Introduction
Sleep is a behavioral state characterized by decreased motor activity and reduced interaction with the external environment with easy reversibility. It is one of the most vital primary activities of the brain during infancy and plays an important role in neurocognitive and psychosocial development in early life. Current theoretical models suggest that there are two independent but interacting regulatory processes that control the timing, intensity, and duration of sleep: circadian sleep process and homeostatic sleep process. It is also known as the two-process model of sleep regulation, which accounts for the sleep-wake patterns in humans and their alertness during the day. Although these two sleep processes are the major intrinsic biologic drivers of an individual’s sleep-wake pattern, sleep behaviors in children are also significantly influenced by the environment, cultural values, parental and social beliefs. Sleep also has its internal rhythmic organization, as known as ultradian rhythms, which includes sleep cycles of rapid eye movement (REM) sleep and non-rapid eye movement (NREM) sleep. All these physiologic processes change over the life course, especially in the first few years of life. Understanding how sleep processes evolve and the factors influencing the development is important to define the normality of sleep across early childhood and to investigate the associations between sleep and neurodevelopment. An understanding of sleep physiology and regulation is also critical for effective parent counseling and sleep interventions for children with problematic sleep or sleep disorders. This chapter will review the sleep characteristics, circadian development, and homeostatic sleep process in infancy.
Sleep and ultradian rhythms in infancy
Human sleep is composed of ultradian cycles between REM sleep and NREM sleep. In older children and adults, these two main sleep stages can be easily captured by monitoring the brain waves, eye movements, and chin muscle tone in a standard polysomnography (PSG). However, REM and NREM sleep in early infancy, also known as active sleep (AS) and quiet sleep (QS), respectively, may be difficult to be differentiated from each other based on these physiological signals because our brains in early life are not mature enough to produce the key features of REM and NREM sleep. The characteristics of REM and NREM during infancy are described in Table 2.1 . Apart from EEG, EOG, and chin EMG, additional characteristics, including breathing regularity and behavioral observations for body, limb, or facial movements, provide essential information for the differentiation between REM and NREM sleep. Therefore, an audio-video recording synchronized with standard PSG is recommended for the sleep studies in infancy. Nonetheless, there is still a substantial proportion of sleep time that cannot be definitely categorized into REM or NREM sleep, owing to the concurrent occurrence of both REM and NREM features in the same epoch. These uncertain epochs, which mostly occur during wake-sleep and REM-NREM transitions, are labeled as transitional sleep (also known as indeterminate sleep, IS). The emergence of different sleep states is one of the most significant aspects of early brain maturation. Premature infants and neonates also show distinct oscillatory features, such as trace alternant, delta brush, and temporal theta, which are age and region dependent.
Characteristics | ||||
---|---|---|---|---|
Stage | Behavioral | Respiration | EEG | EOG and Muscle Tone |
Awake |
| Irregular, rapid, and shallow |
| Eye blinks, REMs, scanning eye movements; transient eye closures may be seen in wakefulness especially when the infant is crying |
Active sleep (REM sleep) | Eyes closed, REM seen under closed eyelids, squirming, sucking, grimacing, small movements of the face or limbs | Irregular, some central pauses (may or may not meet criteria for apnea) | Low voltage, irregular or mixed frequency | Eyes closed with REMs; absence of muscle tone, frequent muscle twitches and body jerks that break through the muscle inhibition of infant REM sleep |
Quiet sleep (NREM sleep) | Eyes closed, few movements, sucking can occur | Regular | Low frequency, high voltage; trace alternant (high-voltage slow activity interrupted by near electrical silence) in 37–44 weeks post conceptional age | Eyes closed, not moving; low muscle tone |
The origin of sleep and ultradian development is found during the fetal period. As early as by 32 weeks of gestation, AS and QS could be differentiated while a large amount of time is spent in IS. Between 32 and 40 weeks postconceptional age, QS significantly increases, and IS decreases without significant change in AS. Preterm birth per se does not appear to change the time course of the sleep development, which suggests this is an endogenous brain mechanism and a measure of brain maturation.
