PHYSICAL EXAMINATION
General
The examination begins with a visual assessment of mental status, signs of distress, perfusion, and skin color. Documentation of heart rate, respiratory rate, blood pressure (in all four extremities), and oxygen saturation is essential. Many congenital cardiac defects occur as part of a genetic syndrome (Table 20–2), and complete assessment includes evaluation of dysmorphic features that may be clues to the associated cardiac defect.
Table 20–2. Cardiac defects in common syndromes.
Cardiovascular Examination
A. Inspection and Palpation
Chest conformation should be noted in the supine position. A precordial bulge indicates cardiomegaly. Palpation may reveal increased precordial activity, right ventricular lift, or left-sided heave; a diffuse point of maximal impulse; or a precordial thrill caused by a grade IV/VI or greater murmur. The thrill of aortic stenosis is found in the suprasternal notch. In patients with severe pulmonary hypertension, a palpable pulmonary closure (P2) is frequently noted at the upper left sternal border.
B. Auscultation
1. Heart sounds—The first heart sound (S1) is the sound of atrioventricular (AV) valve closure. It is best heard at the lower left sternal border and is usually medium-pitched. Although S1 has multiple components, only one of these (M1—closure of the mitral valve) is usually audible.
The second heart sound (S2) is the sound of semilunar valve closure. It is best heard at the upper left sternal border. S2 has two component sounds, A2 and P2 (aortic and pulmonic valve closure). Splitting of S2 varies with respiration, widening with inspiration and narrowing with expiration. Abnormal splitting of S2 may be an indication of cardiac disease (Table 20–3). A prominent or loud P2 is associated with pulmonary hypertension.
Table 20–3. Abnormal splitting of S2.
The third heart sound (S3) is the sound of rapid left ventricular filling. It occurs in early diastole, after S2, and is medium- to low-pitched. In healthy children, S3 diminishes or disappears when going from supine to sitting or standing. A pathologic S3 is often heard in the presence of poor cardiac function or a large left-to-right shunt. The fourth heart sound (S4) is associated with atrial contraction and increased atrial pressure, and has a low pitch similar to that of S3. It occurs just prior to S1 and is not normally audible. It is heard in the presence of atrial contraction into a noncompliant ventricle as in hypertrophic or restrictive cardiomyopathy or from other causes of diastolic dysfunction.
Ejection clicks are usually related to dilate great vessels or valve abnormalities. They are heard during ventricular systole and are classified as early, mid, or late. Early ejection clicks at the mid left sternal border are from the pulmonic valve. Aortic clicks are typically best heard at the apex. In contrast to aortic clicks, pulmonic clicks vary with respiration, becoming louder during inspiration. A mid to late ejection click at the apex is most typically caused by mitral valve prolapse.
2. Murmurs—A heart murmur is the most common cardiovascular finding leading to a cardiology referral. Innocent or functional heart murmurs are common, and 40%–45% of children have an innocent murmur at some time during childhood.
A. CHARACTERISTICS—All murmurs should be described based on the following characteristics:
(1) Location and radiation—Where the murmur is best heard and where the sound extends.
(2) Relationship to cardiac cycle and duration—Systolic ejection (immediately following S1 with a crescendo/decrescendo change in intensity), pansystolic (occurring throughout most of systole and of constant intensity), diastolic, or continuous. The timing of the murmur provides valuable clues as to underlying pathology (Table 20–4).
Table 20–4. Pathologic murmurs.
(3) Intensity—Grade I describes a soft murmur heard with difficulty; grade II, soft but easily heard; grade III, loud but without a thrill; grade IV, loud and associated with a precordial thrill; grade V, loud, with a thrill, and audible with the edge of the stethoscope; grade VI, very loud and audible with the stethoscope off the chest.
(4) Quality—Harsh, musical, or rough; high, medium, or low in pitch.
(5) Variation with position—Audible changes in murmur when the patient is supine, sitting, standing, or squatting.
