Overview
Cell-free DNA (cfDNA) screening has been rapidly introduced into prenatal care since it became clinically available in 2011. cfDNA screening can detect more than 99% of cases of trisomy 21 and also has high sensitivity and specificity for the detection of trisomy 13 and 18 and the common sex chromosome aneuploidies. cfDNA screens for aneuploidy by sequencing cfDNA from the maternal serum, which includes fetal cfDNA, largely derived from the placenta from apoptotic trophoblasts.
Pretest counseling should be performed for all women considering cfDNA screening, and all women with positive tests or test failures should receive posttest counseling. Confirmatory testing with chorionic villus sampling (CVS) or amniocentesis should be recommended to women with positive results as false-positive results occur and may be due to confined placental mosaicism (CPM) as well as other rare biologic causes including an underlying maternal chromosomal abnormality, a vanishing twin and in very rare cases, a maternal tumor or malignancy.
The entire fetal genome is represented in short cell-free fragments in the maternal plasma. Additional potential applications using cfDNA technology include screening for microdeletions, fetal Rh-determination, and detection of single gene disorders.
What Is Cell-Free DNA and How Is It Used for Screening for Fetal Aneuploidy?
cfDNA is increasingly being used to screen for the common fetal aneuploidies because of the higher sensitivity and specificities compared with conventional serum screening. Prenatal cfDNA screening was initially described in 1997 and became clinically available in 2011. The cfDNA in the maternal plasma consists of free-floating small fragments of maternal DNA and placental DNA. Placental cfDNA is primarily derived from apoptotic trophoblasts. It can be detected in maternal blood as early as 4 weeks’ gestation. The fetal fraction, which is the proportion of cfDNA of fetal (placental) origin to maternal cfDNA, exceeds 4% in the majority of pregnant women beginning at about 10 weeks’ gestation. It continues to increase by 0.1% per week through 21 weeks’ gestation and approximately 1% per week after 21 weeks’ gestation. Data suggest that a fetal fraction of at least 4% is needed to obtain a reliable result with cfDNA screening.
cfDNA screening is currently used to detect trisomies 21, 18, and 13; 45 X; and 47 XXX, XXY, and XYY. The American College of Medical Genetics and Genomics (ACMG) has endorsed the acronym NIPS (noninvasive prenatal screening) to emphasize the fact that cfDNA is a screening test and not a diagnostic test. In this chapter, we use the terminology “cell-free DNA” instead of “noninvasive prenatal screening,” as all current screening tests are considered noninvasive.
Placental cfDNA has a very short half-life; therefore, a cfDNA screening result will not be affected by a previous pregnancy. Screening is available after approximately 9–10 weeks of gestation when the fetal fraction is generally above 4%. cfDNA screening may be used either as a primary screening test or as a secondary screening test. Traditional serum screening (first trimester screening or second trimester maternal serum screening) is not recommended after cfDNA screening, and concurrent testing with cfDNA and traditional serum screening is also not recommended.
What Techniques Are Used in Cell-Free DNA Screening?
Several different methods of assaying cfDNA are currently in use for aneuploidy screening using next-generation sequencing technology: whole-genome sequencing (massively parallel sequencing), chromosome-selective (or targeted) sequencing, and single-nucleotide polymorphism (SNP) analysis. Although all the three methods appear to have similar sensitivities and specificities, there are advantages and limitations associated with each method.
Massively parallel sequencing randomly analyzes DNA from the entire genome. It sequences the entire DNA that is extracted from the maternal sample, counts cfDNA fragments, and assigns them to their specific chromosomal origin.
Targeted sequencing, also known as chromosome-specific sequencing, specifically amplifies chromosomal areas that are of interest and subsequently evaluates if there is an excess of one specific chromosome over another. An advantage of this technique is increased efficiency and overall lower cost (because only specific chromosomal areas are sequenced). A microarray-based technology that does not require next-generation sequencing has been used to quantify the relative contributions from specific chromosomes. This approach yielded high sensitivities and specificities that were comparable with next-generation sequencing approaches. This approach may result in additional decreased cost and turnaround time and testing of samples with a lower fetal fraction because of individual hybridization instead of sample multiplexing.
The third method uses a single-nucleotide polymorphism-based approach with analysis of the quantitative contributions of maternal and fetal DNA. The SNP-based approach cannot be used in pregnancies involving egg donors because this approach uses maternal genotypic information. This approach may identify consanguinity or uniparental disomy, in which both copies of a chromosome are inherited from the same parent.
