Key Points
- •
This chapter describes the tissue types present in early embryos and the interactions between these tissues.
- •
The membrane systems and cytoskeletal elements within a typical cell are reviewed.
- •
Early embryos contain only epithelial and mesenchymal populations.
- •
Each tissue type produces specialised extracellular matrix molecules and proteins which permit and encourage tissue interactions.
- •
Specific interactions between developing epithelia and mesenchyme are presented, and the common cytokines and growth factors are discussed.
This chapter provides a brief overview of concepts of cells and tissues and the terminology by which developmental processes are described. It should be noted that the genetic programme of the early conceptus is now being studied by analysis of substances secreted and metabolites produced, some of the very early cell interactions between the fertilised oocyte and the maternal endothelium have been revealed, and the ways in which cell types interact before and during organogenesis is now being explored in human embryonic stem cell and human-induced pluripotent stem cell culture.
General Characteristics of all Cells
All cell types have a plasma membrane and internal organelles, and all are supported by a range of cytoskeletal structures. The details and arrangement of proteins within cells and within the matrices they synthesise encompass a vast region of research beyond the scope of this text. Only the main proteins and structures necessary for the appreciation of developmental processes are presented. Readers are recommended to consult other texts for further details.
Plasma Membrane
The plasma membrane of cells is composed of phospholipids molecules arranged in a bilayer. Within this layer are vast numbers of proteins which may reside entirely within the bilipid layer or protrude intracellularly, extracellularly, or both. Proteins which project exteriorly are covered with carbohydrates as glycoproteins or proteoglycans and contribute to the glycocalyx of the cell ( Fig. 2.1 ). The glycocalyx also contains glycoproteins and proteoglycans which have been secreted into the extracellular space around the cells and then absorbed onto the cell surface. Thus it is difficult to specify exactly where a cell plasma membrane ends and the surrounding extracellular matrix (ECM) begins. The glycocalyx is fundamental in cell–cell and cell–matrix communication. Cells place specific protein and carbohydrate groups into the glycocalyx when touching and adhering to other cells, when displaying cell markers to other cells, in blood clotting cascades and in inflammatory responses.

The membrane systems (organelles) within the embryonic cells (i.e., nucleus, mitochondria, endoplasmic reticulum, Golgi apparatus, lysosomes and secretory vesicles) are also made of the plasma membrane; secretory vesicles can become incorporated into the external plasma membrane to release contained contents. Intracellular membrane systems are supported within the cytoplasm by a range of cytoskeletal elements which also allow cells to maintain surface specialisations (microvilli), to change shape (as in the movements of endocytosis and exocytosis), to move in specific directions (with the glycocalyx molecules) and to adhere strongly to a substrate when movement ceases.
The Cytoskeleton
The cytoskeleton is a highly dynamic network of protein filaments that extends throughout the cell. As it also allows the cell to move or move portions of its plasma membrane, the cytoskeleton is less like a bony framework and more like a moveable muscular system. Three types of protein filaments produce a diverse range of cytoskeletal elements, including actin filaments , microtubules and intermediate filaments ( Fig. 2.2 ); these are synthesised from actin, tubulin and a range of fibrous proteins (e.g., vimentin, laminin, respectively).

Actin filaments
Actin filaments are polar structures composed of globular molecules of actin arranged as a helix. They work in networks and bundles, often found just beneath the plasma membrane, where they crosslink to form the cell cortex. Actin filaments are used to change the shape of the plasma membrane, moving it outwards in projections or inwards in invaginations. Whereas discrete bundles of actin, anchored into the cortex, can produce thin spiky protrusions of the plasma membrane, microvilli, sheet-like extensions of the membrane (lamellipodia) are supported by continuous flattened bundles of actin similarly anchored. Conversely, actin filaments can pull portions of the membrane inwards in the formation of endocytotic vesicles or in cell division. Here, contractile bundles of actin associated with the motor protein myosin form. Although myosin is most familiar in muscle fibres, nonmuscle cells contain various myosin proteins. Contractile bundles of actin filaments and myosin filaments are synthesised for specific functions and then disassembled (e.g., during cell division after chromosomal separation, the plasma membrane constricts to the middle of the cell, allowing two daughter cells to separate). Such assemblies of actin and myosin are also found in development near the apical surface of epithelial cells where they play a role in folding of epithelial sheets and in mesenchyme where they can form stress fibres which allow the cells to exert tension on the ECM.