In the first few months postterm, infants’ sleep is divided evenly between AS and QS. By the second half of the first year postterm, AS and QS gradually transition into more mature form of REM sleep and NREM sleep, respectively. When young infants fall asleep, the initial sleep episode is typically REM sleep. At 3 months postterm, sleep-onset REM periods become replaced by the adult pattern of sleep-onset NREM periods. The proportion of REM sleep also decreases during early childhood. Active/REM sleep is 50% of sleep at term, 40% at 3 to 5 months postterm, and close to the adult level of 25% to 30% of nocturnal sleep by about 1 year of age. The cyclic alternations of REM and NREM sleep throughout the night are known as ultradian rhythm. The ultradian cycle is about 45 to 60 minutes in infancy and gradually increases to 90 to 110 minutes by school age. During the first weeks of life, power spectrum analysis of electroencephalography (EEG) shows higher delta activity during QS when compared to AS. However, different stages of NREM sleep, such as stage N1, N2, and slow wave sleep (SWS), cannot be classified. Sleep spindles rapidly develop during the first 3 months postterm, likely reflecting developmental changes in thalamocortical activity. A recent study identified an independent EEG band of 10 to 16 Hz (corresponding to sleep spindle frequencies) using principal component analysis at 41 weeks postconceptional age, suggesting that sleep spindle development may start at the first week postterm. Slow waves become visible between 2 and 5 months postterm, and the development of which is the principal component for lengthening the duration of QS or NREM sleep in infancy. By 6 months postterm, the discrete electronical patterns of REM and NREM sleep progressively resemble those seen in adults. Sleep spindles (stage N2) and SWS during NREM sleep become classifiable. However, the development of REM and NREM sleep may begin before these EEG features become visually identifiable. A study using multiscale permutation entropy to quantify EEG signal complexity demonstrated that EEG during both REM and NREM sleep become more complex from 2 to 5 weeks of age, suggesting that the brain wave begins to develop in the first weeks of life.
The sleep and wakefulness of newborns are distributed equally across day and night. Subsequently, sleep becomes gradually consolidated toward the nighttime with an increase in the nocturnal sleep duration and a decrease in the daytime sleep. The development of the 24-hour rhythm in sleep-wake behavior during infancy is driven by the emergence of both circadian and homeostatic sleep pressure, as well as by daily activities such as feeding patterns. Over the first few months, the ability to retain calories increases, with a decrease in the caloric need and growth relative to size. Infants gradually become able to consume adequate calories during the day and require fewer nighttime meals. Simultaneously, the biological rhythms that contribute to sleep regulation mature, with decreased sleep fragmentation and increased sleep consolidation, making infants capable of sleeping through the night. Most infants eventually begin sleeping through the night by the age of 6 to 9 months. A consolidated sleep through the nighttime is considered a major developmental milestone and is a crucial area of infant care.
Circadian development
Circadian rhythm is a near 24-hour periodicity found in every physiological process, including sleep, in the human brain and body. It is endogenously synchronized by the circadian system, and adjusted through the influence of exogenous factors. The underlying endogenous circadian mechanisms have a distinct neuroanatomic locus in the suprachiasmatic nuclei (SCN) of the hypothalamus, known as the biologic clock, which sets the timing of rhythms by regulating neuronal activity, body temperature, and hormonal signals. This clock is synchronized by daily exogenous environmental cues known as zeitgebers, and the most powerful zeitgeber is light. Light activates photoreceptors in the retina inhibiting pineal gland secretion of the sleep-promoting hormone, melatonin. Moreover, various molecular and genetic components of the clock have been identified.
