Abstract
Oxidative stress (OS) is the consequence of an imbalance between reactive oxygen species (ROS) and the failure of antioxidants to neutralize excessive ROS production. Although many sperm functions require physiological levels of ROS, excessive levels of ROS are detrimental to the sperm [1]. OS is one of the most common etiologies of male infertility affecting 30–80 percent of infertile men [2, 3]. The role of OS in men with unexplained infertility has been clearly established [4]. OS affects sperm quality as a result of alterations in proteins, lipid peroxidation, DNA damage and apoptosis [1]. Damage to sperm DNA can compromise the contribution of paternal genome to the embryo [4]. Hence the advent of numerous tests to diagnose OS in the semen. There are several laboratory tests available to measure OS – both direct and indirect. Direct tests measure OS or free radicals such as ROS and reactive nitrogen species. These include chemiluminescence, nitroblue tetrazolium, cytochrome C reduction test, electron spin resonance, fluorescein isothiocynate (DFITC)-labeled lectins, and measurement of oxidation reduction potential. Indirect tests measure oxidized products resulting from ROS sources such as the oxidized form of nicotinamide adenine dinucleotide (NADPH)-oxidase in the sperm, the reduced form of NAD (NADH)-dependent oxidoreductase in mitochondria, or leukocytospermia. These include myeloperoxidase or Endtz test, antioxidants (both enzymatic and non-enzymatic), lipid peroxidation, and DNA damage. In this chapter we will discuss the indirect tests that are available to assess OS and also elaborate on the interpretation and their clinical significance [4, 5].
15.1 Introduction
Oxidative stress (OS) is the consequence of an imbalance between reactive oxygen species (ROS) and the failure of antioxidants to neutralize excessive ROS production. Although many sperm functions require physiological levels of ROS, excessive levels of ROS are detrimental to the sperm [1]. OS is one of the most common etiologies of male infertility affecting 30–80 percent of infertile men [2, 3]. The role of OS in men with unexplained infertility has been clearly established [4]. OS affects sperm quality as a result of alterations in proteins, lipid peroxidation, DNA damage and apoptosis [1]. Damage to sperm DNA can compromise the contribution of paternal genome to the embryo [4]. Hence the advent of numerous tests to diagnose OS in the semen. There are several laboratory tests available to measure OS – both direct and indirect. Direct tests measure OS or free radicals such as ROS and reactive nitrogen species. These include chemiluminescence, nitroblue tetrazolium, cytochrome C reduction test, electron spin resonance, fluorescein isothiocynate (DFITC)-labeled lectins, and measurement of oxidation reduction potential. Indirect tests measure oxidized products resulting from ROS sources such as the oxidized form of nicotinamide adenine dinucleotide (NADPH)-oxidase in the sperm, the reduced form of NAD (NADH)-dependent oxidoreductase in mitochondria, or leukocytospermia. These include myeloperoxidase or Endtz test, antioxidants (both enzymatic and non-enzymatic), lipid peroxidation, and DNA damage. In this chapter we will discuss the indirect tests that are available to assess OS and also elaborate on the interpretation and their clinical significance [4, 5].
15.2 Tests to Detect Factors Causing an Oxidation-Reduction Imbalance
15.2.1 Tests to Detect Leukocyte Products
Apart from immature spermatozoa, presence of white blood cells (WBC) or leukocytes is an important source of ROS in semen [5]. Leukocytes, mainly polymorphonuclear neutrophils (PMN) or granulocytes [6], when activated in the presence of a chronic infection or inflammation due to male accessory gland infection due to prostatitis epididymitis or inflammation of the seminal vesicles can release 100-fold higher levels of ROS compared to abnormal spermatozoa [7, 8]. Leukocyte activation results in increased NADPH production via the hexose monophosphate shunt. This increases superoxide anion concentration which results in oxidative stress [9]. Leukocytes when present in concentrations >1 × 106 WBC/mL of semen result in leukocytospermia [10].
15.2.1.1 Granulocyte Elastase Enzyme Immunoassay
Elastase is one of the major proteomic enzymes released by the leukocytes (granulocytes) at the site of inflammation [11]. During inflammation, polymorphonuclear granulocytes (PMN) discharges proteases such as elastase in large quantity. The elastase-α1–protease inhibitor complex (Ela/α1–PI) of the elastase enzyme is a sensitive marker of genital tract inflammation [12]. In addition, elastase itself causes the release of ROS which when in excess triggers cell deterioration. Politch et al. described this method as a gold standard for leukocytospermia using monoclonal antibodies [13].
15.2.1.1.1 Principle
This method is based on the quantitative determination of granulocyte elastase with alpha 1-proteinase inhibitor.