B. INNOCENT MURMURS—The six most common innocent murmurs of childhood are
(1) Newborn murmur—Heard in the first few days of life, this murmur is at the lower left sternal border, without significant radiation. It has a soft, short, vibratory grade I–II/VI quality that often subsides when mild pressure is applied to the abdomen. It usually disappears by age 2–3 weeks.
(2) Peripheral pulmonary artery stenosis (PPS)—This murmur, often heard in newborns, is caused by the normal branching of the pulmonary artery. It is heard with equal intensity at the upper left sternal border, at the back, and in one or both axillae. It is a soft, short, high-pitched, grade I–II/VI systolic ejection murmur and usually disappears by age 2. This murmur must be differentiated from true peripheral pulmonary stenosis (Williams syndrome, Alagille syndrome, or rubella syndrome), coarctation of the aorta, and valvular pulmonary stenosis. Characteristic facial features, extracardiac physical exam findings, history, and laboratory abnormalities suggestive of the syndromes listed above are the best way to differentiate true peripheral pulmonary stenosis from benign PPS of infancy as the murmurs can be similar.
(3) Still murmur—This is the most common innocent murmur of early childhood. It is typically heard between 2 and 7 years of age. It is the loudest midway between the apex and the lower left sternal border. Still murmur is a musical or vibratory, short, high-pitched, grade I–III early systolic murmur. It is loudest when the patient is supine. It diminishes or disappears with inspiration or when the patient is sitting. The Still murmur is louder in patients with fever, anemia, or sinus tachycardia from any reason.
(4) Pulmonary ejection murmur—This is the most common innocent murmur in older children and adults. It is heard from age 3 years onward. It is usually a soft systolic ejection murmur, grade I–II in intensity at the upper left sternal border. The murmur is louder when the patient is supine or when cardiac output is increased. The pulmonary ejection murmur must be differentiated from murmurs of pulmonary stenosis, coarctation of the aorta, atrial septal defect (ASD), and peripheral pulmonary artery stenosis.
(5) Venous hum—A venous hum is usually heard after age 2 years. It is located in the infraclavicular area on the right. It is a continuous musical hum of grade I–III intensity and may be accentuated in diastole and with inspiration. It is best heard in the sitting position. Turning the child’s neck, placing the child supine, and compressing the jugular vein obliterates the venous hum. Venous hum is caused by turbulence at the confluence of the subclavian and jugular veins.
(6) Innominate or carotid bruit—This murmur is more common in the older child and adolescents. It is heard in the right supraclavicular area. It is a long systolic ejection murmur, somewhat harsh and of grade II–III intensity. The bruit can be accentuated by light pressure on the carotid artery and must be differentiated from all types of aortic stenosis. The characteristic findings of aortic stenosis are outlined in more detail later in this chapter.
When innocent murmurs are found in a child, the physician should assure the parents that these are normal heart sounds of the developing child and that they do not represent heart disease.
Extracardiac Examination
A. Arterial Pulse Rate and Rhythm
Cardiac rate and rhythm vary greatly during infancy and childhood, so multiple determinations should be made. This is particularly important for infants (Table 20–5) whose heart rates vary with activity. The rhythm may be regular or there may be a normal phasic variation with respiration (sinus arrhythmia).
Table 20–5. Resting heart rates.
B. Arterial Pulse Quality and Amplitude
A bounding pulse is characteristic of run-off lesions, including patent ductus arteriosus (PDA), aortic regurgitation, arteriovenous malformation, or any condition with a low diastolic pressure (fever, anemia, or septic shock). Narrow or thready pulses occur in patients with conditions reducing cardiac output such as decompensated heart failure pericardial tamponade, or severe aortic stenosis. A reduction in pulse amplitude or blood pressure (> 10 mm Hg) with inspiration is referred to as pulsus paradoxus and is a telltale sign of pericardial tamponade. The pulses of the upper and lower extremities should be compared. The femoral pulse should be palpable and equal in amplitude and simultaneous with the brachial pulse. A femoral pulse that is absent or weak, or that is delayed in comparison with the brachial pulse, suggests coarctation of the aorta.