Microtubules
Microtubules are long, hollow cylinders composed of the globular protein tubulin. They are much more rigid than actin filaments. Microtubules emanate from the centre of the cell in a region termed the centrosome . They lengthen by adding tubulin to the proximal end of each microtubule while subunits are lost from the distal end. The centrosome offers a focus and region of stabilisation for the proximal ends of the hundreds of microtubules in a cell; it also contains the two centrioles which are used by the cell when dividing. Vast numbers of microtubules extend in all directions to the plasma membrane and seem to ensure that the centrosome is at the centre of the cell. From this position, the microtubule array sites the other cellular organelles and holds them in place using a range of contact proteins. If a cell touches another cell, there may be internal movements of the organelles driven by the microtubules, resulting in repositioning of the centrosome. Microtubules display a dynamic instability, with new subgroups being added or subtracted very rapidly. The turnover of distal units can be slowed by contact with proteins close to the plasma membrane; this allows cells to maintain a particular shape and polarity. Microtubules are also used in cell-surface specialisations, where they form the basis of cilia in the familiar 9+2 arrangement of nine microtubule doublets around a pair of single microtubules.
Intermediate filaments
Intermediate filaments are made of a variety of proteins all formed from highly elongated fibrous molecules. They are arranged as rope-like fibres which span each cell often from one cell junction to another. They are termed intermediate filaments because their apparent diameter on electron microscopy is between that of actin filaments and thick myosin filaments. Specific varieties of intermediate filaments are present in epithelial and mesenchymal cells. Whereas epithelial cells contain keratin filaments, mesenchymal cells have vimentin and vimentin-related filaments, and in the cells which will develop a myogenic lineage, desmin filaments are seen. Neuroepithelial cells develop neurofilaments and glial fibrillary acidic protein filaments are seen in astrocytes.
Embryonic Tissues
Two early tissue arrangements can be seen in embryos – epithelia and mesenchyme. Individual cells within each arrangement secrete extracellular proteins which form the ECM. This structures the space around and within cell populations and provides the appropriate conditions for development.
Epithelia
Cells composing epithelia are polarised with apical and basal surfaces. Whereas the apical surface commonly displays specialised features such as microvilli, the basal surface is the site of extracellular protein deposition in the form of a basal lamina. Laterally, the cells contact their neighbours via varieties of juxtaluminal junctional complexes which bridge the narrow intercellular clefts. Epithelial cell polarity factors regulate the relative size of the surfaces or domains and the internal organisation of the cytoskeleton. The transmembrane protein Crumbs specifies the apical domain, Baz/PAR-3 controls the position and extent of the junctions, Scribble restricts the size of the junctional domains, an internal contractile actomyosin network which produces the planar polarity of epithelia is contiguous across the lateral borders of the cells through E-cadherin adhesion, and the basal region displays integrins which link to the basal lamina.
Basal lamina
Basal laminae are thin, flexible sheets of ECM which are made by and underlie epithelial cells ( Fig. 2.3 ). Basal laminae are also found surrounding individual skeletal muscle fibres, fat cells and Schwann cells. The presence or absence of a basal lamina beneath an epithelium during development is of consequence. Basal laminae organise the proteins in adjacent cell membranes, induce cell differentiation and cell metabolism, serve as routes for cell migration and can influence cell polarity in those cells that touch them; they can change with time during development and thus can maintain a developmental impetus.

The basal lamina is described in electron microscopic studies as having an electron lucent layer, the lamina lucida or rara , closest to the basal surface of the cell and an electron-dense layer, the lamina densa below. If the epithelial layer rests on underlying mesenchyme, a layer of collagen fibrils connects the basal lamina to the underlying tissue, sometimes with specialised anchoring collagen fibrils. The strength of this connection is important for development and growth. Many textbooks do not distinguish between the basal lamina as described and the basement membrane , a thicker layer which includes the basal lamina and extracellular components of the underlying connective-tissue matrix.