In animal studies, rhythms of metabolic activity and gene expression are evident in the SCN of rodents and nonhuman primates by mid-to-late gestation. In humans, melatonin and dopamine receptors appear as early as gestational age 18 weeks in the fetal SCN, suggesting melatonin and dopamine may serve as the primary communicators of circadian development in fetus. Melatonin from the mother readily passes through the placenta and the fetal blood-brain barrier and appears to be the predominant relay of circadian information to the fetus. The origin of circadian rhythm development has been observed since the fetal period and believed to be entrained by the mother to the light-dark cycle. A day-night rhythm of fetal heart rate synchronized with maternal rest-activity, heart rate, cortisol, melatonin, and body temperature is found in humans. , The lack of circadian rhythm in an anencephalic fetus despite the day-night rhythm in the maternal heart rate supports that fetal brain is necessary for the development of this endogenous rhythm. Fetal and early neonatal circadian rhythms are important as they prepare the infant for the later adaptation to the environmental light-dark cycle. Previous animal studies reported that exposing pregnant nonhuman primates to constant light suppresses the emergence of melatonin and body temperature rhythms in their offspring following birth. Gestational circadian disruption also promotes social avoidance and hyperactivity behaviors in mice. These findings highlight the importance of maternal circadian stability during pregnancy for the early development of fetal circadian systems.
Early childhood is a critical time period when consolidation of sleep-wake patterns over the 24-hour period occurs. Newborns (0–3 months) do not have an established circadian rhythm. Episodes of sleep and waking seem randomly distributed across day and night. Moreover, day and night reversal is common in the first few weeks after birth. In fact, infants are born with low levels of maternally transferred melatonin, which dissipates by 1 week. Endogenous melatonin does not rise to detectable levels until approximately 6 weeks after birth, and circadian rhythm emerges by 2 to 3 months of age. Melatonin levels are still very low at 12 to 16 weeks, but by 6 months they become a stable part of the sleep-wake cycle. Nighttime sleep consolidation develops between the age of 4 and 12 months, while daytime sleep decreases progressively. The number of naps commonly decreases from 2 naps to once by 18 months, with discontinuation of daytime sleep typically by the age of 5 years.
Besides sleep-wake patterns, biological rhythms integral to the circadian cycle also include changes in body temperature and circadian-driven hormones such as melatonin and cortisol. A nocturnal trough of body temperature, which is a good marker of human circadian rhythms, is already present at 6 to 12 weeks of age in term infants. Endogenous production of melatonin and cortisol also begins to cycle in a 24-hour rhythm at the first 3 months of age, although the exact timing of the development of hormonal circadian rhythm remains controversial. Kennaway et al. found no evidence of circadian rhythm in melatonin before 9 to 12 weeks of age in term infants. A delay of 2 to 3 weeks in development of melatonin circadian rhythm in preterm versus term infants was also reported. On the other hand, a study in term infants demonstrated the development of cortisol circadian rhythm by 8 to 12 weeks of age. Another longitudinal study in preterm infants (gestational age 31–34 weeks) showed circadian rhythm in salivary cortisol level in more than half of the infants at 2 to 8 weeks of life. This group as a whole developed circadian rhythm of cortisol between 8 and 12 postnatal weeks similar to term infants. A more recent longitudinal study of 130 healthy full-term infants with monthly saliva sampling demonstrated that circadian rhythm of salivary cortisol secretion is already established by one month of age. Similar findings were obtained in preterm infants in a subsequent study which showed that salivary cortisol circadian rhythm is established by one month corrected age. Despite the inconsistency shown in previous studies, it is widely agreed that the biological rhythms and their synchronization with the times of day develop rapidly over the first 6 months of life, highlighting the importance of effective behavioral routines that reinforce this development early in infancy.
The early development of circadian rhythms is based on specific maturation processes of the brain, set by both genetic and environmental factors. There are individual differences in the development of circadian rhythms. The length of circadian cycle can be affected by circadian gene variants. For the first few months after birth, nocturnal sleep onset is typically coupled with sunset and then to family bedtime thereafter, suggesting that the circadian system is initially entrained by light but is subsequently also entrained by other environmental and social factors. The factors that potentially affect the development of circadian rhythms include feeding (scheduled versus on-demand, mother’s milk versus formula milk), environmental lighting (indoor versus outdoor, regular versus irregular light-dark cycle), and chronological or postconceptional age of the infant. Therefore, the results of circadian research in human infancy are often significantly confounded by maternal and/or environmental factors as well as by the recording methods.