C. Arterial Blood Pressure
Blood pressures should be obtained in the upper and lower extremities. Systolic pressure in the lower extremities should be greater than or equal to that in the upper extremities. The cuff must cover the same relative area of the arm and leg. Measurements should be repeated several times. A lower blood pressure in the lower extremities suggests coarctation of the aorta.
D. Cyanosis of the Extremities
Cyanosis results from an increased concentration (> 4–5 g/dL) of reduced hemoglobin in the blood. Bluish skin color is usually, but not always, a sign. Visible cyanosis also accompanies low cardiac output, hypothermia, and systemic venous congestion, even in the presence of adequate oxygenation. Cyanosis should be judged by the color of the mucous membranes (lips). Bluish discoloration around the mouth (acrocyanosis) does not correlate with cyanosis.
E. Clubbing of the Fingers and Toes
Clubbing is often associated with severe cyanotic congenital heart disease. It usually appears after age 1 year. Hypoxemia with cyanosis is the most common cause, but clubbing also occurs in patients with endocarditis, chronic liver disease, inflammatory bowel diseases, chronic pulmonary disease, and lung abscess. Digital clubbing may be a benign genetic variant.
F. Edema
Edema of dependent areas (lower extremities in the older child and the face and sacrum in the younger child) is characteristic of elevated right heart pressure, which may be seen with tricuspid valve pathology or heart failure.
G. Abdomen
Hepatomegaly is the cardinal sign of right heart failure in the infant and child. Left heart failure can ultimately lead to right heart failure and therefore, hepatomegaly may also be seen in the child with pulmonary edema from lesions causing left-to-right shunting (pulmonary overcirculation) or left ventricular failure. Splenomegaly may be present in patients with long-standing heart failure (HF), and is also a characteristic of infective endocarditis. Ascites is a feature of chronic right heart failure. Examination of the abdomen may reveal shifting dullness or a fluid wave.
Finley JP et al: Assessing children’s heart sounds at a distance with digital recordings. Pediatrics 2006;118:2322–2325 [PMID: 17142514].
Mahnke CB et al: Utility of store-and-forward pediatric telecardiology evaluation in distinguishing normal from pathologic pediatric heart sounds. Clin Pediatr (Phila) 2008;47:919–925 [PMID: 18626106].
Markel H: The stethoscope and the art of listening. N Engl J Med 2006;354:551–553 [PMID: 16467541].
ELECTROCARDIOGRAPHY
The electrocardiogram (ECG) is essential in the evaluation of the cardiovascular system. The heart rate should first be determined, then the cardiac rhythm (Is the patient in a normal sinus rhythm or other rhythm as evidenced by a P wave with a consistent PR interval before every QRS complex?), and then the axis (Are the P and QRS axes normal for patient age?). Finally, assessment of chamber enlargement, cardiac intervals, and ST segments should be performed.
Age-Related Variations
The ECG evolves with age. The heart rate decreases and intervals increase with age. RV dominance in the newborn changes to LV dominance in the older infant, child, and adult. The normal ECG of the 1-week-old infant is abnormal for a 1-year-old child, and the ECG of a 5-year-old child is abnormal for an adult.
Electrocardiographic Interpretation
Figure 20–1 defines the events recorded by the ECG.
Figure 20–1 Complexes and intervals of the electrocardiogram.
A. Rate
The heart rate varies markedly with age, activity, and state of emotional and physical well-being (Table 20-5).
B. Rhythm
Sinus rhythm should always be present in healthy children. Extra heart beats representing premature atrial and ventricular contractions are common during childhood, with atrial ectopy predominating in infants and ventricular ectopy during adolescence. Isolated premature beats in patients with normal heart structure and function are usually benign.
C. Axis
1. P-wave axis—The P wave is generated from atrial contraction beginning in the high right atrium at the site of the sinus node. The impulse proceeds leftward and inferiorly, thus leading to a positive deflection in all left-sided and inferior leads (II, III, and aVF) and negative in lead aVR.
2. QRS axis—The net voltage should be positive in leads I and aVF in children with a normal axis. In infants and young children, RV dominance may persist, leading to a negative deflection in lead I. Several congenital cardiac lesions are associated with alterations in the normal QRS axis (Table 20–6).