Embryonic basal laminae are composed chiefly of laminin . Later, other extracellular molecules such as type IV collagen , perlecan and entactin (see below) contribute to the feltwork of the layer (see Fig. 2.3 ). In some regions of the body, basal laminae form specialised structures or units which have a specific function during development or in adult life. An example of this arrangement is seen in tooth development, where initially ameloblasts and odontoblasts are separated by a basal lamina. The ameloblasts deposit enamel directly onto one side of this basal lamina, and the odontoblasts deposit dentine onto the other (see Fig. 2.12 ); in this way, the tooth is formed. In both the kidneys and the lungs, the basal lamina from the specialised cells of the organ abuts directly onto the endothelial basal lamina, producing a selectively permeable barrier. In the kidneys, this is the glomerular basement membrane.
In development, the basal lamina acts as a selective barrier to the movement of cells, and migrating cells will move along basal laminae but not through them. Cells beneath an epithelial layer see only the basal lamina which the overlying cells produce. Changes in the local basal laminal composition is one way by which the epithelial cells can communicate with the cells migrating beneath them. In adult tissues, the basal lamina permits the movement of macrophages, lymphocytes and nerve processes and plays an important part in tissue regeneration after injury.
Cell–Cell junctions
Juxtaposed cells usually do not touch. For contact to be established, the cells produce specific molecules which promote the development of a cell–cell junction between them ( Fig. 2.4 ). Junctional complexes allow sheets of epithelial cells to act in concert in maintaining a barrier or in producing alterations in the overall epithelial morphology; they also permit cell–cell communication and are in this respect especially important in development. Cell junctions are classified into three main groups: (i) tight junctions , which prevent leakage of molecules between cells from one side of a sheet of cells to the other ; (ii) anchoring junctions , where the neighbouring cell membranes attach and are supported by cytoskeletal elements within the cells, either actin or intermediate filaments (this type of junction also anchors epithelial cells to the ECM); and (iii) communicating junctions , which mediate the passage of electrical or chemical signals from one cell to another.

The formation of these junctional complexes is dependent on a range of cell adhesion molecules (CAMs) (see Fig. 2.4 ). In cell–cell anchoring junctions (adhesion belts and desmosomes), the CAMs involved are termed cadherins ; they are attached intracellularly to intermediate or actin filaments in the cell cortex. The latter run parallel to the plasma membrane; thus the actin bundles of adjacent cells are linked. Concomitant contraction of the actin bundles results in narrowing of the apices of the epithelial cells and rolling of the epithelial layer into a deep groove or a tube.
Epithelial cells contact the underlying basal lamina they synthesise by different types of anchoring junctions (hemidesmosomes and focal contacts). In these cases, the transmembrane linker proteins belong to the integrin family of ECM receptors. Cytoskeletal filaments support the connection of the integrin within the cell membrane to the ECM.
Communication between adjacent epithelial cells is mediated by gap junctions . In forming a gap junction, each cell contributes six identical protein subunits (called connexins ) which form a structure, similar to an old-fashioned cotton reel, termed a connexon . This is situated across the bilaminar membrane with the thicker rims extending into the extracellular and intracellular spaces. Each connexon is capable of opening and closing, thus controlling the gap. When two connexons from adjacent cells are aligned, a tubular connection is made between the cells. Each gap junction is really a cluster of apposed connexons which each permit molecules smaller than 1000 daltons to pass through them. In early embryos, most cells are electrically coupled to one another by gap junctions. Later in development, epithelial cells synthesise gap junctions at particular stages when it is inferred that information is passing from cell to cell. When gap junctions are removed, there is often a difference in differentiation in the cellular progeny. Gap junctions are seen in adult tissues (e.g., connecting cardiac myocytes to permit transmission of the electrical signals of the cardiac cycle). (For further information on CAMs, see Alberts et al. )
Mesenchymal Cells
Mesenchymal cells, in contrast to epithelial cells, have no polarity and thus no directional surface specialisations. They have junctional complexes which are not juxtaluminal, and they produce extensive ECM molecules and fibres from the whole cell surface ( Fig. 2.5 ). As development proceeds, proliferating mesenchymal populations begin to differentiate. This is often first seen by the upregulation of specific mRNA in the cell or in the production of different ECM molecules by selected progeny.

