center with advanced neonatal critical care capabilities, such as ECMO, inhaled nitric oxide (iNO) therapy, and oscillating ventilators.5 A prenatal diagnosis allows the mother and family to be properly informed of treatment options and outcomes.
Prenatal Glucocorticoids
In animal models, the hypoplastic lungs of CDH infants are structurally and functionally immature. Biochemical markers for lung maturity demonstrate decreased total lung DNA, total lung protein, and desaturated phosphatidylcholine in addition to a deficiency of surfactant.61 In one study, prenatal administration of glucocorticoids demonstrated a reduction in alveolar septal thickness, increased DNA synthesis, and increased total lung protein production.106,107 Initial results from small patient series seemed promising in that antenatal administration of glucocorticoids suggested improved lung function.108,109 However, a prospective randomized trial failed to demonstrate any benefit for CDH.110 At this point, no data exist on proper dosing or timing of steroid administration in the setting of CDH. As such, prenatal steroids are not currently recommended for CDH.
Resuscitation and Stabilization
After confirming the diagnosis, initial postnatal therapy is targeted at resuscitation and stabilization of the infant in cardiopulmonary distress. A rapid overall assessment is important to determine hemodynamic stability and the severity of disease. In severe cases, prompt endotracheal intubation and mechanical ventilatory support is likely to be required. A nasogastric tube should be inserted to avoid gastric and intestinal distention. Arterial and venous catheters assist in resuscitative maneuvers. Acid–base balance and oxygenation-ventilation status should be carefully monitored.
Invasive monitoring is important in accurately assessing the infant’s overall perfusion and the severity of pulmonary hypertension and hypoplasia. Umbilical venous catheters may be helpful and, if possible, should be positioned in the right atrium to measure central venous pressures. In addition, an approximation of cerebral perfusion should be available using preductal oxygen content and/or saturation via either a right radial arterial catheter or a transcutaneous saturation probe.
Targets for initial resuscitation include preductal arterial saturation (SaO2) between 85–95% with a minimal amount of positive airway pressures. In order to maintain lower peak inspiratory pressures (PIP), a moderate level of hypercarbia (PaCO2, 45–60 mmHg) is accepted without a compensatory acidosis and lactate of 3–5 mmol/L. Occasionally, higher levels of PaCO2 are tolerated as long as the pH > 7.2 is maintained. Failure to provide adequate tissue oxygenation can result in metabolic acidosis which may exacerbate the PHTN. Pulmonary vascular resistance (PVR) is increased by hypoxia and acidosis, which should be avoided or corrected. If severe ductal shunting develops, iNO can be tried, although PHTN in association with CDH is less responsive to iNO than other causes of PHTN.
Depending on the degree of PHTN and associated cardiac anomalies, hemodynamic stability can be difficult to achieve. PHTN may be exhibited by a different pre- and postductal SaO2. However, echocardiography can better characterize the degree of PHTN. Sonographic findings of PHTN include poor contractility of the right ventricle, flattening of the interventricular septum, enlarged right heart chambers, and tricuspid valve regurgitation. There may be right-to-left or bidirectional shunting across the ductus arteriosus.
Almost all infants with CDH and severe PHTN exhibit some left ventricular dysfunction. Vasopressor agents such as dopamine, dobutamine, and milrinone may be needed in hemodynamically unstable patients. These inotropic agents can augment left ventricular output and increase systemic pressures in order to ameliorate right-to-left ductal shunting.