Whether environmental factors can influence the time course of circadian rhythm maturation is controversial. A study by McMillen et al. found that in a group of infants discharged home with a setting of regular day-night cycle, a single caregiver and on-demand feeding, circadian rhythm of sleep adaptation occurred after 6 to 10 weeks regardless of whether the infants were preterm or term. There was an inverse relationship between gestational age and the postnatal age at when the entrainment occurred in preterm infants, suggesting this postnatal delay in entrainment of the more preterm infants to be secondary to the longer period of nonentraining stimulation in the neonatal nursery environment. Regular environmental entrainment factors appear to be more important than preterm/term delivery in later adaptation of the infants to a light-dark cycle. However, in other studies, no differences were observed for the time of entrainment to day-night rhythm in infants exposed to differently lighted environments (a cycled light nursery versus continuous dim nursery; or preterm infants exposed to continuous light in the NICU versus varying light intensity at home). , The exposure of preterm infants to continuous lighting for several weeks before discharge home does not appear to retard the development of sleep-wake circadian rhythms if an appropriate lighting regime is experienced at home. Despite the controversy, however, there is no rationale that would justify a chaotic noncircadian environmental approach in the neonatal nursery. In fact, some studies demonstrate that nursing premature infants under regular light-dark schedules in the neonatal intensive care unit leads to greater weight gain when compared to constant bright light or dim light. A systematic review concluded that cycled light seems to shorten the length of hospital stay, although the evidence is hampered by the small sample size and the inability to blind the intervention. The lack of circadian rhythmicity, not only in light but also in the pattern of noise, parental care, may subject the infant’s developing circadian rhythm to conflicting temporal cues. Regular light-dark schedules support the maturation of rest-activity, sleep-wake, and melatonin rhythms earlier in premature infants. Furthermore, if the SCN is responsive as early as 28 weeks of gestation, cycled light at this time until discharge home may influence circadian organization. Continuing regular day-night rhythm at home as well as maximizing the day-night differences by minimizing nighttime caregiver intervention, including feeding, may benefit the development of preterm and term infants. The American Academy of Pediatrics also recommends that parents should start promoting good sleep hygiene, with a sleep-promoting environment and a bedtime routine in infancy, and throughout childhood, to promote health and to prevent and manage sleep problems. Larger multicenter randomized trials will be needed to determine the extent to which different light-dark schedules might improve long-term health outcomes in both preterm and term infants.
Homeostatic sleep process
Homeostatic sleep process, known as process S, represents a sleep-wake-dependent homeostatic component of sleep. It describes the body’s internal neurophysiologic drive toward either sleep or wakefulness. The homeostasis is governed by the principles of equilibrium. Process S builds up as a function of wakefulness and decreases after sleep onset. There is a neurophysiologic drive to sleep after periods of wakefulness, and similarly a drive to wake after periods of sleep. Research has indicated that the homeostatic sleep drive may be produced by sleep-promoting substances, somnogens, that accumulate during prolonged wakefulness and are depleted during sleep. , , Adenosine is widely known as one of these somnogens. It is a byproduct of biological activity in the brain from dephosphorylation of adenosine triphosphate, and therefore accumulates with activity, and then dissipates with rest and sleep. Adenosine promotes sleep by inhibiting arousal, it activates the hypothalamic ventrolateral preoptic nucleus neurons that inhibit arousal-promoting centers.