Extracellular matrix
A substantial part of the developing embryo is made up of ECM. This name is given to the vast array of complex molecules which are secreted locally by mesenchymal cells and assembled into networks which structure the spaces between the embryonic cells. In early development, mesenchymal populations are composed of migrating epithelial cells and mesenchymal cells generated from germinal (proliferative) epithelia. It is the latter group which will give rise to the range of connective tissues seen in the adult. Connective tissues form the architectural framework of the body, and the matrices determine the tissue’s physical properties (i.e., in bone, cartilage or fascia). Variation in the constituents and amount of the matrix molecules gives the diversity of connective tissues ( Fig. 2.6 ).

There are two main classes of ECM molecules, glycosaminoglycans (GAGs) which may be linked covalently to proteins as proteoglycans , and fibrous proteins , such as collagen , elastin , laminin and fibronectin . The GAG and proteoglycan molecules form a highly hydrated gel-like ground substance into which the fibrous proteins are embedded.
Four main groups of GAGs have been described: (i) hyaluronic acid, (ii) chondroitin sulphate and dermatan sulphate, (iii) heparan sulphate and heparin and (iv) keratan sulphate. Hyaluronic acid is the simplest GAG. It is especially abundant in embryos, where it fills the spaces between cells and because of its level of hydration becomes turgid and generally resists compression. By synthesising hyaluronic acid, cells can open up migration pathways or support epithelia which are undergoing morphological change. The other GAGs, which are more complex than hyaluronic acid, have much shorter disaccharide chains, contain sulphated sugars and are usually bound to a protein core forming proteoglycans. Aggregates of hyaluronate and proteoglycans can make huge molecules which occupy a volume equivalent to a bacterium.
Within tissues, GAGs can form gels of varying pore size and thus act as filters regulating the movement of molecules according to their size or charge. The heparan sulphate proteoglycan perlecan is found in the basal lamina of the kidney glomerulus and functions as a filter in the glomerular basement membrane. Proteoglycans which bind various growth factors act as reservoirs for messages which can be positioned in the matrix by cells at one stage to be read by cells developing later. GAGs and proteoglycans associate with the fibrous proteins in the matrix and provide support between the fibres.
Collagens form the major fibrous proteins in the matrix. Several families or groups of collagens are described, each made from a range of basic α-chains and each encoded by a separate gene. Each collagen molecule is made from three α-chains wound around one another like a rope. The main types of fibrillar collagen found in connective tissue are types I, II, III, V and XI. These types aggregate into the huge hawser-like bundles which can be seen on electron microscopy. Collagen types IX and XII are smaller and link the larger fibres to one another and to other matrix molecules. Types IV and VII are found in the basal laminae, where type IV forms the feltwork of the mature basal lamina, and VII forms the anchoring structures which attach the basal lamina to the underlying matrix. Collagen is used to provide the initial matrices for cartilage and bone and is particularly seen in tendons and ligaments. In these cases, the amount of GAGs is reduced, and the collagen fibres are aligned by the fibroblasts in response to the direction of the stresses acting on the collagen. In this way, the connective tissues of the body are responsive to the physical demands placed upon them. Anomalies of collagen synthesis can give rise to diseases in life (e.g., the condition osteogenesis imperfecta is caused by mutations in type I collagen production, leading to bones which are brittle and fracture with little stress; mutations in type II collagen lead to disorders of cartilage).
Elastin is composed of short elastin proteins which are crosslinked so that when relaxed, the fibres are randomly coiled but when stretched each elastin molecule can expand so contributing to the overall effect. Elastic fibres are at least five times more extensible than a rubber band of the same cross-sectional area. Usually collagen fibres are interwoven with elastic fibres to prevent overstretching and damage.
Laminin is one of the first extracellular proteins synthesised in the embryo, forming most of the early basal laminae. Each molecule is shaped like an asymmetric cross. Molecules join together to form a feltwork often supported by smaller entactin molecules.