Mechanical Ventilation
Mechanical ventilation is a critical component in the care of infants with respiratory failure secondary to CDH. However, the physiologic limits of the hypoplastic lung make mechanical ventilation a challenge. Hypoplastic lungs in CDH infants are characterized by a decreased number of airways and smaller airspaces. Also, the pulmonary vasculature exhibits decreased vascular branching as well as increased adventitia and medial wall thickness.111,112 This combination results in varying degrees of respiratory failure and PHTN. Fortunately, pulmonary and vascular development continues after birth and these pulmonary sequelae of CDH can improve.113,114 Because of this ongoing maturation, mechanical ventilation strategies have trended towards less aggressive approaches with the goal of maintaining oxygenation while limiting the risks of ventilator-induced lung injury, a major contributor to mortality.115
The optimal type of mechanical ventilation in infants with CDH is individual clinician preference, but most cases of CDH can be managed using a pressure-cycled mode. A fractional inspired oxygen (FiO2) of 1.0 is initially utilized to maintain adequate SaO2. Typically, higher respiratory rates and lower peak airway pressures (18–22 cmH2O) are employed while titrating the FiO2 to a preductal PaO2 greater than 60 mmHg, or the preductal SaO2 > 85% and a PaCO2 less than 60 mmHg. Maintaining an acceptable pH and PaCO2 are important in managing PHTN.116,117 The ventilation strategy of induced respiratory alkalosis with hyperventilation to reduce ductal shunting has been abandoned in most centers.118–121 Initial conventional mechanical ventilation settings should include pressure-limited ventilation rates between 40–60 breaths per minute with PIP < 25 cmH2O to minimize barotrauma.118,122 The initial PIP can be weaned to minimal settings, but should be done with caution. While targeting a PaCO2 < 45 mmHg, rapid reduction in mechanical support may allow transient periods of stability, but may also produce potential refractory exacerbations of PHTN. Spontaneous respirations are maintained by avoiding neuromuscular paralysis and minimal ventilation rates. This combination of spontaneous respiration and permissive hypercapnia has been a well-documented preoperative stabilization strategy in some centers with survival rates of almost 90% in patients with isolated CDH.118–120
If conventional ventilation fails to reverse hypercapnia and hypoxemia, HFOV strategies can be tried to avoid ventilatory-induced lung injury by preserving end-expiratory lung volume without overdistending the lung parenchyma. As the understanding of the CDH lungs continues to evolve, HFOV strategies have also changed. Initially used in a high-pressure, lung recruitment mode, this strategy demonstrated no benefit due to the nonrecruitable nature of these hypoplastic lungs.123,124 High-frequency strategies as a rescue therapy for infants with profound hypoxia and refractory hypercapnia on conventional ventilators have also not been successful.4,125 As the concept of preoperative stabilization became better defined, HFOV began to be used as means to avoid barotrauma early in the treatment course prior to refractory respiratory failure. In fact, some institutions have utilized HFOV as primary therapy.5,126,127 These strategies of preoperative stabilization to prevent lung injury along with delayed operation, regardless of ventilator modalities, have resulted in improved survival.5,115,126–128
In order to achieve lower peak airway pressures, HFOV should be considered when PIP exceeds 25 cmH2O with conventional ventilation. Initial HFOV settings include a mean airway pressure between 13–15 cmH2O, 10–12 Hz, amplitude between 30–50 cmH2O, and inspiration to expiration ratio of 50%. The initial PaCO2 should be maintained in the range of 40–55 mmHg and can be weaned by decreasing the amplitude. Eight rib expansion of the contralateral hemithorax can be used as a guide to achieve optimal lung expansion without overdistention.126,127 Tidal volumes are directly related to the amplitude and inversely related to frequency. As such, significant increases in tidal volume can be seen when frequencies are below 10 Hz. This can result in hyperinflation which, in turn, may adversely affect the pulmonary vasculature by impeding venous return. Constant assessment of acid-base status and end-organ perfusion is necessary as lung compliance can change before and after CDH repair.
Surfactant
Animal studies of CDH have demonstrated altered surfactant levels and composition.129,130 Experimentally, surfactant decreases PVR, improves pulmonary blood flow, and reduces ductal shunting.131 However, supporting data are not definite.62 Initial reports demonstrated surfactant administration augmented iNO delivery and improved gas exchange, leading to its empiric utilization.132 However, clinical evidence in term and preterm infants with CDH has not shown benefit.133 Van Meurs et al. suggested that surfactant therapy in term infants with CDH was associated with an increased use of ECMO, increased incidence of chronic lung disease, and higher mortality.134 Similar findings were found in preterm infants despite adjusting for Apgar scores and gestational age.133 Even as a rescue therapy in CDH infants on ECMO, surfactant failed to demonstrate a survival advantage.135,136 Despite the lack of proven efficacy, exogenous surfactant therapy continues to be used with unclear risks and benefits. Proponents of this therapy argue that the clinical evidence is based on a heterogeneous population of disease severity, and that the lack of clinical evidence to support its efficacy is due to its use in the most severe CDH infants. Given the current clinical data, surfactant therapy should only be used in the setting of clinical research.