In adults, the time course of the homeostatic process is often derived from EEG slow wave activity (SWA) (EEG delta power in the frequency range 0.75–4.5 Hz) during NREM sleep. EEG delta power during NREM sleep is in a quantitative relationship with prior sleep-wake history. It increases during early NREM sleep proportionally with increasing wake duration. SWA decreases during subsequent sleep cycles in the night in adults and children. However, relevant data in infant sleep regulation and homeostasis are relatively scarce. Sleep demonstrates substantial age-related changes. During early development, both animal and human studies showed that the ultradian sleep cycle is characterized by an alternating pattern of SWS in every other sleep cycle. In human infants, it was also found that NREM sleep with high levels of low-frequency delta activity (0.75–1.75 Hz) is present in alternate NREM sleep episodes across the night, together with an age-dependent increase in the low-delta activity likely secondary to the vast increase in cortical connectivity due to increasing synaptogenesis. , This alternating pattern was most prominent at 6 months of age, and no decline in the low-frequency delta activity across the night was observed. In contrast, theta activity (6.5–9 Hz) progressively declines over consecutive NREM episodes, suggesting that theta activity may better reflect the dissipation of sleep pressure during infancy than SWA. A study found a negative correlation between the rate of process S diminishing and prior wakefulness in infants during the first year of life. However, delta latency was not correlated to prior wakefulness. Therefore, SWA alone may not be an appropriate marker for sleep homeostatic pressure in infants. The exact age at which SWA becomes the sleep homeostatic marker is not known. On the other hand, more recent studies demonstrate that slow waves, when originating from a particular location, travel across the cortex to involve more populations of cortical neurons. Changes in sleep pressure affect the alternating firing pattern of cortical neurons and the level of synchronization among populations of cortical neurons represents a cellular counterpart of the homeostatic sleep regulation. The slope of slow waves, as measured in the EEG, may be a marker of this neuronal synchronization and thus a marker of sleep pressure. Fattinger et al. demonstrated a decrease in the amplitude and the slope of slow waves from the first to the last hour of NREM sleep in infants, suggesting that the level of neuronal synchronization decreases across the night in infants. These findings support that the homeostatic sleep regulation develops early in infancy.
The dynamics of sleep homeostatic mechanisms slow down in the course of development. In infants, several studies have demonstrated that the accumulation rate of homeostatic sleep pressure with waking during the day, and its dissipation during sleep are faster than in older children and adults. , The quicker accumulation of sleep pressure in infants and younger children necessitates longer and more frequent daily daytime naps and also accounts for shorter nap sleep-onset latencies. While homeostatic sleep pressure accumulates more slowly across development, older children become able to sustain wakefulness for longer periods of time, fewer naps and shorter nap durations, and eventually a consolidated sleep-wake pattern. A more recent study also demonstrated a developmental increase in wakefulness across the vulnerable preterm period, which occupied 15% of the time by full-term age.
Interaction between circadian processes and sleep homeostasis
Circadian and sleep homeostatic processes are independent but complexly interacting regulatory mechanisms, which control vigilance during wakefulness, sleep timing, and maintenance. During wakefulness, the cumulative increase in the homeostatic sleep pressure is opposed by the increasing circadian alertness to maintain constant levels of vigilance. On the other hand, during sleep, the increasing circadian sleep tendency counteracts the declining homeostatic sleep pressure to maintain sleep. The developing interaction of the two processes likely contributes significantly to determine the sleep-wake behavior in early childhood. However, data regarding how the two processes interact in infancy are scarce. As the dynamics of sleep homeostatic mechanisms are believed to be different during infancy when compared to older children and adults, the development of a circadian-dependent alerting signal in the absence of the opposing sleep homeostatic process during the first few months of life may explain the tendency for some infants to express crying behavior preferentially at certain times of day, such as in the evening hours. Moreover, a misalignment of the two processes may be the culprit to the problems at sleep onset, nocturnal awakenings, difficulties awakening in the morning, or daytime sleepiness, which are common sleep problems during childhood. More future research will be needed in this regard to provide additional insights.