15.2.1.1.2 Methodology
The semen sample is centrifuged at 300×g for 10 minutes and kept at 37°C for 25 minutes. The supernatant is separated and diluted with phosphate buffered saline (PBS) containing 10 g/L bovine serum albumin (BSA) and 20 mM Ethylenediaminetetraacetic acid (EDTA). The solution is incubated with 500 μL of sample diluent for one hour at 37°C and then washed with distilled water containing 0.5 g/L polyoxyethylene sorbitan monolaurate (PESM). The mixture is then incubated with 500 μL reagent with alkaline phosphatase against α-1 proteinase inhibitor complex for one hour at 37°C. This complex is prepared by adding 2 mL of 30 mM tris(hydroxymethyl)aminomethane/HCl buffer containing 85 mM NaCl to a mixture of 0.5 mg elastase and 2.8 mg α-1 proteinase inhibitor incubated for 30 minutes at 37°C. The sample is washed again with distilled water containing 0.5 g/L PESM and incubated with 500 μL of 10 mM of 4-nitrophenyl phosphate in 1molar diethanolamine/HCl-buffer containing 0.5 mM MgCl2. The reaction of the proteinase enzyme reaction is stopped by adding 500 μL of 2 M NaOH and the absorbance is read at 405 nm [14].
15.2.1.1.3 Interpretation
The concentration of elastase in the sample is determined colorimetrically using a standard curve, and expressed in ng/mL. A three-step grading system of PMN-elastase values are described below:
Study shows elastase concentrations to be highly correlated with the number of peroxidase-positive cells (p<0.01) and negatively correlated with sperm vitality (p<0.01). Furthermore, significantly negative correlations were seen with sperm motility (p<0.05), progressive motility (p<0.05) and sperm morphology (p<0.05). In addition, a significant negative correlation was observed between elastase concentrations and percentage of spermatozoa with intact DNA [11].
15.2.1.2 Myeloperoxidase Test or Endtz Test
The test is recommended by the World Health Organization (WHO) to detect leukocytospermia and is performed when the number of round cells is ≥1.0 × 106/mL on routine semen analysis for men presenting with infertility [10].
15.2.1.2.1 Principle
The Myeloperoxidase or Endtz test is based on the principle that peroxidase present in the leukocyte granules or polymorphonuclear granulocytes oxidize the substrate benzidine from a colorless form to an insoluble/brown derivative in presence of hydrogen peroxide (H2O2) [6, 15]. Myeloperoxidase presenting granulocytes such as neutrophils, polymorphonuclear leukocytes, and macrophages can thus be differentiated from germinal cells.
15.2.1.2.2 Methodology
The stock solution is prepared by mixing 50 mL of 96 percent ethanol, 0.125 g benzidine and 50 mL of sterile water. Working Endtz solution is prepared by adding 2 mL of stock solution and 25 µL of 3 percent H2O2. A 20 µL aliquot of the liquefied semen is vortexed and mixed with equal volume of PBS (pH 7.0) in a microfuge tube to which 40 µL of the working Endtz solution is added. The mixture is vortexed and incubated for five minutes at 37°C. The Makler counting chamber is loaded with 5 µL of the above suspension and examined for cells that stain dark brown, indicating that they are peroxidase containing granulocytes (Figure 15.1). These granulocytes are counted in all 100 squares of the grid in a Makler chamber under 10× bright field objective [16].
15.2.2 Pro-Inflammatory Cytokines and Other Immune Factors
Seminal plasma contains many cytokines and immunological factors such as: interleukins (IL) (IL1α, IL 1β, IL 2, IL6, IL 8, IL10 and IL 12); interferons (IFN-α and γ); macrophage inflammatory protein (MIP-1α and 1β); Regulated on Activation Normal T cell Expressed and Secreted (RANTES) protein also known as chemokine ligand 5; stromal cell-derived factor (SDF-1α); transforming growth factor (TGF-β1) and immunoglobulins (IgA and IgG). These can be measured using Bio-Plex or enzyme-linked immunosorbent assay to determine quantities of immunoglobulin (Ig) isotypes, chemokines, cytokines and growth present in the semen [21]. The concentrations are presented in pg/mL or ng/mL of immunoglobulins.
15.2.2.1 Clinical Significance
Semen of healthy, fertile men contains a broad array of immunologic factors. Many studies demonstrate the significance of elevated pro-inflammatory cytokines in altered sperm function [22]. Pro-inflammatory cytokines increase lipid peroxidation of the sperm membranes [23, 24]. Although they contribute to OS, they are intermediate rather than causative factors of OS [25].
15.2.3 Measurement of Antioxidants
The antioxidant capacity of the seminal fluid can be assessed by measuring individual antioxidants or total antioxidant capacity (TAC) wherein the reducing capacity of various antioxidants against an oxidative reagent is evaluated. The antioxidant capacity of seminal plasma is the sum of enzymatic (superoxide dismutase, catalase, glutathione peroxidase) and non-enzymatic antioxidants (α-tocopherol (Vitamin E), ascorbate (Vitamin C), β-carotene (Vitamin A), folic acid (Vitamin B9), ferritin and carnitines, N-acetyl L-cysteine, coenzyme Q10, ceruloplasmin, selenium, L-arginine, urate and zinc) [26, 27]. A number of assays are available to measure antioxidants. These are described below:
15.2.3.1 Total Antioxidant Capacity Measurement
Total antioxidant capacity (TAC) assay measures the ability of antioxidants in the seminal plasma to scavenge the stable blue-green radical cation ABTS+ (2,2′-azinobis(3-ethylbenzothiazoline-6-sulfonic acid). The ability of the antioxidants to reduce the absorbance at 750 nm by inhibiting the oxidation of ABTS to generate ABTS+ is compared to a water-soluble analogue, Trolox (6-hydroxy-2, 5, 7, 8-tetramethylchroman-2-carboxylic acid) used as a standard [28].
