Key Points
- •
SLE and neisserial infections are the common complement deficiency phenotypes seen in Allergy Immunology practices.
- •
CH50 and AH50 assays represent logical initial studies to diagnose many complement deficiencies.
- •
Management of infection risk and autoimmunity are evolving but are critically important.
The complement system was first identified at the end of the 19th century as a serum activity that ‘complemented’ the action of antibody in the lysis of Gram-negative bacteria. During the next 100 years there was a growing appreciation that complement not only played an important role in host defense against infection but also was important in the generation of inflammation, the clearance of immune complexes and apoptotic cells, and the production of a normal humoral immune response.
Pathophysiology of Increased Susceptibility to Infection
An increased susceptibility to infection is a prominent clinical expression of most of the complement deficiency diseases. The activation of the complement system by microorganisms results in the generation of cleavage products and macromolecular complexes that possess opsonic activity (C3b), anaphylatoxic activity (C4a, C3a, and C5a), chemotactic activity (C5a) or bactericidal/bacteriolytic activity (C5b, C6, C7, C8 and C9) ( Table 10-1 ). All play a role in the host’s defense against infection. Its protective effects are critical in the generation of the initial inflammatory response to infection, prevention of spread of the infection from the initial site of infection to other areas of the body and clearance of the microorganism from the bloodstream. Furthermore, it appears to play its most important role in the early stages of infection, and the generation of opsonic activity is among its most critical functions. The opsonic function of C3b renders the pathogen more easily phagocytosed.
Components | Functions |
---|---|
C4a, C2a, C3a | Anaphylatoxins, histamine release |
C3b | Opsonin, costimulation of B cells |
C5a | Chemotaxis |
C5, C6, C7, C8, C9 | Membrane attack complex, lysis |
The nature of the infections in complement-deficient individuals generally reflects the specific roles of the deficient component in host defense. For example, C3 is responsible for generating complement-mediated opsonic activity. Thus, patients with C3 deficiency are unduly susceptible to infection from encapsulated bacteria (e.g. Streptococcus pneumoniae, Haemophilus influenzae and Neisseria meningitidis), organisms for which opsonization is a critical host defense mechanism. In contrast, patients with deficiencies of C5, C6, C7, C8 or C9 possess C3 and their susceptibility is limited to Neisseria . Bactericidal activity, mediated by C5b through C9, is critical to defense against this genus. Interestingly, although a number of Gram-negative bacteria are susceptible to the bactericidal activity of complement, the susceptibility of patients with deficiencies of C5 through C9 appears to be limited to Neisseria spp. The infections seen in complement-deficient individuals can be localized (e.g. pneumonia or sinusitis), although systemic infections (e.g. bacteremia/sepsis, meningitis or osteomyelitis) are common and often are recurrent.
A number of studies have examined the prevalence of complement deficiencies among patients with characteristic infections. Although complement-deficient patients do not appear to be sufficiently common among patients with single episodes of pneumococcal, streptococcal or H. influenzae sepsis and/or meningitis to justify routine screening of patients with these infections, complement deficiencies are sufficiently common among patients with systemic neisserial infections to make routine screening worthwhile. For example, estimates of the prevalence of complement deficiencies among patients with a single episode of meningococcal sepsis have varied from 5% to 15%. A study from South Africa demonstrated that 13% of patients with meningococcal disease had either C5 or C6 deficiency. Not unexpectedly, the prevalence is as high as 40% if the patient has had recurrent meningococcal sepsis, has an infection with an uncommon serotype or has a positive family history of meningococcal systemic infections.
Pathophysiology of Systemic Autoimmune Disorders
Systemic lupus erythematosus (SLE) is common in patients with deficiencies of C1, C4, C2 and C3. A variety of pathophysiologic mechanisms exist by which complement deficiencies can lead to the development of systemic autoimmune disorders. The two most attractive relate to the role of the complement system in the processing and clearance of immune complexes and apoptotic cells.
The complement system is a major factor in the processing and clearance of immune complexes via a variety of mechanisms. First, immune complexes carrying C3b can be ingested by phagocytic cells. Second, the activation of C3 by immune complexes maintains them as soluble complexes. Third, humans possess receptors (CR1) for cleavage products of C3 on their erythrocytes, and circulating immune complexes containing C3b can reversibly bind to those receptors. As erythrocytes carrying immune complexes pass through the liver, the immune complexes are picked off the surface by Kupffer cells, ingested and processed, thus effectively removing them from the circulation and preventing their deposition in other organs such as the kidney.