Table 20–6. QRS axis deviation.
D. P Wave
In the pediatric patient, the amplitude of the P wave is normally no greater than 3 mm and the duration no more than 0.08 second. The P wave is best seen in leads II and V1.
E. PR Interval
The PR is measured from the beginning of the P wave to the beginning of the QRS complex. It increases with age and with slower rates. The PR interval ranges from a minimum of 0.10 second in infants to a maximum of 0.18 second in older children with slow rates. Rheumatic heart disease, digitalis, β-blockers and calcium channel blockers can prolong the PR interval.
F. QRS Complex
This represents ventricular depolarization, and its amplitude and direction of force (axis) reveal the relative ventricular mass in hypertrophy, hypoplasia, and infarction. Abnormal ventricular conduction (eg, right or left bundle-branch block) is also revealed.
G. QT Interval
This interval is measured from the beginning of the QRS complex to the end of the T wave. The QT duration may be prolonged as a primary condition or secondarily due to drugs or electrolyte imbalances (Table 20–7). The normal QT duration is rate-related and must be corrected using the Bazett formula:
Table 20–7. Causes of QT prolongation.a
The normal QTc is less than or equal to 0.44 second.
H. ST Segment
This segment, lying between the end of the QRS complex and the beginning of the T wave, is affected by drugs, electrolyte imbalances, or myocardial injury.
I. T Wave
The T wave represents myocardial repolarization and is altered by electrolytes, myocardial hypertrophy, and ischemia.
J. Impression
The ultimate impression of the ECG is derived from a systematic analysis of all the features above as compared with expected normal values for the child’s age.
O’Connor M, McDaniel N, Brady WJ: The pediatric electrocardiogram. Part I: age-related interpretation. Am J Emerg Med 2008 May:26(4):506–512 [PMID: 18416018].
CHEST RADIOGRAPH
Evaluation of the chest radiograph for cardiac disease should focus on (1) position of the heart, (2) position of the abdominal viscera, (3) cardiac size, (4) cardiac configuration, and (5) character of the pulmonary vasculature. The standard posteroanterior and left lateral chest radiographs are used (Figure 20–2).
Figure 20–2. Position of cardiovascular structures in principal radiograph views. AO, aorta; IVC, inferior vena cava; LA, left atrium; LA APP, left atrial appendage; LV, left ventricle; PA, pulmonary artery; RA, right atrium; RV, right ventricle; SVC, superior vena cava.
Cardiac position is either levocardia (heart predominantly in the left chest), dextrocardia (heart predominantly in the right chest), or mesocardia (midline heart). The position of the liver and stomach bubble is either in the normal position (abdominal situs solitus), inverted with the stomach bubble on the right (abdominal situs inversus), or variable with midline liver (abdominal situs ambiguous). The heart appears relatively large in normal newborns at least in part due to a prominent thymic shadow. The heart size should be less than 50% of the chest diameter in children older than age 1 year. The cardiac configuration on chest radiograph may provide useful diagnostic information (Table 20–8). Some congenital cardiac lesions have a characteristic radiographic appearance that suggests the diagnosis but should not be viewed as conclusive (Table 20–9). The pulmonary vasculature should be assessed. The presence of increased or decreased pulmonary blood flow suggests a possible congenital cardiac diagnosis, particularly in the cyanotic infant (Table 20–10).
Table 20–8. Radiographic changes with cardiac chamber enlargement.
Table 20–9. Lesion-specific chest radiographic findings.
Table 20–10. Alterations in pulmonary blood flow in cyanotic cardiac lesions.
Laya BF et al: The accuracy of chest radiographs in the detection of congenital heart disease and in the diagnosis of specific congenital cardiac lesions. Pediatr Radiol 2006;36:677–681 [PMID: 16547698].