Fibronectin is a high-molecular-weight glycoprotein found in extracellular matrices and in blood plasma. Within the ECM, fibronectins promote cells adhesion. Generally, contact with fibronectin causes cells to move. It has been shown in culture that neural crest cells preferentially migrate along fibronectin-rich substrates, and within three-dimensional cultures, migrating cells can achieve their greatest speeds migrating along fibronectin pathways. It is interesting to note that bonding with fibronectin does not necessarily fix a cell to one position within the matrix, so contacts with this protein are made and then released with ease.
Biomechanical properties of the ECM
It is now appreciated that mechanical characteristics and movements of the ECM are fundamental to developmental processes and that mesenchymal cells are sensitive to the tension and stiffness of the matrix itself. The application of shear forces to matrix molecules results in fibre alignment along the axis of force and the development of tension: an individual mesenchymal cell is able to sense parts of the a matrix as soft or stiff depending on whether the tension is generated perpendicular (soft) or parallel (stiff) to a fibre. ECM movements occur across tissue-level scales exhibiting both vortical and convergent extension motion patterns. The physical-chemical and biochemical reactions which drive matrix fibre assembly collectively exert compression and stretching forces on embryonic cell arrangements.
Cell–Matrix junctions
Cells interact with the ECM molecules via protein receptors or co-receptors in the plasma membrane. Syndecans are proteoglycan co-receptors which span the plasma membrane. The extracellular part carries chondroitin sulphate and heparan sulphate chains while the intracellular domain interacts with the cell cortex actin filaments. Integrins are receptor proteins which bind to and respond to the ECM information. An extracellular receptor site binds with matrix molecules, especially fibronectin and laminin; intracellularly, the integrin binds via vinculin to the actin cytoskeletal network or intermediate filaments. When the integrins in the cell membrane contact a matrix molecule (e.g., fibronectin), the orientation of the fibronectin will cause alignment of the actin cytoskeleton and a reorientation of the cell itself. Later, when the cells are depositing ECM molecules, the actin cytoskeleton will exert forces to orient the matrix molecules in a similar configuration. Thus the interaction between matrix molecules and cells and then cells and matrix can drive development and propagate order from cell to cell. The cell–matrix junctions permit communications within the embryo just as gap junctions permit communications between cells. However, the cell–matrix mechanisms allow messages to be left in the matrix which may indicate migration routes or halt migrating cells and suggest a differentiation pathway. The matrix information system can thus control the temporal pattern of development.
Transformation from Epithelium to Mesenchyme
The two embryonic states of epithelial tissue and mesenchyme are not necessarily immutable, and transition from epithelia to mesenchyme and vice versa occurs during development. However, such a change requires a temporal or external inductive agent and causes dramatic upregulation and synthesis of a whole variety of special intra- and extracellular molecules as previously described. Generally, in an embryo, epithelial cells seem to derive from existing epithelial populations, but mesenchymal cells are produced initially from proliferative (germinal) epithelia and then later by amplifying mitoses within the mesenchymal population. Changes from one cell state to another are considered important in development.
The early migrating cells derived from the primitive streak have a mesenchymal morphology yet become epithelial when they reach their destinations forming the somites, the somatopleuric and splanchnopleuric epithelia lining the intraembryonic coelom, including the lining of the pericardial cavity. All of these epithelia become germinal centres which produce further mesenchymal populations. However, an additional group of mesenchymal cells derived from the neural crest never reverts to epithelia. Angiogenic mesenchyme, which is believed to arise from extraembryonic sources initially, is especially proliferative and capable of great migration within the embryo; it differentiates into endothelium throughout early development. A small subset of endothelial cells in the heart are induced to transform back to mesenchyme at the atrioventricular canal and the proximal outflow tract. This may be the only example of a mesenchymal population derived from an endothelial lineage ; the cells retain expression of an endothelial marker.