The antioxidant assay buffer concentrate is diluted 10 times in a conical tube. The lyophilized metmyoglobin powder is added to 600 μL of the prepared assay buffer. The working solution is prepared by two serial dilutions, first by adding 10 μL of 8.82 M hydrogen peroxide to 990 μL of ultrapure water and then further diluting 20 μL of this with 3.98 mL of ultrapure water again. Chromogen (containing ABTS) is added to 6 mL of ultrapure water under indirect light as it is light sensitive.
The seminal plasma samples are centrifuged at 300×g for seven minutes and then diluted with the assay buffer at a ratio of 1:9. Trolox standards and reagents are prepared as per the manufacturer’s instructions at the time of the assay. One milliliter of reconstituted lyophilized Trolox is used to prepare the standard curve. Ten microliters of Trolox standard and test samples are loaded into the corresponding wells of a 96-well plate (Cayman Chemical, Ann Arbor, MI). Ten microliters of metmyoglobin along with 150 μL of chromogen are added to all standard and sample wells. The addition of 40 μL of hydrogen peroxide as quickly as possible initiates the reaction. The plate is then covered and incubated for five minutes on a horizontal plate shaker at room temperature (Eppendorf MixMate, Hauppauge, NY). Absorbance is monitored at 750 nm using a microplate reader (BioTek Instruments, Inc., Winooski, VT) [28].
Calculation of assay results: Determination of the reaction rate was done by calculating the average absorbance of each standard and sample. The average absorbance of the standards as a function of the final Trolox concentration (μM of Trolox equivalent) is plotted for the standard curve in each run, from which the unknown samples are determined.

The total antioxidant concentration of each sample is calculated using the equation obtained from the linear regression of the standard curve by substituting the average absorbance values for each sample into the equation [28] (Figure 15.2). In healthy men, the reference value for TAC levels in seminal plasma is >1900 µM Trolox equivalent. The sensitivity, specificity, positive and negative predictive value and accuracy of the TAC assay is shown in Figure 15.3. Infertile men have lower TAC values compared to healthy men (Figure 15.4).
Figure 15.3 Receiver Operating Characteristics (ROC) curve showing the Area under Curve (AUC), cut-off, sensitivity, specificity, positive predictive value, negative predictive value and accuracy of the assay.
Figure 15.4 Distribution of TAC levels between healthy donors (n = 46) and infertile patients (n = 279).
15.2.3.2 Ferric Reducing Antioxidant Power Assay
Ferric Reducing Antioxidant Power (FRAP) Assay is a spectrophotometric method, that works on the principle of the reducing ability of phenols to convert yellow ferric 2,4,6-tripyridyl-s-triazine (Fe3+-TPTZ) to blue ferrous complex (Fe2+-TPTZ). The change in absorbance is monitored at 593 nm to calculate the antioxidant capacity [29].
The difference in absorbance is translated into a FRAP value expressed in micromoles (µM) by comparing the absorbance at 593 nm of test sample to that of a standard solution of known FRAP value [29].

The FRAP assay offers a putative index of the antioxidants or reducing agents potential of biological fluids.
15.2.3.3 Oxygen Radical Absorbance Capacity
Oxygen Radical Absorbance capacity (ORAC) is the direct capacity of peroxyl radical-induced oxidation by chain-breaking antioxidants such as α-tocopherol. It measures the hydrogen atom donating ability of antioxidants. In this assay, β-phycoerythrin (β-PE) is used as the fluorescent probe, 2,2′-azobis(2-amidinopropane) dihydrochloride (AAPH) as a peroxyl radical generator and Trolox as a control or standard.
When AAPH is added to the sample, it slows the decrease in the fluorescence intensity. The loss of fluorescence indicates the extent of decomposition, when it reacts with the peroxyl radical. In the presence of antioxidants, this decrease in the intensity is delayed and is proportional to the amount of antioxidants present in the system [30].
The antioxidant capacity is obtained by calculating the difference in the decrease of the fluorescence intensity between sample and Trolox. Results are expressed as ORAC units, where 1 ORAC unit equals the net protection conferred by 1 μM Trolox.
15.2.4 Measurement of Individual Antioxidants, Micronutrients and Vitamins
Individual antioxidants can be identified by assessing the activity of enzymes highly specific to a particular antioxidant, particularly for superoxide dismutase (SOD), catalase, and glutathione peroxidase (GPx) – the three vital ROS scavengers in the semen.