Sleep and early development
Sleep is a critical window for brain development and maturation in early human life. Evidence supports that sleep homeostasis is involved in aspects of learning and neural plasticity. REM sleep is believed to be important in brain development and growing and strengthening of new neural connections. It has been suggested that infants have proportionally more REM sleep that stimulates structural development, neural differentiation, and the development of neural pathways during the neonatal period. During the first year of life, the amount of REM sleep decreases, while NREM sleep becomes the predominant state. The topographic distribution of SWA during NREM sleep and the local SWA maxima parallel the time course of synaptic density, cortical gray matter and behavioral maturation during childhood and adolescence. These findings suggest that SWA is a good marker not only for sleep homeostasis but also for cortical plasticity and maturation during brain development. During infancy, memory retention after the exposure to an artificial language was found to be associated with greater fronto-central SWA. On the contrary, another study showed that SWA during nap did not correlate with event-related potentials during the memory test that involved the encoding of novel word meanings. Therefore, the role of SWA in declarative memory during infancy needs to be clarified. Besides SWA, sleep spindle is another NREM feature that is known to reflect neural plasticity, myelination, and brain maturation. More specifically, sleep spindle activity during infancy correlated with the offline generalization of new word meanings, supporting the role of sleep spindles for aggregating recent memories and for anchoring new knowledge into semantic long-term memory. , A recent study assessing the associations between the SWA and sigma powers and psychomotor development measured by Bayley-III in 36 infants at the age of 8 months showed that the occipital SWA and centro-occipital sigma power correlated with cognitive scales, and the frontal and occipital SWA and centro-occipital sigma power correlated with language and fine motor scales. In fact, SWA and sleep spindles are implicated in various neurodevelopmental disorders, including autism spectrum disorder (ASD) and attention-deficit hyperactivity disorder (ADHD).
Circadian disruptions may promote vulnerability to or the progression of certain disorders, particularly in a developmental context. Dysfunction in circadian rhythms and sleep disturbance are often associated with childhood neurodevelopmental disorders. Unaffected parents of children with ASD have lower evening melatonin levels and lower activity of the acetylserotonin methyltransferase (ASMT) enzyme, which converts N-acetylserotonin to melatonin. These findings suggest a genetic basis for impaired melatonin synthesis in ASD. Moreover, melatonin is a potent antioxidant. Therefore, reductions in melatonin during early development may lead to an accumulation of oxidative stress, causing harm to the developing brain and increase the risk of neurodevelopmental disorders such as ASD. Treatments and interventions that target the circadian rhythms may improve clinical symptoms and daily functioning for the individual, for example, regular sleep and activity schedules seem to ameliorate daytime sleepiness and daytime behavioral problems in children and adolescents with ASD or ADHD.
Summary
Infant sleep development is a highly complex and dynamic process in parallel to rapid neurocognitive and physical growth. It is regulated by circadian and homeostatic processes, which change across the lifespan with the most prominent change observed during childhood. Little is known about the interaction between the two processes during infancy. While there is a high interindividual and intraindividual variability in sleep development, how variations in sleep relate to developmental outcomes and the mediation by environmental and social factors in healthy infants remain to be elucidated. Further research is needed to enhance our understanding of the maturational change and the functional role of sleep during early development.