Pulmonary Vasodilators
PHTN is a common consequence in CDH infants. Even if asymptomatic, the initial management in the CDH newborn is directed at reducing PVR. With increased PVR, patients often display super-systemic pressures leading to right-to-left shunting in both the pre- and postductal circulations. This can cause right heart failure with increases in preductal desaturation, narrowing of the pre- and postductal SaO2, and, ultimately, systemic hypotension and decreased perfusion. In previously stable neonates, signs of poor perfusion may indicate closure of a PDA. In response, prostaglandin E1 (PGE1) can be instituted to re-open the ductus and improve right ventricle (RV) pressures.122 In infants that demonstrate significant PHTN and RV dysfunction, left ventricular filling/function and overall perfusion may improve by off-loading the RV to improve the geometry of the interventricular septum.137 Preductal SaO2 > 85% should be maintained to ensure adequate cerebral perfusion.118 As the RV is unloaded, systolic blood pressure may decrease, especially in the setting of left ventricular dysfunction. Adequate preload should be maintained with volume loading or vasopressor support, such as epinephrine, to ensure ventricular function and coronary artery perfusion. Increasing the systemic pressure may decrease the degree of right-to-left shunting. In a small study, CDH infants with persistent PHTN and right-to-left shunting through the ductus, despite PGE1 use, had an 80% mortality.138
iNO is a potent pulmonary vasodilator that has been shown to have tremendous benefit in the treatment of persistent pulmonary hypertension of the neonate (PPHN).4,139–142 Typically, iNO is utilized in the setting of echocardiographic evidence of right-to-left shunting and RV pressures greater than two-thirds systemic pressures. In clinical studies, iNO has been shown to improve oxygenation and decrease the need for ECMO in infants with respiratory failure secondary to PPHN.142,143 However, the efficacy of iNO as a rescue therapy for PHTN secondary to CDH by either decreasing the need for ECMO or a reduction in mortality has not been supported.144 In the Neonatal Inhaled Nitric Oxide Study Group trial, the CDH subgroup had a greater likelihood for ECMO or death.145 The response to iNO is variable and unpredictable in infants with CDH. The initial improvement in oxygenation in CDH infants suggest that iNO could be utilized as a bridge for transport or until ECMO can be initiated.122 Some infants may demonstrate a rebound PHTN that is more difficult to control than the initial disease.146,147 Also, the effect appears to be transient and does not obviate the need for ECMO.77,148 A meta-analysis of iNO utilization for persistent PHTN did not show benefit in CDH infants, and recommended its use only in hypoxic respiratory failure infants without CDH.149
Phosphodiesterase (PDE) inhibitors are cyclic guanosine monophosphate (cGMP) modulators that target vascular remodeling by limiting smooth muscle cell prolifeation.150 Type 5 PDEs (dipyridamole and sildenafil) are the most active on visceral and vascular smooth muscle and have been utilized with iNO for the treatment of PHTN in patients with and without CDH. In limited case reports, dipyridamole produces a transient improvement in oxygenation which may allow weaning of ventilatory support and may avoid escalation of therapy to ECMO.151–153 Sildenafil has been shown to improve oxygenation and decrease PVR when used independently or in combination with iNO.146,154,155 Sildenafil has been used in oral or intravenous forms for congenital heart disease and CDH-associated PHTN.146,148,156,157 Similar to dipyridamole, utilization of sildenafil may have benefit in improving oxygenation and avoiding ECMO in the setting of refractory iNO.146,158 However, despite its approval for use in adults, the USA Food and Drug Administration has warned against using sildenafil for pediatric pulmonary hypertension between ages 1 and 17 years due to a potential increase in mortality during long-term therapy.159 Other vasodilators have been utilized in infants with CDH, but the experience to date is limited.160–174
Extracorporeal Membrane Oxygenation