15.2.4.1 Superoxide Dismutase
Superoxide dismutase (SOD) catalyzes dismutation of superoxide and prevents lipid peroxidation. It scavenges both extracellular and intracellular superoxide anion, a major source of ROS, into oxygen and hydrogen peroxide. SOD activity may be quantified according to the method described by Fridovich et al. [32]. In this method, xanthine and xanthine oxidase generates superoxide radicals that react with 2-(4-iodophenyl)-3-(4-nitrophenol)-5- phenyltetrazolium chloride (INT) to produce a red formazan dye. SOD is measured by the inhibition of the reaction indicated by the decrease in red color measured at 505 nm. The assay medium consists of phosphate buffer, a 3-cyclohexilamino-1-propanesulfonic acid (CAPS) buffer solution and substrate xanthine, INT and xanthine oxidase. One unit of enzyme activity is defined as the amount of SOD capable of inhibiting 50 percent of nitrite formation at the particular absorbance. SOD activity is expressed as U/mg of protein [32].
15.2.4.2 Catalase
Catalase is the second most abundant enzymatic antioxidant (after superoxide dismutase) responsible to balance the levels of reactive oxygen species. Catalase acts to dissociate hydrogen peroxide (H2O2) into oxygen and water. The assay medium consists of EDTA buffer solution, phosphate buffer solution, and H2O2. Catalase activity is measured by the spectrophotometric method in which the change of absorbance at 240 nm occurs at high H2O2 levels. Hydrogen peroxide inhibits the catalase enzyme and the decrease in concentration of catalase is measured at 240 nm. The calculation is based on the rate of reduction of the absorbance for one minute. The catalase activity is expressed as µmole of H2O2 decomposed/min/mg protein [33].
15.2.4.3 Glutathione Peroxidase
Glutathione peroxidase (GPx) is a major enzyme that removes H2O2 generated by SOD in cytosol and mitochondria [34]. GPx catalyzes both H2O2 and organic peroxide (ROO) decomposition generating glutathione disulphide (GSSG), water and organic alcohol (ROH) with the release of reduced glutathione (GSH). The reaction is initiated by the addition of 25 μL of 0.72 mM cumene hydroperoxide and the change in absorbance is monitored at 340 nm at 30°C for 15 minutes. GPx catalyzes the oxidation of glutathione by cumene hydroperoxide. In the presence of glutathione reductase and NADPH, oxidized glutathione is immediately converted into the reduced form with concomitant oxidation of NADPH to NADP+. Reduced glutathione is used as the control or standard. This oxidation process is associated with a decrease in absorbance at 340 nm, allowing GPx activity to be monitored colorimetrically. GPx activity is expressed as mU/mL seminal plasma. One enzymatic unit is defined as 1 μmole of oxidized NADPH per minute at 30°C by the glutathione reductase-linked kinetic reaction [35].
15.2.4.3.1 Clinical Significance
Although SOD, catalase and GPx levels correlate with routine semen parameters [35], the individual levels required to preserve fertility in men have not yet been established. This is particularly difficult as all the antioxidants combined play a role in scavenging ROS.
15.2.4.4 Ascorbic Acid (Vitamin C)
Ascorbic acid concentration in the seminal fluid (400 µM) is much higher than its concentration in the blood plasma (60 µM), reflecting an important physiological role [36]. The seminal fluid is incubated with 9 vol. of 5 percent metaphosphoric acid (1 mM EDTA and 2 mM cysteine) which reduces ascorbic acid to dehydroascorbate, and is then treated with 2,4‐dinitrophenylhydrazine (pH of <2) to form bis-2,4‐dinitrophenylhydrazine. Sulfuric acid is mixed with the bis derivative and the red color produced is measured at 520 nm [37].
Fraga et al. demonstrated that a reduction of dietary intake of ascorbic acid from 250 mg/dL to 5 mg/dL reduced the level of ascorbic acid concentration in the seminal plasma [37]. Furthermore, seminal ascorbic acid level was significantly lower in the patients with leukocytospermia compared to patients with normal semen parameters. A significantly greater percentage of men with abnormal DFI were observed in the patients with low levels of seminal ascorbic acid compared with those with normal or high levels of ascorbic acid (59% versus 33%, p<0.05). Men with lower ascorbic acid levels were reported to have sperm DNA damage [37]. However, wide discrepancies in the results have been reported [37, 38]. Assessment of individual antioxidant, micronutrient or vitamin does not provide an overall picture of the seminal antioxidant status.
15.3 Tests to Detect Effect of Oxidative Stress on Sperm
Unlike the somatic cells, the lipid bilayer in the plasma membrane of the human spermatozoa is rich in polyunsaturated fatty acids making them highly susceptible to damage caused by excess ROS [39–41].
The breakdown of polyunsaturated fatty acids to form lipid peroxides is known as lipid peroxidation [42]. Lipid peroxides are unstable and decompose to form complex compounds such as 4-hydroxynonenal (4-HNE), acrolein (ACR) and malondialdehyde (MDA) which freely react and modify lipids, proteins and DNA as they are relatively more stable than free radicals. They act as cytotoxic second messengers of oxidative stress causing alterations in the sperm functions [43, 44]. Measurement of the end products of lipid peroxidation is a widely accepted marker of oxidative stress. Some of the common methods to measure lipid peroxidation and oxidative stress are described below.