Dextrocardia
Dextrocardia is a radiographic term used when the heart is on the right side of the chest. When dextrocardia occurs with reversal of position of the other important organs of the chest and abdomen (eg, liver, lungs, and spleen), the condition is called situs inversus totalis, and the heart is usually normal. When dextrocardia occurs with the other organs normally located (situs solitus), the heart usually has severe defects.
Other situs abnormalities include situs ambiguous with the liver central and anterior in the upper abdomen and the stomach pushed posteriorly; bilateral right-sidedness (asplenia syndrome); and bilateral left-sidedness (polysplenia syndrome). In virtually all cases of situs ambiguous, congenital heart disease is present.
ECHOCARDIOGRAPHY
Echocardiography is a fundamental tool of pediatric cardiology. Using multiple ultrasound modalities (two-dimensional imaging, Doppler, and M-mode), cardiac anatomy, blood flow, intracardiac pressures, and ventricular function can be assessed. Echocardiography is based on the physical principles of sound waves. The ultrasound frequencies utilized in cardiac imaging range from 2 to 10 million cycles/s.
M-mode echocardiography uses short bursts of ultrasound sent from a transducer. At acoustic interfaces, sound waves are reflected back to the transducer. The time it takes for the sound wave to return to the transducer is measured and the distance to the interface is calculated. That calculated distance is displayed against time, and a one-dimensional image is constructed that demonstrates cardiac motion. Two-dimensional imaging extends this technique by sending a rapid series of ultrasound bursts across a 90-degree sector, which allows construction of a two-dimensional image of the heart. Doppler ultrasound measures blood flow. The ultrasound transducer sends out a known frequency of sound which reflects off moving red blood cells. The transducer receives the reflected frequency and compares it with the transmitted frequency. The blood flow velocity can be calculated from the measured frequency shift. This information is used to estimate pressure gradients by the simplified Bernouli equation, in which the pressure gradient is equal to four times the calculated velocity (Pressure gradient = 4(V2)).
A transthoracic echocardiogram is obtained by placing the transducer on areas of the chest where there is minimal lung interference. At each transducer position, the beam is swept through the heart and a two-dimensional image appears on the screen. Complex intracardiac anatomy and spatial relationships can be described, making possible the accurate diagnosis of congenital heart disease. In addition to structural details, Doppler gives information about intracardiac blood flow and pressure gradients. Commonly used Doppler techniques include color flow imaging, pulsed-wave, and continuous-wave Doppler. Color flow imaging gives general information on the direction and velocity of flow. Pulsed- and continuous-wave Doppler imagings give more precise measurements of blood velocity. The role of M-mode in the ultrasound examination has decreased as other ultrasound modalities have been developed. M-mode is still used to measure LV end-diastolic and end-systolic dimensions and permits calculation of the LV-shortening fraction, a standard estimate of LV function (SF = LV end-diastolic volume – LV systolic volume/LV end-diastolic volume). Three-dimensional echocardiography, tissue Doppler, strain, and strain rate imaging are newer modalities that provide more sophisticated assessment of systolic and diastolic function and can detect early changes in myocardial function.
A typical transthoracic echocardiogram performed by a skilled sonographer takes about 30 minutes, and patients must be still for the examination. Frequently infants and children cannot cooperate for the examination and sedation is required. Transesophageal echocardiography requires general anesthesia in infants and children and is primarily used to guide interventional procedures and surgical repair of congenital heart disease. In cases of difficult imaging windows due to patient size, air interference or when looking for evidence of vegetations on cardiac valves, transesophageal echocardiography may be necessary.
It is important to note that fetal echocardiography plays an important role in the prenatal diagnosis of congenital heart disease. A fetal echocardiogram is recommended if the fetus is considered high risk for the development of congenital heart disease or if there is suspicion for structural heart disease or fetal arrhythmias based on the obstetric fetal ultrasound. In utero management of fetal arrhythmias and post-delivery planning for the fetus with complex heart disease has resulted in improved outcomes for this challenging group of patients.
Elkiran O et al: Tissue Doppler, strain, and strain rate measurements assessed by two-dimensional speckle-tracking echocardiography in healthy newborns and infants. Cardiol Young 2013 Feb 6:1–11 [PMID: 23388082].