The most important mechanism driving the susceptibility to systemic autoimmune disorders is the failure to clear apoptotic cells. The early components of the classical pathway, especially C1q, participate in the clearance of apoptotic cells. As cells undergo apoptosis, intracellular constituents are reorganized and appear on the surface of the cell in blebs. Autoantigens targeted in patients with SLE are often found on the surface in these blebs, rendering a normally ‘invisible’ antigen ‘visible’. Thus patients deficient in these components may develop SLE because they lack an important mechanism of clearance of apoptotic cells. Also contributing to the development of autoantibodies directed to nuclear antigens is the potential role of complement mediating B cell tolerance in the bone marrow.
There are some clinical and laboratory features that are characteristic of SLE seen in complement-deficient individuals. For example, the SLE seen in C2-deficient patients is frequently associated with photosensitive dermatitis. It is not uncommon for C2-deficient patients to have low (or absent) titers of antinuclear antibody (ANA) or antibodies to double-stranded DNA (dsDNA), whereas the prevalence of anti-Ro antibodies in C2-deficient patients with lupus is greater than in non-C2-deficient patients. Patients deficient in C1 or C4 usually have an early onset of clinical symptoms with prominent cutaneous manifestations. SLE in C1- or C4-deficient individuals can be severe.
Pathophysiology of Atypical Hemolytic Uremic Syndrome (HUS)
Factor H deficiency has been found to be the underlying basis for 15% to 30% of patients with atypical HUS. The term atypical HUS refers to the fact that there is no antecedent diarrheal illness, which is seen in most sporadic forms of HUS. The basis for the HUS in factor H deficiency is thought to be an inability to protect fenestrated endothelium in the glomerulus from complement-mediated damage. Microtrauma arises frequently due to the high oncotic pressure and the damaged basement membrane is able to support complement activation if not protected by factor H. Interestingly, recurrent atypical HUS has been seen in patients with antibodies to factor H. Also supporting a role for factor H in the protection of basement membranes is the finding of a common polymorphism associated with macular degeneration. The central region of the retina is gradually destroyed by a process that leaves a deposit of protein, termed drusen. These deposits contain complement components. It has been hypothesized that the abnormal factor H provides less protection to the choroidal vessels, allowing smoldering complement activation and gradual damage to the endothelium. Additional complement regulatory protein deficiencies associated with atypical HUS alter the deposition of factor H or the function of the assembled complex. This activation of complement on endothelial cells is associated with microthrombi, and polymorphisms and mutations in plasminogen (which degrades thrombi) have also been found in atypical HUS.
Inherited Complement Deficiencies
Genetically determined deficiencies have been identified for most of the individual components of complement. Most are inherited as autosomal recessive traits, although one is inherited as an X-linked recessive trait (properdin deficiency), one is inherited as an autosomal dominant trait (C1 esterase deficiency), and the defects associated with HUS have variable patterns of inheritance ( Table 10-2 ). Except where noted, the mutations are diverse and can lead to either absence of protein or production of a dysfunctional protein.
Component | Inheritance | Major Clinical Expression |
---|---|---|
CLASSICAL PATHWAY | ||
C1q, C1r, C1s, C4, C2 | Autosomal recessive | SLE and bacterial infections with encapsulated organisms |
C3 | Autosomal recessive | Glomerulonephritis, severe bacterial infections HUS can be due to gain-of-function mutations |
TERMINAL COMPONENTS | ||
C5, C6, C7, C8, C9 | Autosomal recessive | Neisseria |
REGULATORY PROTEINS | ||
C1 inhibitor | Autosomal dominant | Angioedema |
Factor B | Autosomal dominant Gain-of-function | HUS |
Factor H | Variable | Infections and HUS |
Factor I | Variable | Infections and HUS |
MCP | Variable | Atypical HUS |
Properdin | X-linked recessive | Neisseria |
Factor D | Autosomal recessive | Neisseria |
C1q Deficiency
C1q is one of the three subcomponents of C1; the other two are C1r and C1s. C1q is composed of six identical subunits, each of which is composed of three different polypeptide chains, C1qA, C1qB and C1qC. IgG or IgM, after engaging antigen and forming an immune complex, binds C1q, which then activates C1r, in turn activating C1s. Activated C1s then cleaves both C4 and C2, creating the bimolecular enzyme C4b2a, which activates C3 and ultimately the terminal components (C5 through C9) via the classical pathway ( Figure 10-1 ). Thus, C1q plays a critical role in activation of the classical pathway and the generation of the biologic activities of C3 and C5 through C9. In addition, recent studies have shown that C1q recognizes apoptotic cells and targets them for clearance.