15.3.1 Measurement of 4-Hydroxynonenal Histidine (HNE-His) Adduct
15.3.1.1 Principle
Highly reactive aldehyde 4-hydroxynonenal (HNE)-protein adducts can be quantified using ELISA with a monoclonal antibody that rapidly detects the HNE-histidine adducts [45].
15.3.1.2 Methodology
Hydroxynonenal modified BSA (HNE-BSA) standards are prepared. Fatty acid free BSA (without HNE) is diluted in PBS (10 mg/mL). One mM of HNE is added to fatty acid free BSA to give 250 pmol/mg of ELISA with non-commercial antibody or 5000 pmol/mg of ELISA with commercial antibody. These stock solutions are incubated overnight at 4oC to allow binding of HNE to BSA and stored at -20oC.
The samples and standards are diluted in 0.05 M carbonate binding buffer (pH 9.6; 0.015 M sodium carbonate, 0.035 M sodium bicarbonate) and 100 mL of this mixture is placed in ELISA plate wells, kept overnight at 4oC for protein absorption and washed with 300 mL of PBS the following day. Freshly prepared blocking solution consisting of 5 percent fat free dry milk in carbonate is added to these wells and incubated for 2.5 hours at 37oC and washed with 0.1 percent Tween 20 in PBS.
Primary antibody solution (1 percent BSA in PBS; 1:100 for non-commercial; 1:500 for commercial antibody) is incubated for two hours at 37oC and then washed. The plate is then incubated with peroxidase blocking solution containing 3 percent H2O2 in PBS for 30 minutes at room temperature and washed again. One hundred mL of the secondary antibody solution (1 percent BSA in PBS) is added to the wells and incubated for one hour at 37°C and washed. The wells are then treated with 0.05 mg/mL of freshly prepared substrate solution for 30 minutes at 37oC followed by 50 mL of stopping solution (2 M sulfuric acid) [45].
15.3.1.3 Clinical Interpretation
Predetermined HNE-BSA standard curves are used to compare the results. The absorbance of each well is read on a microplate reader against a primary wavelength of 450 nm. Results are expressed as pmol/mg.
15.3.1.4 Clinical Significance
4-HNE may impair sperm capacitation by targeting the protein kinase A affecting the tyrosine phosphorylation pathway and thus reducing sperm motility [46].
15.3.2 Measurement of Isoprostanes
F2-isoprostanes (F2-IsoP) is a reliable marker of oxidative stress. Isoprostanes are a series of prostaglandin F2-like compounds formed by peroxidation of arachidonic acid that are bound with phospholipids and are independent of the cyclooxygenase pathway. 8-iso-PGF2α is the most represented isomer for F2-IsoP measurement [47].
15.3.2.1 Methodology
Isoprostanes can be determined by quantification of the amount of F2-IsoP present in the semen which is the sum of free and esterified isoprostanes or 8-iso-PGF2α localization in sperm.
For F2-IsoP determination, samples are incubated in 500 μL/mL of aqueous 1M KOH for 45 minutes at 45°C and 500 μL/mL of 1 M HCl is added to adjust the pH to 3. For an internal standard, 500 pg of derivative of PGF2α (PGF2α-d4) is added to the sample. The sample is then applied to an octadecylsilane (C18) and an aminopropyl (NH2) cartridge to extract the isoprostanes. The carboxylic and hydroxyl groups of F2-IsoP (sum of free and esterified isoprostanes) is derived as pentafluorobenzyl and trimethylsilyl ethers respectively. The F2-IsoP is determined using gas chromatography or negative ion chemical ionization tandem mass spectrometry analysis. The measured ions are derived from precursor ions produced from the 8-iso-PGF2α and PGF2α-d4 [48, 49].
For immunocytochemical staining with polyclonal 8-iso-PGF2α antibody, samples are washed in PBS and air dried on a glass slide and rinsed in PBS again. The sample is treated with methanol for 15 minutes at −20°C to fix the cells. It is further treated with blocking solution containing 1 percent BSA and 5 percent normal goat serum in PBS for 20 minutes at 37°C. The mixture is incubated with monoclonal anti-β-tubulin antibody overnight at 4°C. The following day, the sample is washed thrice and then treated with goat anti mouse IgG antibody. Finally, the samples are mounted on a slide and a cover slip is placed and observed under fluorescence microscope [50].
15.3.2.2 Interpretation
For F2-IsoP determination, 8-isoprostane (IsoP) levels are expressed as ng/mL. For immunocytochemical analysis, a total of 500 sperm are counted in each sample. The signal is absent in normal sperm but shows green fluorescence in sperm with oxidative damage of the lipid membrane.