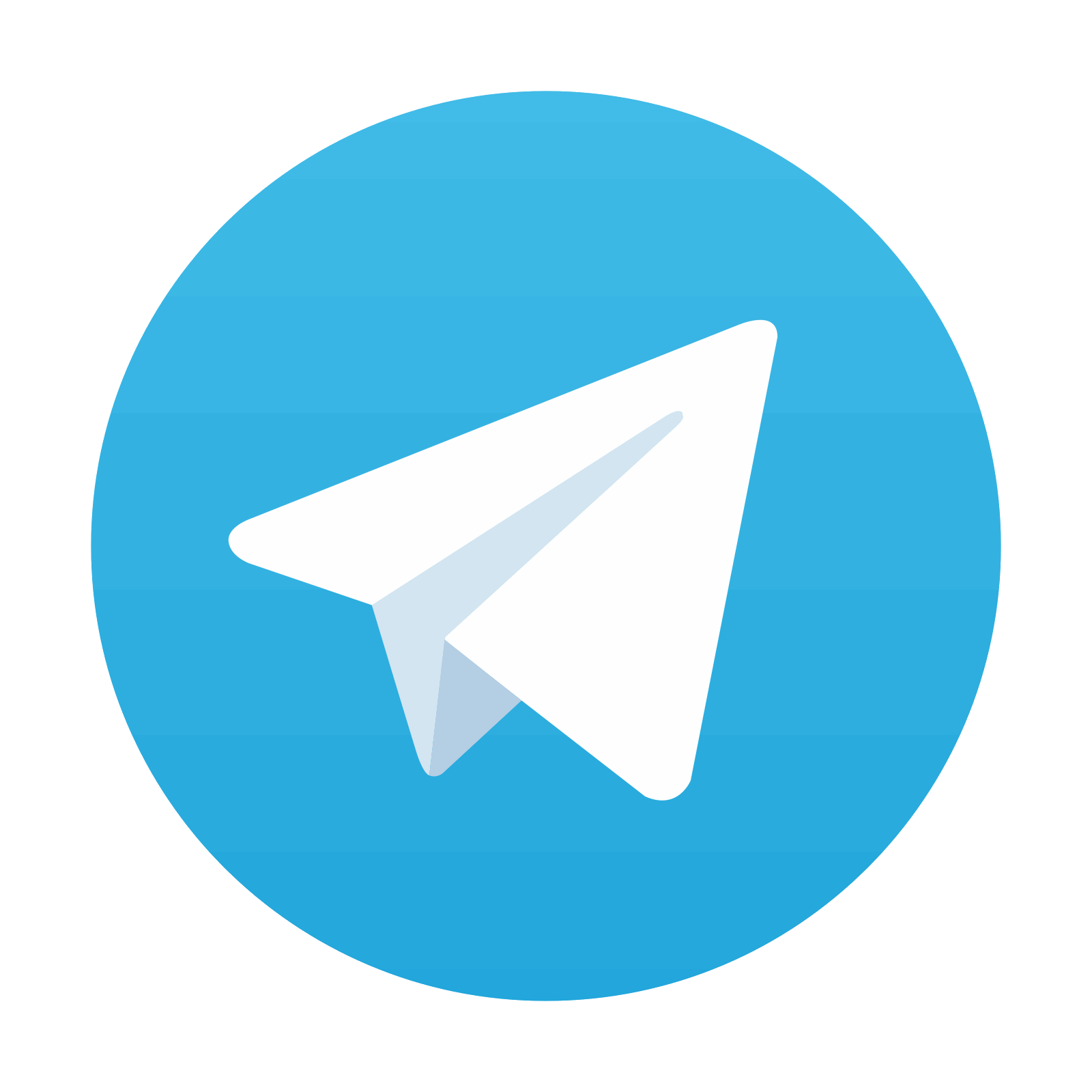
Stay updated, free articles. Join our Telegram channel

Full access? Get Clinical Tree
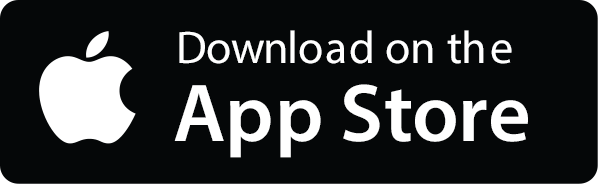
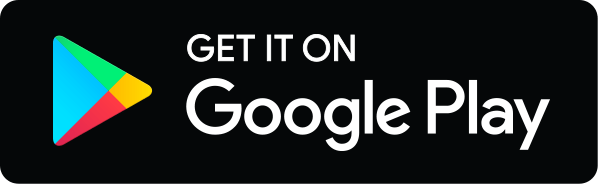