C1q-deficient individuals have markedly reduced serum total hemolytic activity and C1 functional activity. In most affected individuals, C1q protein level is also markedly reduced, but in some patients a dysfunctional immunoreactive protein is produced. There is a founder mutation in Turkey.
The most prominent clinical manifestation of C1q deficiency is SLE. C1q-deficient patients carry the highest risk (>90% prevalence) of SLE among the complement deficiencies. C1q-deficient patients have impaired clearance of the immune complexes and apoptotic cells and fail to tolerize B cells properly. The age of onset of SLE tends to be earlier, usually prepubertal, and the disease tends to be more severe. Anti-dsDNA antibodies may be negative.
C1q-deficient individuals also have an increased susceptibility to encapsulated bacteria, reflecting their inability to activate the classical pathway and efficiently generate opsonically active C3b. In fact, nearly one third of C1q-deficient patients have significant bacterial infections and 10% have died of infections. C1q, almost uniquely among complement components, is produced in substantial amounts by hematopoietic cells and a recent report documented a cure of SLE in a C1q-deficient patient who received a hematopoietic stem cell transplant.
C1r/C1s Deficiency
The C1 complex is composed of six C1q subunits and two subunits each of C1r and C1s. The genes for C1r and C1s are closely linked on chromosome 12 and encode highly homologous serine proteases. C1q, after it binds to an immune complex, activates C1r, which in turn activates C1s. It is C1s that cleaves C4 and C2, resulting in the assembly of the C3 cleaving enzyme C4b2a.
Patients with C1r deficiency have markedly reduced levels of total hemolytic complement activity and C1 functional activity. Deficiency of C1r or C1s leads to reduced levels of the other, suggesting that neither is stable if not in the heterodimer.
The most common clinical expression of C1r/s deficiency has been SLE. Some patients have also presented with glomerulonephritis or bacterial infections.
C4 Deficiency
The fourth component of complement (C4) is encoded by two closely linked genes (C4A and C4B) located within the major histocompatibility complex (MHC) on chromosome 6. Although the protein products of the two loci share most of their structure and function, there are four amino acid differences between them. The larger cleavage product of C4 is C4b which forms part of the bimolecular enzyme C4b2a that is responsible for activation of C3 and C5 through C9 via the classical pathway. It therefore plays an important role in the generation of the biologic activities of C3 and C5 through C9.
Because C4 is encoded by two distinct genes, patients with complete C4 deficiency are homozygous deficient at both loci (C4A*Q0, C4B*Q0/C4A*Q0, C4B*Q0). In contrast to the rarity of patients with complete C4 deficiency, individuals who are heterozygous for either C4A or C4B deficiency are relatively common. Approximately 13% to 14% of the population is heterozygous for C4A deficiency and 15% to 16% is heterozygous for C4B deficiency, with the corresponding frequencies for homozygous-deficient individuals being 1% and 3%. Individuals who have complete C4 deficiency (i.e. are homozygous deficient for both C4A and C4B) have little, if any, total hemolytic activity in their sera and markedly reduced levels of C4 protein and functional activity. As a result of the absence of C4, these individuals have a markedly decreased ability to generate serum opsonic, chemotactic, tolerogenic and bactericidal activities via activation of the classical pathway.
C4A deficiency is often the result of a large gene deletion or a 2-base pair (bp) insertion in exon 29. Some instances of C4B deficiency are the result of gene deletions. Finally, gene conversions can cause either C4A or C4B deficiency.
Patients with complete C4 deficiency may present with SLE and/or an increased susceptibility to infection. The onset of SLE is usually early in life and is characterized by prominent cutaneous features such as photosensitive skin rash, vasculitic skin ulcers and Raynaud’s phenomenon. Anti-dsDNA antibody may be absent. Patients with complete C4 deficiency also have an increased susceptibility to bacterial infections and most deaths in C4-deficient patients are the result of infection.
Individuals who are homozygous deficient for C4A lack the isotype that is most efficient in interacting with proteins. The prevalence of homozygous C4A deficiency in patients with SLE is thought to be increased. Interestingly, patients with SLE who have C4A deficiency have less neurologic and renal disease but more photosensitivity than other patients with SLE, and they have a lower prevalence of anticardiolipin, anti-Ro, anti-dsDNA and anti-Sm antibodies.