15.3.2.3 Clinical Significance
Seminal plasma levels free 8-iso-PGF2α are shown to be significantly higher in infertile compared with normozoospermic men [51]. In addition, it has a significant negative correlation with MDA and seminal SOD activity in normozoospermic men [52]. Immunofluorescence analysis shows labeling of mid-piece and tail in as high as 65 percent of sperm in infertile men compared to almost none in fertile men [53].
15.3.3 Thiobarbituric Acid-Reactive Substances Assay
This assay is an indicator of lipid peroxide breakdown products wherein thiobarbituric acid (TBA) reacts with aldehydes mainly derived from peroxides and unsaturated fatty acids. Malondialdehyde (MDA), a reactive and mutagenic end product of lipid peroxidation in semen, is measured using this method [42].
15.3.3.1 Principle
In this assay, MDA (an aldehyde) binds with two molecules of TBA to form the MDA-TBA adduct which is measured colorimetrically or fluorometrically.
15.3.3.2 Methodology
Semen sample is centrifuged and then 100 µL of supernatant containing seminal plasma is diluted with distilled water (1:9) to which 500 µL of TBA reagent containing 0.67 g of TBA in 100 mL of distilled water with 0.5 g NaOH for alkaline denaturation and 100 mL glacial acetic acid is added. This mixture is boiled for one hour at 90–100oC. The samples are quickly cooled in ice and centrifuged for 10 minutes at 4000 × g. The supernatant is left for 20 minutes at 37°C [54]. This is loaded onto a microtiter plate and read at wavelength between 530–550 nm [55].
15.3.3.3 Interpretation
The adduct formation is measured either calorimetrically at 530–540 nm or fluorometrically at an excitation and emission wavelength of 530 nm and 550 nm respectively. Lipid peroxidation in sperm is expressed as nmol MDA/107 sperm.
15.3.4 Tests to Detect Abnormal Sperm DNA Integrity
Oxidative stress is one of the major factors responsible for DNA fragmentation [58]. Furthermore, apoptosis as a result of oxidative stress can also explain the generation of DNA fragmentation [59]. Sperm DNA integrity may be compromised due to advanced age, cigarette smoking, chemotherapy, radiation, cancer, varicocele, leukocytospermia and elevated levels of ROS [60, 61]. Studies show that infertile men have high amounts of impaired DNA integrity [62, 63]. Reduced fertility, embryo development, and increased rates of miscarriages have been reported in cases of higher sperm DNA damage [64].
Several tests have been introduced to measure the sperm DNA damage involving different methodological approaches and principles. Currently, several assays are available to assess sperm DNA damage. Some tests measure the maturity and integrity of sperm chromatin (acridine orange, toluidine blue and chromamycin A3 staining), while others measure DNA fragmentation (sperm chromatin structure assay (SCSA); terminal deoxynucleotidyl transferase dUTP nick-end labeling (TUNEL); sperm chromatin dispersion (SCD) or halo sperm test and single-cell gel electrophoresis (Comet) and 8-hydroxy-2-deoxyguanosine (8-OHdG). Of these, SCA, TUNEL, SCD or halo sperm test and Comet are the commonly used tests of sperm DNA fragmentation with well-established and standardized protocols described in the literature. A cross-sectional survey across 19 countries by Majzoub et al. showed that 30.6 percent of SDF measurements are done using TUNEL and SCSA, while 20.4 percent and 6.1percent are done using sperm chromatin dispersion (SCD) and single-cell gel electrophoresis (Comet), respectively [65]. The details of each of the DNA tests are described in other chapters. A brief description of each test is illustrated in Table 15.1.