CDH accounts for approximately one-quarter of all infants requiring ECMO for respiratory failure.175–178 ECMO utilization for CDH was first introduced as a rescue therapy for infants who had severe ventilator-associated lung injury.179 Prior to the era of preoperative stabilization, ECMO was associated with only a modest improvement in survival in high-risk CDH infants.180–182 Since then, ECMO has evolved as a treatment modality to prevent ventilator-induced barotrauma.183,184 In combination with other adjunctive treatments such as HFOV, ECMO is now routinely used for preoperative stabilization.185 The strategy of stabilization with ECMO and delay in operative correction has been shown to be beneficial with a reported survival of 67% in high-risk patients.8,186
Although results are dependent on patient selection and disease severity, survival has been reported between 60–90% for CDH patients requiring ECMO.5,118,139,175,187 Without ECMO, the predicted mortality in the high-risk cohort reaches 80%.175 Despite these data, the benefits of ECMO for CDH are not universally accepted. Some authors report no survival advantage with ECMO,188,189 while others report as high as 80% survival without using ECMO.190,191
Initially, strict criteria were established as indications for ECMO use in CDH. These included an oxygenation index >40 and persistent alveolar–arterial O2 gradient >610 mmHg.177,192 Today, those criteria have eased and the most common indication for ECMO is a ‘failure to respond’ to other therapy. In efforts to maintain lung-protective ventilation, clinicians have opted for ECMO rather than escalation of positive airway pressures. Relative contraindications include significant congenital anomalies, lethal chromosomal anomalies, intracranial hemorrhage, birth weight <2 kg, and gestational age <34 weeks. See Chapter 6 for more information about ECMO.
Surgery
Timing of Operation
With an improved understanding of its pathophysiology, repair of CDH is no longer considered an emergency procedure. However, the optimal timing for repair remains unclear. Historically, early repair was thought to improve ventilation by reducing intrathoracic pressures after reduction of the herniated viscera. However, this strategy often led to urgent procedures being performed on unstable infants.193 A paradigm shift in management to delay the operative repair until the infant is stable became widely adopted in the early 1990s.121,122,194 Several studies have shown no difference in mortality rate or the need for ECMO in infants undergoing early vs late repairs,195–197 including two randomized trials of early (<12 hours) versus delayed repair (after 24 hours197 and after 96 hours195). Today, repair of CDH is usually delayed until cardiopulmonary stability is achieved, although the definition of physiologic stability remains highly variable and inconsistent amongst centers.127,194,196–200
In 1995, Wung et al. reported advantages with a delayed repair strategy for CDH.121 Comparing three eras of treatment strategies, the study contrasted an early period of emergency surgery to the most recent era of delayed repair and ‘gentle ventilation,’ where infants with CDH were managed with a lung-preserving ventilation strategy. Repair was not performed until the pre- and postductal SpO2 gradient equalized and right-to-left shunting on echocardiogram had resolved. With an average of 4.2 days after birth before operation, survival was 94% with only one patient requiring ECMO. From the same institution in 2002, 120 consecutive patients were treated with permissive hypercapnia, spontaneous respiration, and elective repair after 36 hours of life with an overall survival of 84%.118 Of note, only 13.3% of patients needed ECMO and only 7% of infants required a prosthetic patch, suggesting a relatively small proportion of infants with large diaphragmatic defects. A different group reported similar results from a center that did not offer ECMO.201 In this study, all high-risk patients (defined as assisted ventilation within two hours of life) were divided in three historical cohorts with the most recent group being managed by permissive hypercapnia, gentle ventilation, and delayed repair until hemodynamic stability was achieved (PaCO2 < 60 mmHg, PaO2 > 40 mmHg, SaO2 > 85% with a FiO2 < 50%) for at least 48 consecutive hours. Overall survival in this recent cohort was 90%. Despite the benefits of preoperative stabilization and delayed repair, the specific parameters that define hemodynamic stability and timing of operation remain unanswered.