Although the sensitivities and specificities for the detection of aneuploidy are similar for all three approaches, the whole genome sequencing approach is reported to have the lowest failure rate at 1.58%, followed by targeted sequencing at 3.56%, and SNP-based approach at 6.39%. The etiology of these failure rates is multifactorial and varies depending on quality control metrics, measurement of fetal fraction, and patient factors.
How Effective Is Cell-Free DNA in Screening for Fetal Aneuploidy?
cfDNA screening has a higher sensitivity and lower false-positive rate than conventional screening modalities (see Chapter 8 ) in detecting fetal aneuploidy. Clinical studies have demonstrated that the detection rates and false-positive rates for fetal aneuploidy do not differ with maternal age. The performance characteristics of cfDNA screening for fetal aneuploidy are summarized in Table 9.1 . A metaanalysis that included data from 35 studies reported cfDNA screening detection rates of 99.7% for trisomy 21, 97.9% for trisomy 18, and 99.0% for trisomy 13. The detection rates for sex chromosome aneuploidies were greater than 95% ( Table 9.1 ). Of note, it is important to recognize that the cases of fetal aneuploidy in women who did not receive a cfDNA test result were not included in the calculation of the detection rates. As expected, the positive predictive values (PPVs) for trisomy 21, 18, and 13 are higher in older women as the prevalence of these conditions increases with advancing maternal age.
Chromosomal Abnormality | Number of Affected Cases 8 | Detection Rate (%); 95% CI 8 | False-Positive Rate (%); 95% CI 8 | Positive Predictive Value a | |
---|---|---|---|---|---|
25-Year Old | 40-Year Old | ||||
Trisomy 21 | 1963 | 99.7 (99.1–99.9) | 0.04 (0.02–0.07) | 51% | 93% |
Trisomy 18 | 563 | 97.9 (94.9–99.1) | 0.04 (0.03–0.07) | 15% | 69% |
Trisomy 13 | 119 | 99.0 (65.8–100.0) | 0.04 (0.02–0.07) | 7% | 50% |
Monosomy X | 36 | 95.8 (70.3–99.5) | 0.14 (0.05–0.38) | 41% | 41% |
47 XXY 47 XYY 47 XXX | 17 b | 100.00 (83.6–97.8) b | 0.004 (0.0–0.08) b | 29% | 52% |
25% | 25% | ||||
27% | 45% |
a Positive predictive values obtained using PPV calculator from https://www.perinatalquality.org/Vendors/NSGC/NIPT/ .
What Is the Accuracy of Screening for Sex Chromosome Aneuploidies?
The performance characteristics are presented in Table 9.1 . Although the overall false-positive and false-negative rates for the detection of sex chromosome aneuploidies are very low, the PPV appears to be limited. Bianchi et al. reported a low frequency (0.26%–1.05%) of false-positive results for sex chromosome aneuploidy in a cohort of approximately 18,000 women who had cfDNA testing. Although clinical follow-up was only obtained for 44 of the 148 cases predicted to have monosomy X, 35 (79.5%) of the cases were false positives. Among 38 screen positive cases of XXX, 12 of 13 (92.3%) with clinical follow-up were false positive. Of the 12 false-positive cases, 2 were due to a maternal XXX cell line. Among six XYY cases, two had follow-up. One was confirmed as XYY and the other as XXY. Of the 12 XXY cases, 2 had follow-up and were confirmed to be true positives. These findings raise concerns about the limitations of cfDNA in screening for sex chromosome aneuploidy and highlight the importance of pretest counseling and confirmatory diagnostic testing following a screen-positive cfDNA test result. False-negative cases are likely to remain largely undetected because of the absence of fetal and neonatal findings in the most common sex chromosome aneuploidies. More information about performance characteristics of cfDNA for detection of sex chromosome aneuploidies will likely be forthcoming from future large prospective studies.
What Are the Potential Advantages and Disadvantages Associated With Cell-Free DNA Screening for Fetal Aneuploidy Compared With Conventional Screening Tests?
cfDNA is the most sensitive screening option for trisomy 13, 18, and 21. The false-positive rates associated with cfDNA screening are lower compared with conventional screening. Conventional screening tests (first trimester screening, second trimester screening, or stepwise sequential screening) have detection rates of 80%–95% with false-positive rates of 5%. This could potentially lead to an increased number of diagnostic procedures in women who have conventional screening compared with cfDNA screening.
In addition, the PPV (the likelihood that a woman with a positive test has an affected fetus) is considerably higher with cfDNA screening compared with conventional screening. In a study of approximately 1900 women with a mean age of 29.6 years, the PPVs for the detection of trisomy 21 were 45.5% with cfDNA screening and 4.2% with conventional screening.