Table 15.1 Common Tests of Sperm DNA Fragmentation
Test | Principle | Method | Result | Cut-off values | Advantage | Disadvantage | Reference |
---|---|---|---|---|---|---|---|
SCSA | Measures the susceptibility of sperm DNA to denaturation. | Acid denaturation, followed by staining by AO. Measurement by flow cytometry. | Normal DNA fluoresces green. Denatured DNA fluoresces orange-red. Result presented as DNA fragmentation index (% DFI) and high DNA stain ability (% HDS). | 30% 27% | Reliable estimate of the percentage of DNA damaged sperm. Standardized protocol available. Rapid evaluation of large number of spermatozoa. Correlations with results of other SDF assays. Established clinical thresholds Can be performed on fresh or frozen samples. | Indirect assay involving acid denaturation. Proprietary protocol with no commercial assay. Requires expensive instrument and highly skilled technicians. | [66–68] |
TUNEL | Quantifies the enzymatic incorporation of dUTP into DNA breaks as percentage of fluorescent sperm. | Labeled nucleotides are added to the site of DNA fragmentation. Fluorescence is measured by flow cytometry or fluorescence microscopy. | Sperm with fragmented DNA show fluorescence. Result presented as percentage of fluorescent sperm. | 36.5% 36% 35% 15% | Direct assay can be performed in fresh or frozen samples. Can be performed on few sperm. Detects both single- and double-strand DNA breaks. Commercial assay available. Reliable estimate of the DNA damaged sperm with minimal interobserver variability | Requires standardization between laboratories. Time-consuming Immature spermatozoa are not evaluated. Variable clinical thresholds reported in the literature. | [69–72] |
SCD or Halo test | Assess dispersion of DNA fragments after denaturation. | Agarose-embedded sperm are subjected to a denaturing solution to remove nuclear proteins. Uses bright field or fluorescent microscopy to observe chromatin dispersion after staining. | Sperm with fragmented DNA do not produce halo. Characteristic halo of dispersed DNA loops are observed in sperm with non-fragmented DNA. Result presented as percentage of sperm with non-dispersed chromatin. | 35% 30% | Relatively simple test. | Indirect assay involving acid denaturation. Inter-observer variability. Time-consuming and labor intensive. Inter-observer variability. | [73–75] |
Comet | Electrophoretic assessment of DNA fragments of lysed DNA. | Gel electrophoresis performed in alkaline or neutral conditions. | Size of comet tail represents the amount of DNA fragments that stream out of the sperm head. Result presented as mean amount of DNA damage per spermatozoon. | 56% 45.6% 44% | Direct assay can be performed on few sperm. Detect multiple types of DNA damage of individual spermatozoon Result correlates well with other SDF assays. | Requires fresh sample. Inter-observer variability. Time consuming. Requires experienced observer. | [76–77] |
SCSA: sperm chromatin structure assay; AO: acridine orange; DFI: DNA fragmentation index; HDS: high DNA stainability; SDF: sperm DNA fragmentation; TUNEL: terminal deoxynucleotidyl transferase-mediated deoxyuridine triphosphate-nick end labeling; dUTP: deoxyuridine triphosphate.
15.3.4.1 Clinical Significance of Sperm DNA Fragmentation Tests
The oocyte has the ability to repair sperm DNA damage. Although a sperm with damaged DNA can fertilize an egg, it can result in compromised embryonic growth, miscarriage, or childhood deformities [78–82]. Higher SDF has been shown in men with varicocele compared to fertile men [83]. In a study by Esteves et al. using SCD test, varicocele was identified with 94 percent accuracy based on the rates of degraded sperm determined by the proportion of degraded sperm in the population of spermatozoa with fragmented DNA which was 8-fold higher in men with varicocele than in donors [84]. Following varicocele repair SDF was significantly reduced post-operatively and higher pregnancy rates through natural conception with ART were reported [85]. SDF is also increased in the presence of oxidative stress in these men [86–88]. Other reasons for testing DNA fragmentation are unexplained infertility, failed intrauterine inseminations, recurrent pregnancy loss, in vitro fertilization and intracytoplasmic sperm injection failure, and cancer patients undergoing chemotherapy or radiotherapy [89]. SDF was higher in couples experiencing recurrent pregnancy loss compared to fertile controls (18.8 percent ± 7.0 percent versus 12.8 percent ± 5.3 percent; p<0.9001) [90].
15.3.5 Measurement of Reactive Oxygen Species-Induced Post-Translational Modifications
Post-translational modifications (PTMs) not only confer structural changes in the proteome of the spermatozoa cells, but they also increase the diversity of the proteome and introduce specific modifications that could be translated into functional changes in the affected spermatozoa [91].
A diverse range of PTMs takes place in the developing spermatozoa, of these ROS result in the most common types of PTMs namely, S-glutathionylation (GSS-R), nitrotyrosine modifications (Nitro-Y) and carbonylation [92–94]. Protein modifications by ROS result in altered functions such as activation or inhibition of transcription factors, signal transducers and enzymes [84, 92], thus altering the protein structural and functional integrity.
S-glutathionylation of proteins occurs by the addition of glutathione (GSH) to cysteine residues of certain target proteins under normal conditions as well as under conditions of oxidative stress. The disulfide linkage between the glutathione and the protein is reversible. This modification affects the functions of proteins, enzymes and receptors, thus altering normal cell biology [95].
Nitrotyrosine is formed by the reaction of peroxynitrite or donors of NO• with tyrosine residues [95]. In the sperm cell, the reaction of superoxide and NO• produce nitrotyrosine [96]. The nitrotyrosine protein modification can result in alteration of protein function or structure [97], however low amounts in the spermatozoon are essential for sperm to undergo capacitation [96].
Reactive carbonyls are produced by direct oxidative reaction of specific amino acids namely proline, arginine, lysine and threonine with low- or high-molecular weight dicarbonyls generated during lipid peroxidation and glycoxidation, causing modification of these amino acids to aldehyde or ketone and their eventual cleavage [98, 99].
ELISA is a widely used assay to detect and measure a particular protein using specific antibodies to immobilize proteins in microplate wells. Enzymes are chemically linked with the common antibodies. Such enzyme activities produce a measurable signal when combined with solutions containing appropriate substances [100, 101]. The end-product is a stable color that can be measured colorimetrically or fluorometrically using fluorophore-labeled antibodies particularly in multiplex arrays.