In contrast to individuals with homozygous C4A deficiency, homozygous C4B-deficient individuals lack the C4 isotype that interacts most efficiently with polysaccharides. There is an increased prevalence of homozygous C4B deficiency in children with bacteremia and/or bacterial meningitis.
C2 Deficiency
The second component of complement (C2) is encoded by a gene within the MHC on chromosome 6. Like C4, C2 is cleaved by C1s into two fragments, the larger of which (C2a) forms part of the C3-cleaving enzyme of the classical pathway, C4b2a. Thus C2, like C4, plays a critical role in generating the biologic activities of C3 and the terminal components C5 through C9.
C2-deficient patients usually have absent total hemolytic activity and less than 1% of the normal levels of C2 protein and function. Serum opsonic, chemotactic and bactericidal activities are usually present but not generated as quickly or to the same degree as those in individuals who possess C2.
The majority of C2-deficient individuals (>95%) have the same molecular genetic defect, a 28-bp deletion at the 3′ end of exon 6, which causes premature termination of transcription. The deletion is associated with a conserved MHC haplotype consisting of HLA-B18, C2*Q0, Bf*S, C4A*4, C4B*2, and DR*2 . This haplotype has a strong association with autoimmunity. C2 deficiency is the most common of the genetically determined complete complement deficiencies in Caucasians and the gene frequency of this deletion is between 0.05 and 0.007 in individuals of European descent, which translates into a prevalence of homozygotes of approximately 1 : 10,000.
The most common clinical manifestation of C2 deficiency is SLE. Patients with C2 deficiency manifest many of the typical clinical features of SLE, although photosensitive cutaneous lesions are more common. They also have a lower prevalence of anti-DNA antibodies than other patients with SLE, but the prevalence of anti-Ro and -La antibodies is higher. Unlike C1 and C4 deficiencies, the SLE is not necessarily more severe than typical SLE.
An increased susceptibility to infection is also a prominent clinical presentation of C2 deficiency. The infections are usually caused by encapsulated pyogenic organisms such as Pneumococcus, Streptococcus and H. influenzae and are blood-borne, such as sepsis, meningitis, arthritis and/or osteomyelitis. Infection is the leading cause of death. A recently described phenomenon in C2 deficiency that is likely common to other early component deficiencies is the accelerated atherosclerosis which significantly contributes to mortality.
C3 Deficiency
The majority of serum C3 is derived from hepatic synthesis, although synthesis by monocytes, fibroblasts, endothelial cells and epithelial cells may contribute to local tissue content of C3. Whether activated by the classical or alternative pathways, C3 is cleaved into two fragments of unequal sizes. The smaller, C3a, is an anaphylatoxin, whereas the larger, C3b, is an opsonin and also forms part of the classical and alternative pathway enzyme (C4b2a3b) that activates C5 and the membrane attack complex. Thus, C3 is not only critical in generating C3-mediated serum opsonizing and anaphylatoxic activities, but also in generating the chemotactic and bactericidal activities of C5 through C9.
Patients with C3 deficiency usually have less than 1% of the normal level of C3 in their sera. Similarly, serum opsonic, chemotactic and bactericidal activities are also markedly reduced. The mutations responsible for C3 deficiency in humans have been diverse. However, there is a relatively common 800-bp deletion found among Afrikaans-speaking South Africans (gene frequency of 0.0057).
C3-deficient patients have very severe infections. Patients tend to present in very early childhood. Although the most common infections are blood-borne infections caused by pyogenic bacteria such as Pneumococcus, H. influenzae and Meningococcus, localized infections such as pneumonia and sinusitis have also been reported.
Autoimmune diseases are also relatively common in patients with C3 deficiency. Some patients have arthralgias and vasculitic skin rashes (similar to serum sickness), associated with active infection. Most patients have membranoproliferative glomerulonephritis. True SLE is less common in C3 deficiency than in the other early component deficiencies.
The membranoproliferative glomerulonephritis in C3 deficiency is characterized by proliferation, an increase in the mesangial matrix, and electrodense deposits in both the mesangium and subendothelium of the capillary loops. Immunofluorescent studies have revealed the presence of immunoglobulins in the kidney, and circulating immune complexes may be present in the serum, suggesting that membranoproliferative glomerulonephritis in these patients is the result of immune complex deposition.