An additional potential benefit of cfDNA is the option for earlier genetic screening results compared with some traditional screening methods. This allows for definitive diagnostic testing at an earlier gestational age.
cfDNA screening does not include screening for open neural tube defects. Patients should be offered MSAFP level at approximately 16–18 weeks’ gestation unless the fetal anatomy is adequately evaluated with a second trimester ultrasound by a provider with experience in the detection of neural tube defects, ventral wall defects, and other fetal disorders.
A study of a cohort of 1.3 million women who had traditional aneuploidy screening through the California State screening program showed that 506 (17%) of the 2993 women with a positive aneuploidy screen and an abnormal result on invasive diagnostic testing had chromosomal abnormalities that would not be detectable with cfDNA screening. The abnormalities characterized as undetectable by cfDNA screening included mosaic cases of trisomy 21, trisomy 18 and trisomy 13, mosaic sex chromosome aneuploidies, other trisomies (nonmosaic and mosaic), balanced rearrangements, unbalanced rearrangements, insertions and deletions, triploidy, tetraploidy, and cases with extra structurally abnormal chromosomes. There were 45 cases with CPM. Of note, the clinical outcomes associated with these abnormalities ranged from mild to having a significant disability. Some of the undetected abnormalities including the triploidy cases and the tetraploidy case would likely have resulted in spontaneous pregnancy loss, and the fetuses with balanced translocations would likely have been phenotypically normal. Of note, although cfDNA screening currently does not report on the detection of rare autosomal trisomies, it is possible to detect these abnormalities using this technology. However, with present methods, traditional multiple marker screening is a broader screen for the detection of fetal anomalies compared with the high precision of cfDNA screening. Large-scale prospective studies are needed to evaluate the clinical validity and utility of reporting information on the rare autosomal trisomies.
A decision analytic model comparing clinical outcomes, quality-adjusted life-years and costs associated with different strategies for Down syndrome testing using cost estimates from 2015 determined that cfDNA is the optimal and most cost-effective screen for women of 40 years and older. The authors assessed the outcomes of six testing strategies incorporating diagnostic testing with chromosomal microarray, multiple marker screening, cfDNA screening, and nuchal translucency screening alone, in combination, or in sequence. Multiple marker screening with optional follow-up diagnostic testing was the most effective (highest quality-adjusted life-years) and least expensive strategy at ages of 20–38 years.
What Are Other Potential Uses for Cell-Free DNA in Prenatal Testing?
Determination of Fetal Sex
A metaanalysis involving 60 studies (11,179 cfDNA tests) reported a sensitivity of 98.9% (95% CI 0.980–0.994) and a specificity of 99.6% (95% CI 0.989–0.998) for the detection of fetal sex. Determination of fetal sex allows for refinement of risk for couples at increased risk of having a child with an X-linked recessive condition. Female carriers of X-linked recessive disorders have a 50% risk of transmitting the mutant allele to their male offspring, and thus, having an affected son. Females, themselves, generally are not significantly affected with X-linked recessive disorders because of the protection from a normal X chromosome and lyonization (see Chapter 1 ). Common X-linked recessive disorders include Duchenne muscular dystrophy, hemophilia, and fragile X Syndrome.
Detection of Microdeletions
cfDNA screening panels have been extended by some laboratories to include microdeletion syndromes including 22q11 deletion syndrome (DiGeorge syndrome), Wolf–Hirschhorn syndrome (4p deletion), Cri-du-chat (5p minus syndrome), 1p36 deletion syndrome, and Prader Willi and Angelman syndromes, which both may result from a 15q deletion. Because of the low prevalence of these conditions and the low number of plasma samples available from affected pregnancies to help validate the testing, there is a paucity of data on the performance characteristics (sensitivity and specificity, positive predictive value, and false-positive and false-negative results) associated with screening for microdeletion syndromes.
22q11.2 deletion syndrome is the most frequent microdeletion syndrome, occurring in 1/3000–6000 live births. Approximately, 5%–10% of cases of prenatally diagnosed congenital heart defects have 22q11.2 deletions, depending on the specific defect. Conotruncal defects including Tetralogy of Fallot, truncus arteriosus, and interrupted aortic arch are associated with 22q11.2 deletion syndrome. Prenatal diagnosis of 22q11.2 deletion syndrome may reduce the associated early mortality and morbidity and potentially reduce medical, emotional, and financial costs. Although diagnostic testing with chromosomal microarray analysis is recommended when a fetal heart defect or other structural abnormality is identified on early prenatal ultrasound, cell-free DNA screening for microdeletions including 22q11.2 deletion syndrome may be an option for patients who would not consider invasive prenatal diagnostic testing. Patients with fetal structural abnormalities detected at an advanced gestational age may opt for cfDNA screening including microdeletions as a means of avoiding the risk associated with amniocentesis but potentially influencing decisions including delivery location to optimize neonatal care. In these situations, it should be made clear that cfDNA screening is not diagnostic, that the false-positive and false-negative rates are not known, and that diagnostic testing will likely be performed after delivery.