Friedberg MK et al: Validation of 3D echocardiographic assessment of left ventricular volumes, mass, and ejection fraction in neonates and infants with congenital heart disease: a comparison study with cardiac MRI. Circ Cardiovasc Imaging 2010;3(6):735–742 [PMID: 20855861].
NUCLEAR CARDIOLOGY
Nuclear imaging is not commonly used in pediatric cardiology, but can be a useful adjunct to cardiopulmonary exercise testing in assessing both fixed and reversible areas of myocardial ischemia. It is valuable in evaluating myocardial perfusion in patients with Kawasaki disease, repaired anomalous left coronary artery or other coronary anomalies, myocardial bridging in the setting of hypertrophic cardiomyopathy (HCM), or chest pain in association with ECG changes with exercise.
MAGNETIC RESONANCE IMAGING
Magnetic resonance imaging (MRI) of the heart is valuable for evaluation and noninvasive follow-up of many congenital heart defects. It is particularly useful in imaging the thoracic vessels, which are difficult to image by transthoracic echocardiogram. Cardiac gated imaging allows dynamic evaluation of structure and blood flow of the heart and great vessels. Cardiac MRI provides unique and precise imaging in patients with newly diagnosed or repaired aortic coarctation and defines the aortic dilation in Marfan, Turner, and Loeys-Dietz syndromes. Cardiac MRI can quantify regurgitant lesions such as pulmonary insufficiency (PI) after repair of tetralogy of Fallot (ToF) and can define ventricular function, chamber size, and wall thickness in patients with inadequate echocardiographic images or cardiomyopathies. MRI is especially useful to characterize right ventricular size and function as this chamber is often difficult to image comprehensively by echocardiogram. Because it allows computer manipulation of images of the heart and great vessels, three-dimensional MRI is an ideal noninvasive way of obtaining accurate reconstructions of the heart. General anesthesia is often required to facilitate cardiac MRI performance in children less than 8 years.
Van der Hulst AE et al: Cardiac MRI in postoperative congenital heart disease patients. J Magn Reson Imaging 2012;36(3):511–528 [PMID: 2290365].
CARDIOPULMONARY STRESS TESTING
Most children with heart disease are capable of normal activity. Data on cardiac function after exercise are essential to prevent unnecessary restriction of activities. The response to exercise is helpful in determining the need for and the timing of cardiovascular surgery and is a useful objective outcome measure of the results of medical and surgical interventions.
Bicycle ergometers or treadmills can be used in children as young as age 5 years. The addition of a metabolic cart enables one to assess whether exercise impairment is secondary to cardiac limitation, pulmonary limitation, deconditioning, or lack of effort. Exercise variables include the ECG, blood pressure response to exercise, oxygen saturation, ventilation, maximal oxygen consumption, and peak workload attained. Cardiopulmonary stress testing is routine in children with congenital cardiac lesions to ascertain limitations, develop exercise programs, assess the effect of therapies, and decide on the need for cardiac transplantation. Stress testing is also employed in children with structurally normal hearts with complaints of exercise-induced symptoms in order to rule out cardiac or pulmonary pathology. Significant stress ischemia or dysrhythmias warrant physical restrictions or appropriate therapy. Children with poor performance due to suboptimal conditioning benefit from a planned exercise program.
Arvidsson D, Slinde F, Hulthén L, Sunnegårdh J: Physical activity, sports participation and aerobic fitness in children who have undergone surgery for congenital heart defects. Acta Paediatr 2009 Sep;98(9):1475–1482 [PMID: 19489769].
ARTERIAL BLOOD GASES
Quantitating the partial oxygen pressure (Po2) or O2 saturation during the administration of 100% oxygen is the most useful method of distinguishing cyanosis produced primarily by heart disease or by lung disease in sick infants. In cyanotic heart disease, the partial arterial oxygen pressure (Pao2) increases very little when 100% oxygen is administered over the values obtained while breathing room air. However, Pao2 usually increases significantly when oxygen is administered to a patient with lung disease. Table 20–11 illustrates the responses seen in patients with heart or lung disease during the hyperoxic test. Although the US Department of Health and Human Services recommended newborn screening for critical congenital heart disease in 2010, implementation of comprehensive pulse oximetry screening programs are challenged by variable infrastructure and access to expert interpretation.