According to data from the Congenital Diaphragmatic Hernia Registry (CDHR) and Extracorporeal Life Support Organization (ELSO) over a recent 15 year period, one-third of infants with CDH required ECMO during their initial hospitalization, during which 85% of these infants underwent early CDH repair on ECMO.202 In this study, survival was 71% with only 9% requiring operative intervention for bleeding complications following CDH repair on ECMO compared to survival of 49% and a 14.7% bleeding complication rate from the CDH and ELSO registries. The authors attributed the better outcomes after early repair on ECMO to less body wall edema and a quicker recovery following operation which led to fewer total days on ECMO (11.7 vs 13.3 days). In another study, among infants who underwent repair of a unilateral CDH, 47.6% were repaired on ECMO, 45.8% were repaired after ECMO, and only 6.6% were repaired before ECMO.203
Bleeding remains the most significant complication following CDH repair on ECMO including both surgical site bleeding and intracranial hemorrhage. These risks may be minimized with early repair on ECMO prior to the development of a coagulopathy and significant edema,177,202 as well as repair when PHTN has resolved, but prior to decannulation to allow reinstitution of ECMO if respiratory failure and/or PHTN recur.204 Although rare, recurrent PHTN can develop after repair requiring a second ECMO run.205 In addition, use of aminocaproic acid (Amicar®), a fibrinolysis inhibitor, has significantly decreased bleeding complications. One group reported no changes in intracranial hemorrhage rates, but a significant decrease in surgical site bleeding from 12% to 7% (P < 0.05) with Amicar®.176Amicar® should be used prior to operation and for two to three days after repair. Additional strategies include ECMO without heparinization, fresh ECMO circuits, minimal diaphragmatic muscular dissection, fibrin or thrombin sealants, and utilization of recombinant factor VII for established bleeding.
Operative Approach
Open repair of CDH can be performed using a thoracic or abdominal approach. Advantages to laparotomy include easier reduction of intrathoracic viscera, the ability to mobilize the posterior rim of diaphragm, easier management of intestinal rotational anomalies, and avoidance of thoracotomy-associated musculoskeletal sequelae. The vast majority of neonatal repairs for CDH are through a subcostal incision (91%).206,207 Less than 10% are performed via a thoracotomy. The intra-abdominal contents should be reduced out of the thorax with careful attention to the spleen that can be caught and lacerated on the rudimentary rim of diaphragm. A true hernia sac, which is present less than 20% of the time, should be excised.208 The thoracic and abdominal cavities should be inspected for an associated pulmonary sequestration.
Despite this ‘gold standard’ abdominal approach, the morbidity and respiratory sequelae of open CDH repair remain a concern. In addition to pulmonary hypoplasia and hypertension, respiratory compliance is significantly reduced after open repair. Mortality significantly increases when compliance decreases by >50% which can occur as a result of a tight abdominal wall closure.193,209 Careful attention should be paid to the peak airway pressures as the abdominal fascia is closed. Respiratory compromise should alert the surgeon to leave the abdomen open. This approach is more often needed in CDH infants on ECMO.210 Temporary closure can be achieved using just the skin or a prosthetic silo. Delayed closure, especially in those infants on ECMO, should be attempted after the generalized edema has resolved or the intra-abdominal domain has enlarged.211
The routine use of chest tubes after CDH repair to drain pleural fluid has been abandoned.118,121,201 One concern is that the chest tube can cause ipsi- and contralateral lung injury secondary to mediastinal shift, especially if connected to suction. The thoracic space will eventually fill with fluid, and the lung will gradually grow. Tube thoracostomy should only be used for postoperative chylothorax or pleural fluid causing hemodynamic compromise.212 If a chest tube is needed, it is positioned in the thoracic cavity prior to final closure of the diaphragm. Chest tubes should be placed to water seal rather than suction. Symptomatic pleural fluid can be treated with repeated thoracentesis. If used, chest tubes should be removed early to avoid infectious complications.