References
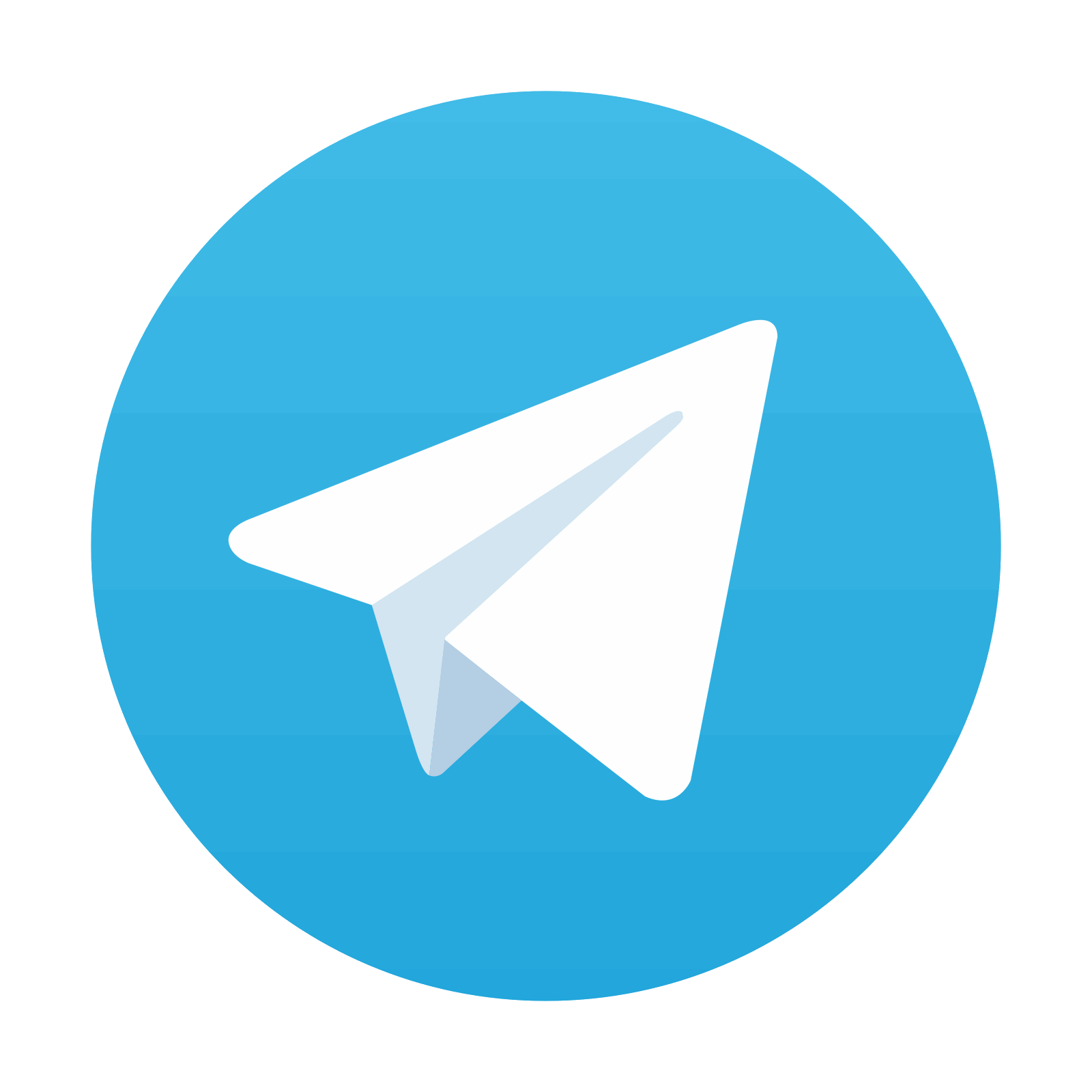
Stay updated, free articles. Join our Telegram channel

Full access? Get Clinical Tree
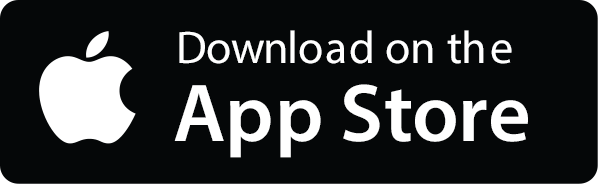
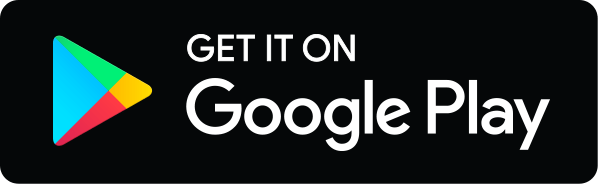