C5 Deficiency
The gene encoding the fifth component of complement (C5) is on the short arm of chromosome 9. When C5 is activated, it is cleaved into two fragments of unequal size. The smaller fragment, C5a, is a potent chemotactic fragment, and the larger, C5b, initiates assembly of the membrane attack complex, C5b through C9, and is critical for bactericidal activity ( Figure 10-1 ).
The most common clinical expression of C5 deficiency is an increased susceptibility to systemic neisserial infections.
C6 Deficiency
The genes for C6 and C7 are located near each other on the long arm of chromosome 5. C6 participates in the formation of the membrane attack complex and therefore plays a critical role in the generation of bactericidal activity. The usual form of C6 deficiency is characterized by absent total serum hemolytic activity and very low levels (<1%) of serum C6. Another form of C6 deficiency, subtotal C6 deficiency (C6SD), is characterized by 1% to 2% of the normal levels of C6 and levels of total hemolytic activity that are reduced but present.
The most common mutation causing C6 deficiency is a single base pair deletion at position 879. Interestingly, the mutations among African Americans are different from those in the African population. C6SD is the result of a loss of the splice donor site of intron 15 and results in a truncated C6 that can support some lytic activity.
C6 deficiency is one of the most common complement deficiencies. Among African Americans in the USA, it is reported to be as common as 1 : 1,600 individuals (0.062%). It is thought to be uncommon among individuals of European descent. Like other terminal components, C6 deficiency is associated with systemic neisserial infections.
C7 Deficiency
C7 participates in the formation of the membrane attack complex and therefore is critical to the generation of serum bactericidal activity. Patients who are deficient in C7 have markedly reduced serum total hemolytic activity and C7 levels. As expected, their serum bactericidal activity is similarly reduced.
Like other patients with deficiencies of the terminal components (C5, C6, C7, C8 and C9), the most prominent clinical manifestation of C7 deficiency is an increased susceptibility to systemic neisserial infections. A few patients have presented with SLE, rheumatoid arthritis, pyoderma gangrenosum and scleroderma, but it is unclear whether these are pathophysiologically related to the C7 deficiency.
C8 Deficiency
C8 is comprised of three different polypeptide chains (α, β and γ), which are encoded by separate genes (C8A, C8B and C8G). The genes C8A and C8B map to the short arm of chromosome 1, and the gene C8G maps to the long arm of chromosome 9. The alpha and gamma chains are covalently linked to form one chain (C8 α–γ), which is joined to the C8 β chain by noncovalent bonds. C8 is an integral part of the pore-forming membrane attack complex C5b-9 and, as such, plays a critical role in the generation of complement-mediated bactericidal activity.
There are two forms of C8 deficiency and each is inherited as an autosomal recessive trait. In one form, patients lack the C8 β subunit, whereas in the other form the α–γ subunit is deficient. In either form, total hemolytic activity is absent from the serum, as is functional C8 activity. However, some C8 antigen can usually be detected in C8 β deficiency because patients possess the C8 α–γ subunit. In contrast, patients with C8 α–γ deficiency usually have undetectable C8 antigen with standard immunochemical techniques. As expected, patients with either form of the deficiency have a marked reduction in serum bactericidal activity.
C8 β deficiency is more common among individuals of European descent and C8 α–γ deficiency is more common among individuals of African descent. Approximately 86% of C8 β-null alleles are the result of C-to-T transition in exon 9, which results in the generation of a premature stop codon. Only a limited number of patients with C8 α–γ deficiency have been examined, and in most instances an intronic mutation alters the splicing of exons 6 and 7 of the C8A chain and creates an insertion that generates a premature stop codon.
As in deficiencies of other components of the membrane attack complex, systemic neisserial infections have been the predominant clinical presentation of C8 deficiency.
C9 Deficiency
The gene for C9 is located on the short arm of chromosome 5. The protein product has sequence homology to other members of the membrane attack complex. Affected individuals have markedly reduced levels of both C9 antigen and functional activity. However, the hemolysis of antibody-sensitized erythrocytes can occur with the insertion of a membrane attack complex lacking C9 (i.e. C5b-8) and thus is not strictly dependent on C9. Therefore, patients with C9 deficiency have some total hemolytic activity, although it is reduced to between one third and one half of the lower limit of normal. Similarly, their sera possess some bactericidal activity, although the rate of killing is significantly reduced.
Genetically determined C9 deficiency is uncommon in general, but is relatively common among individuals of Japanese and Korean descent. A nonsense mutation in exon 4 has a gene frequency of 1 : 1,000 in Japanese populations. Individuals with C9 deficiency have an increased susceptibility to systemic neisserial infections, although the susceptibility appears to be mitigated by the residual bactericidal activity in these individuals.