Based on the low prevalence of the microdeletion syndromes, the PPVs are anticipated to be low. Furthermore, it is possible that screening for microdeletion syndromes may increase the overall false-positive rate and potentially lead to an increased rate of invasive diagnostic testing. The American College of Obstetricians and Gynecologists (ACOG) and Society for Maternal-Fetal Medicine (SMFM) do not endorse routine cfDNA screening for microdeletion syndromes due to a lack of clinical validation.
Wapner and colleagues reported a 1.7% (1 in 60) incidence of clinically relevant microdeletions and microduplications in a cohort of 3118 pregnancies sampled for indications other than fetal structural abnormalities. Women who desire testing for microdeletions (copy number variants) should be offered diagnostic testing with chromosomal microarray. This may be performed by CVS or amniocentesis (see Chapter 14 ).
Single Gene Disorders (see Chapter 1 )
cfDNA to screen for single gene disorders has been reported and is available clinically in some laboratories. Initial cfDNA testing for single gene disorders focused on conditions in which the mother did not carry the mutant allele. This included screening for some autosomal dominant disorders in which the variant is carried on the paternal allele, de novo autosomal dominant disorders in which a new variant occurs in the offspring, and autosomal recessive conditions in cases where the parents carry different variants in the affected gene. The diagnosis in these cases was based on detection or exclusion of the paternally inherited allele. Examples of single gene disorders potentially amenable to cfDNA screening include achondroplasia, Noonan syndrome, osteogenesis imperfecta, craniosynostosis syndromes, Rett syndrome, myotonic dystrophy, neurofibromatosis type 1, cystic fibrosis, and congenital adrenal hyperplasia. De novo autosomal dominant disorders are associated with advanced paternal age. The risk for de novo autosomal dominant mutations is 0.3%–0.5% among the offspring of fathers aged greater than 40 years, which is comparable with the risk of Down syndrome among the offspring of 35- to 40-year-old mothers. Panels to screen for such de novo autosomal dominant disorders are available, but the clinical utility has not been proven and use of such panels is not routinely recommended.
Advances in digital polymerase chain reaction (PCR) and next-generation DNA sequencing technology have made it possible to discriminate affected from unaffected fetuses by determining the relative contribution of the normal and abnormal allele in cases including those involving inheritance of a maternal mutant allele. This has made it possible to detect X-linked recessive and additional autosomal recessive conditions using cfDNA.
Concerns regarding the use of cfDNA in the diagnosis of single gene disorders include the costs of testing, as well as the potential for disparities in access among women of lower socioeconomic groups. Many of these genetic diseases are rare and require individualized testing approaches developed on a family-specific basis. Use of cfDNA for the diagnosis of single gene disorders has not been endorsed by ACOG, SMFM, or ACMG to date.
RhD Determination
cfDNA testing can be used to screen for fetal RhD status in RhD-negative women. Fetal RhD genotyping can be performed as early as 10 weeks of gestation. RhD detection is based on real-time PCR of cfDNA that distinguishes fetal RhD sequences from the maternal plasma. Mackie et al. conducted a bivariate metaanalysis that showed that fetal RhD genotyping with cfDNA is highly sensitive (99.3%) and specific (98.4%) with low false-negative results. In general, earlier testing with cfDNA is preferred because 40% of Rh-negative women will carry an Rh-negative fetus. Identification of RhD-negative fetuses could potentially save unnecessary administration of Rh immune globulin in unsensitized women and avoid screening for fetal anemia (i.e., serial middle cerebral artery Doppler assessments) and unnecessary invasive procedures (i.e., amniocentesis and intrauterine transfusion) in sensitized women. cfDNA determination of fetal RhD status is used in the first trimester in several European countries, where Rh immune globulin is less readily available, as well as in Canada, and may prove to be a cost-effective approach in the management of RhD-negative women in the United States. Future cost-effective studies are indicated.