Minimally Invasive Techniques
The respiratory sequelae and other morbidity seen after open CDH repair has prompted surgeons to adopt minimally invasive surgical (MIS) approaches. Both thoracoscopic and laparoscopic repairs have been performed.207,213–216 Data from the CDHR show that laparoscopic and thoracoscopic strategies are being used worldwide, and have been utilized in 20% of centers since 1995.207
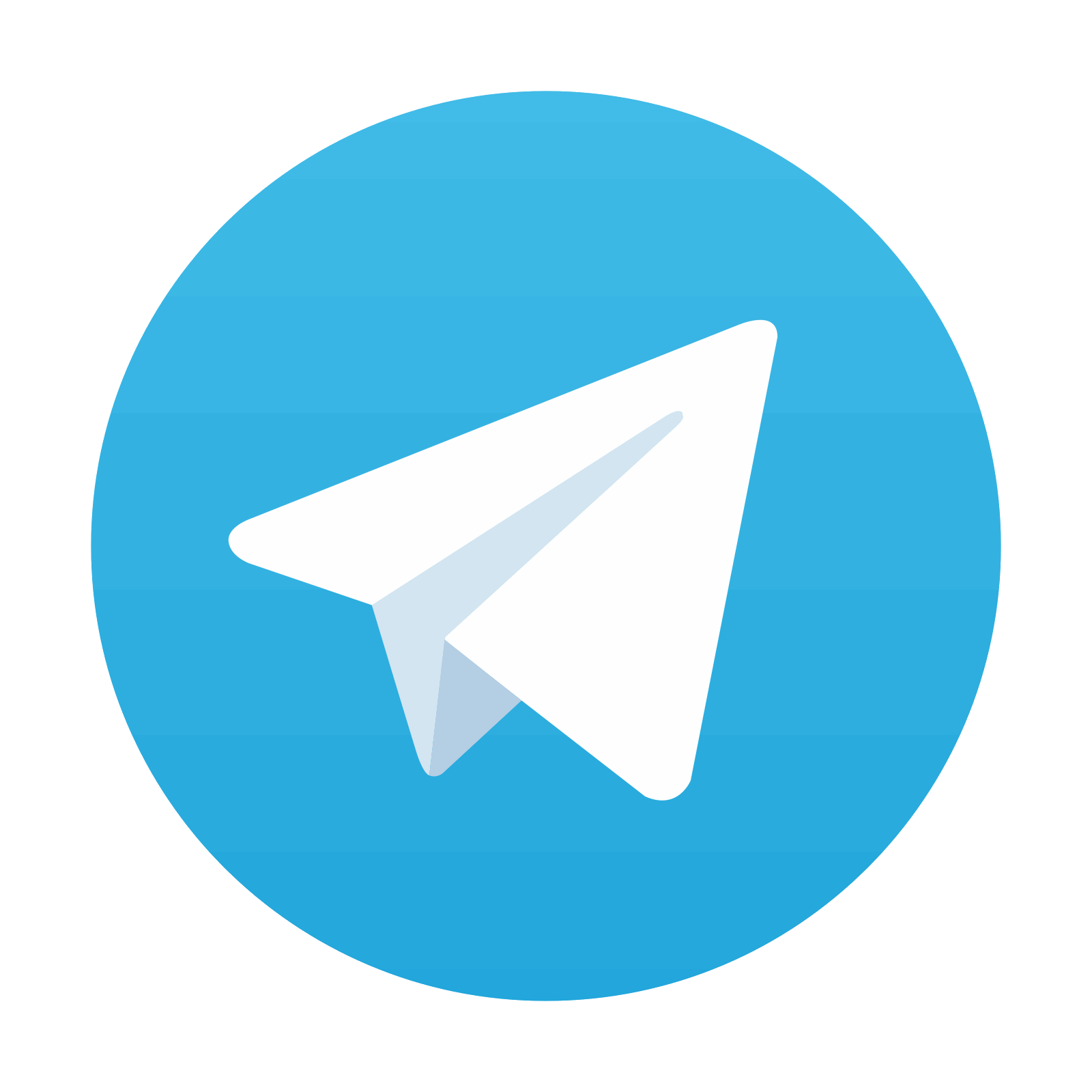
Stay updated, free articles. Join our Telegram channel

Full access? Get Clinical Tree
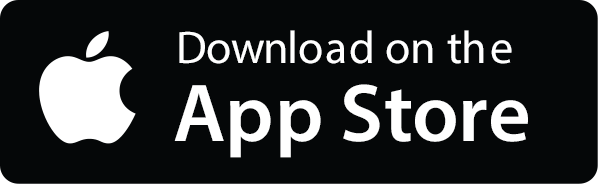
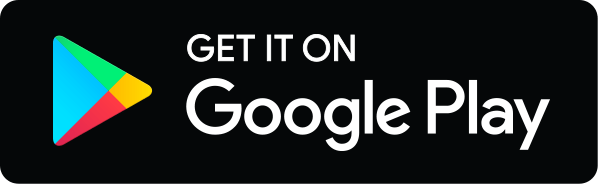