Mannan Binding Lectin Deficiency
Mannan binding lectin (MBL) deficiency was initially identified in a cohort of hospitalized patients with infectious diseases. It is now known that it is quite common, with 2% to 7% of people having MBL deficiency. There are structural polymorphisms that destabilize the higher order complexes and several promoter mutations that compromise production. The mutations/polymorphisms exist in haplotypes of varying severity.
MBL deficiency has, at most, a modest effect on infection susceptibility. Similarly, it represents a modest risk factor or disease modifier in autoimmune diseases such as SLE or rheumatoid arthritis.
Mannan Binding Lectin Associated Serine Protease 2 (MASP2) Deficiency
Mutations and polymorphisms in MASP2 are common and have variable effects on function. MASP2 cleaves C4 and C2 to form the same C3 convertase as the classical pathway. The Asp105Gly variant in MASP2 leads to impaired binding to MBL. Although it was originally described in a patient with recurrent pneumonia and ulcerative colitis, subsequent studies have not identified an increased susceptibility to infection.
C1 Esterase Inhibitor Deficiency
C1 esterase inhibitor (C1-INH) is encoded by a gene on the long arm of chromosome 11. C1-INH binds covalently to C1r and C1s, leading to dissociation of the C1 macromolecular complex and inhibition of the enzymatic actions of C1r and C1s. Genetically determined C1 esterase inhibitor deficiency is inherited as an autosomal dominant trait. In the most common form (type I), accounting for approximately 85% of the patients, the sera of affected individuals are deficient in both C1-INH protein (5–30% of normal) and C1-INH function. In the other less common form (type II), a dysfunctional protein is present in normal or elevated concentrations, but the functional activity of C1-INH is markedly reduced. In either case, C4 levels are usually reduced below the lower limit of normal, both during and between attacks, because of the uncontrolled cleavage by C1s.
C1-INH is the major inhibitor of kallikrein and C1, and therefore diminished levels of C1-INH lead to unregulated activation of the classical pathway and kallikrein after exposure to a mild trigger ( Figure 10-2 ). Complement anaphylatoxins are thought to play a minor role in the process and bradykinin is the major mediator of the angioedema.

C1-INH deficiency is responsible for most cases of the clinical disorder of hereditary angioedema (HAE). The clinical symptoms of HAE are the result of submucosal or subcutaneous noninflammatory edema. The three most prominent areas of involvement are the skin, upper respiratory tract and gastrointestinal tract.
Attacks involving the subcutaneous tissue may involve an extremity, the face, or genitalia. In some instances, there may be changes immediately preceding the edema such as subtle mottling, a transient serpiginous erythema or frank erythema marginatum. The edema usually expands outward from a single site and may vary in size from a few centimeters to the involvement of a whole extremity. The lesions are characteristically nonpruritic. However, early in the development of the lesion, there may be a feeling of tightness in the skin because of the accumulation of subcutaneous fluid. Attacks usually progress for 1 to 2 days and resolve over an additional 2 to 3 days.
Attacks involving the upper respiratory tract represent a significant cause of morbidity and occasionally death. In one series published in 1976, pharyngeal edema had occurred at least once in nearly two thirds of the patients. Laryngeal edema, accompanied by hoarseness and stridor, occurs and progresses to respiratory obstruction. This is a life-threatening emergency. In fact, in that same series, tracheotomies had been performed in 1 of every 6 patients with HAE. Today, with improved treatment, this is becoming less common.
Symptoms in the gastrointestinal tract are related to edema of the bowel wall and may include anorexia, dull aching of the abdomen, vomiting and, in some cases, crampy abdominal pain. Abdominal symptoms are often prominent in childhood and can occur in the absence of concurrent cutaneous or pharyngeal involvement. In some instances, abdominal symptoms may be the only symptoms the patient has ever had, leading to difficulty in diagnosis. The abdominal pain may be so severe that it mimics an acute abdomen and some patients have suffered unnecessary exploratory laparotomies prior to their diagnosis of HAE.
Although the onset of symptoms occurs in more than half of the patients before adolescence, some patients do not experience their first symptoms until they are well into adult life. In just over half of the patients, no specific event can be clearly identified as initiating attacks, although anxiety and stress are frequently cited. Dental extractions and tonsillectomy can initiate edema of the upper airway, and cutaneous edema may follow trauma to an extremity. Some patients report attacks after the use of tight-fitting clothing or shoes, whereas others have related cold exposure to the onset of symptoms.