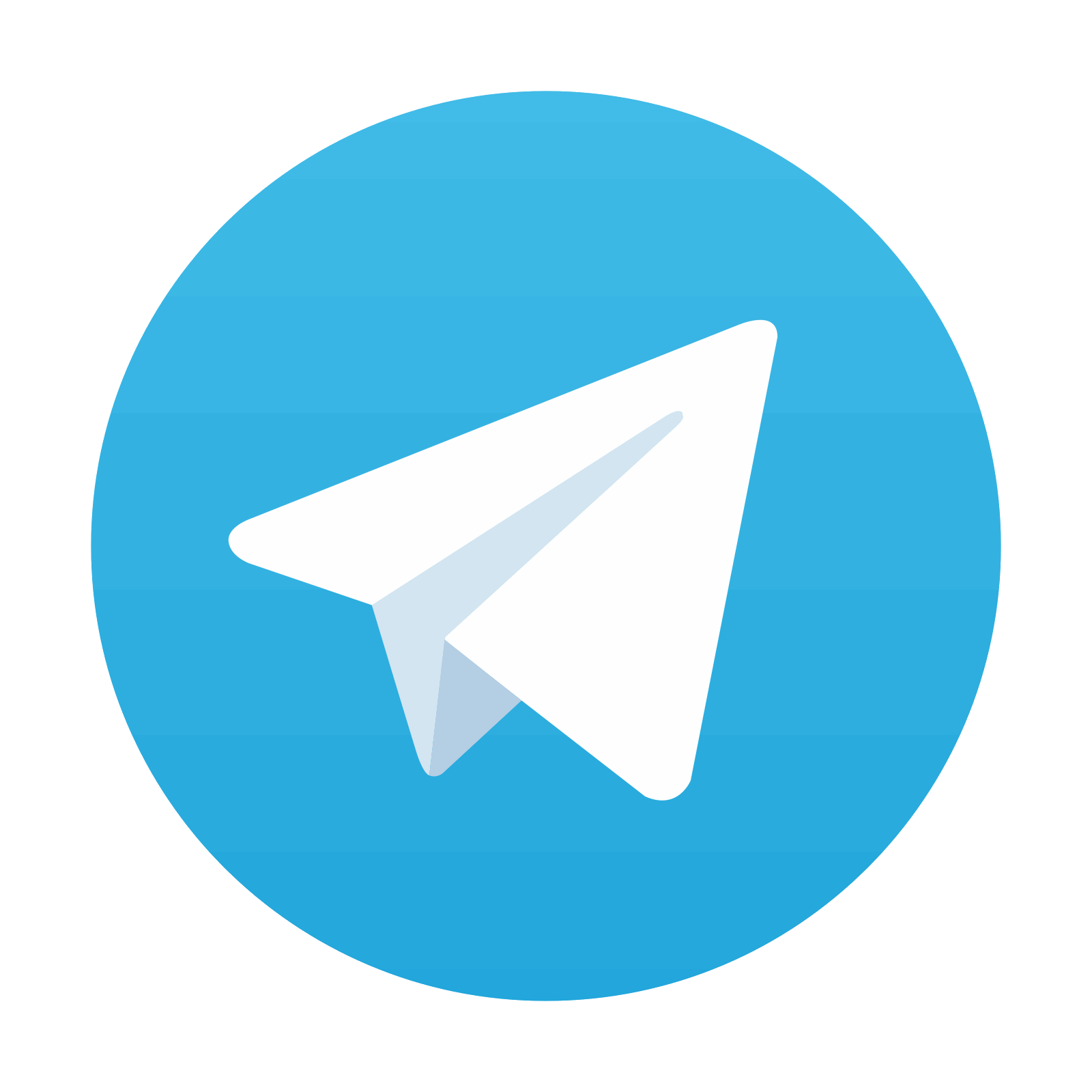
Stay updated, free articles. Join our Telegram channel

Full access? Get Clinical Tree
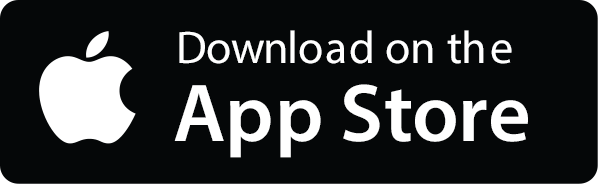
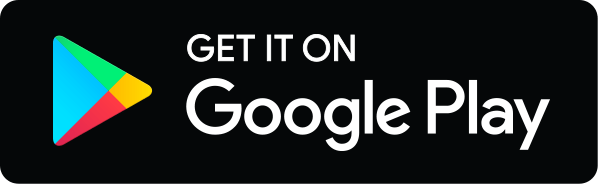