15.3.5.1 Clinical Significance
High amounts of nitrotyrosine were found in patients with impaired motility (asthenozoospermia) or spermatic duct cord blood in varicocele patients [96, 102]. Oxidation products such as protein carbonyls are useful for detection and in estimation of ROS levels in a semen sample [99]. Protein carbonyls are chemically stable, more reliable and frequently used as a marker for protein oxidation [103].
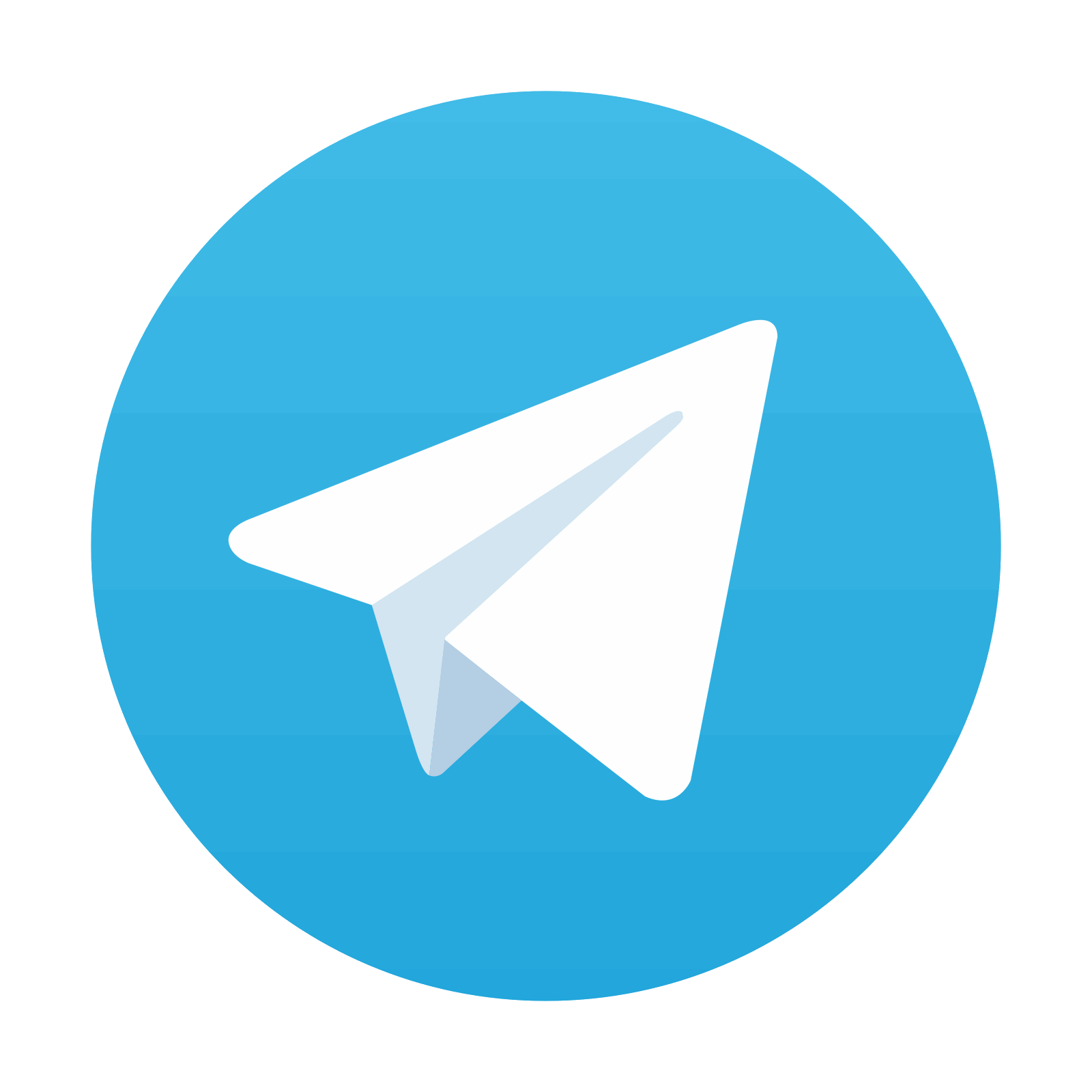
Stay updated, free articles. Join our Telegram channel

Full access? Get Clinical Tree
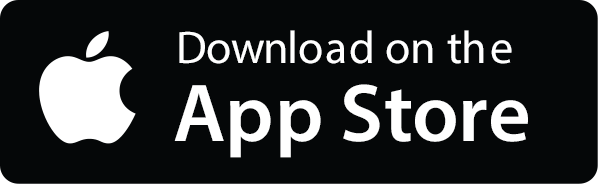
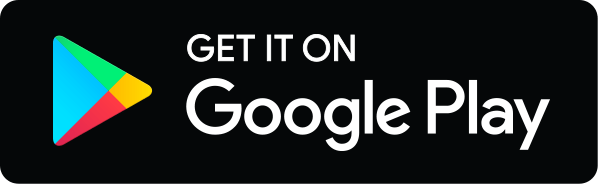