A potential source of diagnostic confusion is the association of HAE with SLE, presumably because the secondary reduction of C4 predisposes to SLE. These patients can be quite difficult to diagnose and manage.
Factor H Deficiency
The basis for the HUS in factor H deficiency is thought to be an inability to protect fenestrated endothelium in the glomerulus from complement-mediated damage (see ‘ Pathophysiology of atypical hemolytic uremic syndrome ’).
Neisserial infections are also seen in patients with factor H deficiency, and the mutations in patients with HUS and neisserial infections are distinct. The infections arise due to unregulated consumption of C3.
HUS is a microangiopathy typically associated with hemolytic anemia, thrombocytopenia and renal failure. Atypical HUS has a mortality rate of 25% and half of the patients develop renal failure. Factor H deficiency is responsible for 15% to 30% of patients with atypical HUS. Both autosomal recessive and heterozygous mutations have been seen. The age of onset is quite young in most cases and the disease is often recurrent. Normal complement (C3, AH50 and factor H) levels are sometimes seen and the only way in which this disorder can be confidently identified is with direct mutation analysis. A common tyrosine-to-histidine polymorphism of factor H was identified as a significant risk factor for macular degeneration in a genome-wide linkage study.
Factor I Deficiency
The gene for factor I is located on the long arm of chromosome 4. Factor I is a serine protease that cleaves C3b to produce iC3b, an inactive cleavage product that cannot function in the C3-cleaving enzyme of the alternative pathway. Patients with factor I deficiency have uncontrolled activation of C3 via the alternative pathway. Patients with factor I deficiency therefore have a secondary consumption of C3 with markedly reduced levels of C3 in their sera and a corresponding decrease in serum opsonic, bactericidal and chemotactic activity. In general, the patients with atypical HUS due to factor I deficiency have heterozygous mutations and the mutations do not localize to a specific protein domain. The mutations associated with infection and atypical HUS are distinct.
An increased susceptibility to infection is a common presentation. Like patients with C3 deficiency, factor I deficient patients have infections caused by encapsulated pyogenic bacteria such as Streptococcus, Pneumococcus, Meningococcus and H. influenzae, organisms for which C3 is an important opsonic ligand. Also like patients with C3 deficiency, some patients have had elevated levels of circulating immune complexes. In fact, there has been one report of a transient illness resembling serum sickness characterized by fever, rash, arthralgia, hematuria and proteinuria.
A second presentation is atypical HUS. Factor I deficiency is responsible for 5% to 10% of patients with atypical HUS. These patients have a phenotype indistinguishable from that of patients with factor H deficiency.
Membrane Cofactor Protein (CD46) Deficiency
Membrane cofactor protein is a widely expressed glycoprotein that inhibits complement activation on host cells. It is a cofactor for factor I-based cleavage of C3b and C4b. Deficiencies of membrane cofactor protein (MCP) are associated with atypical HUS although the presentation is usually later and milder than in patients with factor H or factor I deficiencies. MCP mutations account for approximately 10% of all atypical HUS. MCP is expressed on renal tissues and therefore renal transplantation can be successful. Traditional complement analyses are normal although the mechanism of disease is thought to be the same as for factor H and factor I deficiencies.
Properdin Deficiency
Properdin is the only gene of the complement system that is encoded on the X chromosome. Properdin stabilizes the alternative pathway C3 and C5 convertases by extending the half-lives of the C3 and C5 converting enzymes. Properdin deficiency is inherited as an X-linked recessive trait. The protein may be absent or reduced in the serum, depending on the specific mutation. Patients with properdin deficiency have absent function of the alternative pathway. Similarly, serum bactericidal activity for some strains of meningococci is reduced in properdin-deficient serum.
Approximately 50% of the patients described with properdin deficiency have had systemic meningococcal disease. Isolated cases of SLE and discoid lupus have also been seen in properdin-deficient patients, but these may represent ascertainment bias.
Factor D Deficiency
Neisserial infections are seen in factor D deficiency. Systemic streptococcal infections have also been seen. Other complement levels are typically normal in factor D deficiency, however there is almost no ability to activate the alternative pathway.
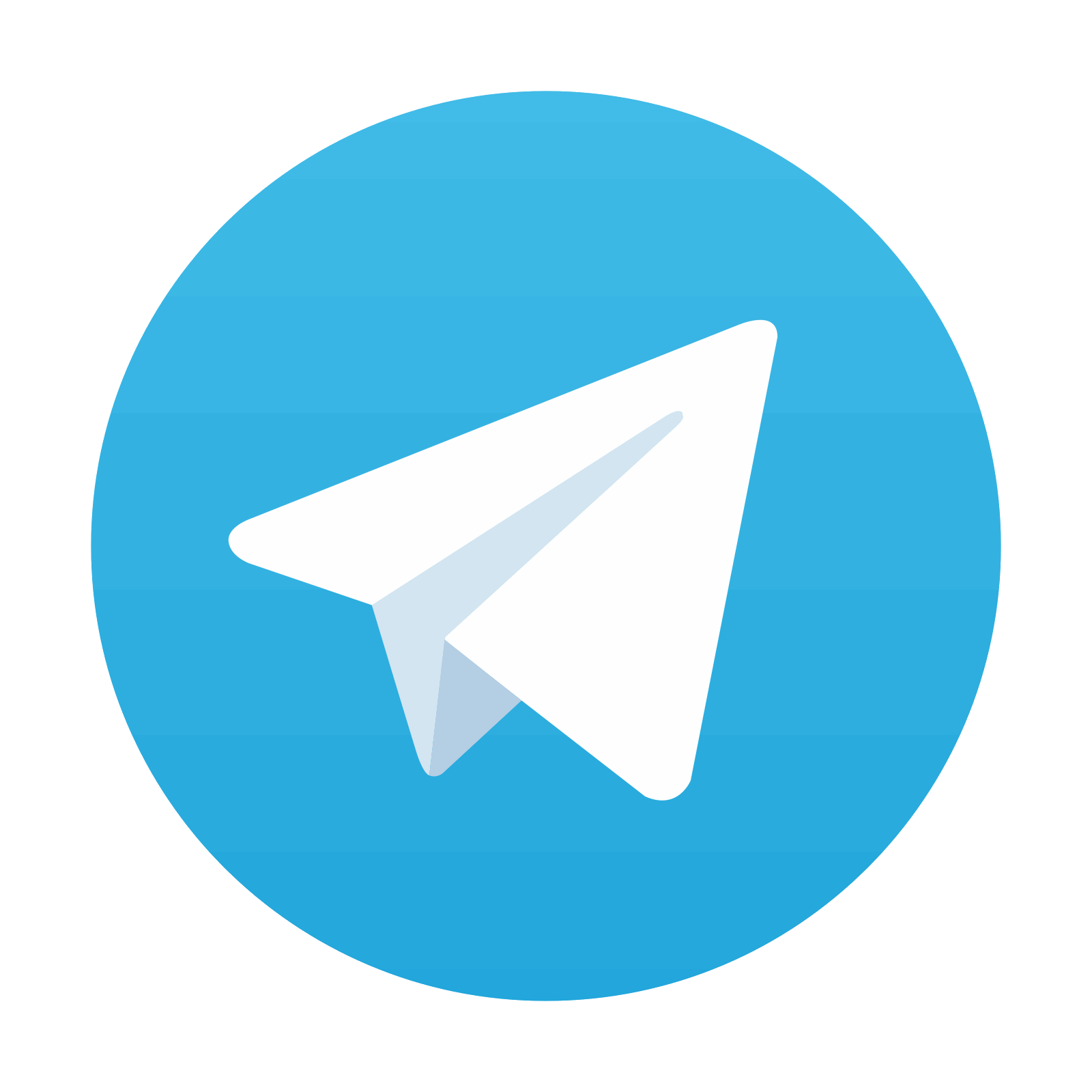
Stay updated, free articles. Join our Telegram channel

Full access? Get Clinical Tree
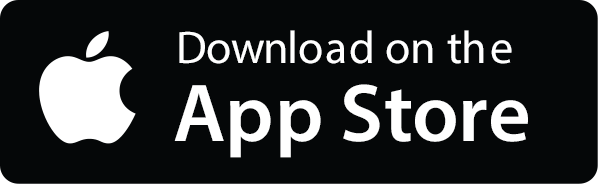
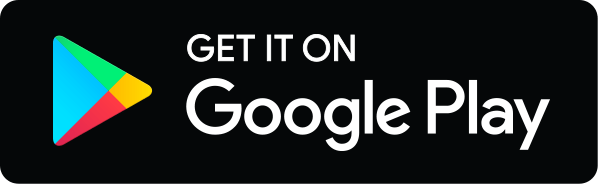