Table 20–11. Examples of responses to 10 minutes of 100% oxygen in lung disease and heart disease.
Kember AR et al: Strategies for implementing screening for critical congenital heart disease. Pediatrics 2011 Nov;128(5):e1259–e1267 [PMID: 21987707].
CARDIAC CATHETERIZATION & ANGIOCARDIOGRAPHY
Cardiac catheterization is an invasive method to evaluate anatomic and physiologic conditions in congenital or acquired heart disease. Management decisions may be made based on oximetric, hemodynamic or angiographic data obtained through a catheterization. In an increasing number of cases, intervention may be performed during a catheterization that may palliate, or even cure, a congenital heart defect without open heart surgery.
Cardiac Catheterization Data
Figure 20–3 shows oxygen saturation (in percent) and pressure (in millimeters of mercury) values obtained at cardiac catheterization from the chambers and great arteries of the heart. These values represent the normal range for a school age child.
Figure 20–3 Pressures (in millimeters of mercury) and oxygen saturation (in percent) obtained by cardiac catheterization in a healthy child. 3, mean pressure of 3 mm Hg in the right atrium; 5, mean pressure of 5 mm Hg in the left atrium.
A. Oximetry, Shunts, and Cardiac Output
Measurement of oxygen levels throughout the heart and surrounding blood vessels can provide a wealth of information about a patient’s physiology. The difference between systemic saturation (in the aorta) and mixed venous saturation (usually in the superior vena cava [SVC]) is usually inversely proportional to the overall cardiac output. Cardiac output is determined by saturation difference across a vascular bed, taking into account oxygen consumption and hemoglobin. This is known as the Fick principle. Cardiac output in a healthy heart varies directly with the body’s oxygen consumption and is inversely proportional to hemoglobin. The circulatory system of patients who are anemic usually tries to generate a higher cardiac output to maintain oxygen delivery to the cells of the body.
An increase in saturation across the right side of the heart (anywhere between SVC and pulmonary arteries) represents a left-to-right shunt. If oxygenated blood can mix with venous blood, the saturation rises—the degree of which correlates with the size of the shunt. Conversely, a fall in saturation across the left heart, between the pulmonary veins and the aorta, is abnormal. This represents the addition of deoxygenated blood to oxygenated blood—a right-to-left shunt.
A commonly referenced ratio in pediatric cardiology is the Qp:Qs. In a normal heart, systemic cardiac output (Qs) and pulmonary blood flow (Qp) are equal, or Qp:Qs = 1. If a step-up in saturation is noted across the right heart, suggesting a left-to-right shunt, pulmonary blood flow will exceed systemic blood flow. This can result, in cases of large shunts, in a Qp:Qs of as high as 3:1 or more. This level of shunt is usually poorly tolerated, but small shunts (such as 1.5:1) may be well tolerated for months or years. In cases of right-to-left shunts, Qs will exceed Qp. In these cyanotic patients, the Qp:Qs may be 0.7 or 0.8.
B. Pressures
Pressures should be determined in all chambers and major vessels entered. Systolic pressure in the right ventricle should be equal to the systolic pressure in the pulmonary artery. Likewise, the systolic pressure in the left ventricle should be equal to the systolic pressure of the aorta. The mean pressure in the atria should be nearly equal to (or a point or two lower than) the end-diastolic pressure of the ventricles. If a gradient in pressure exists, an obstruction is present, and the severity of the gradient is one criterion for the necessity of intervention.
For example, a left ventricular systolic pressure in a small child of 140 mm Hg and an aortic systolic pressure of 80 mm Hg, a gradient of 60 mm Hg, would classify as severe aortic valve stenosis. Balloon aortic valvuloplasty would be indicated at the time of the catheterization.
C. Vascular Resistance
In addition to pressure and flow, resistance completes the “concept triad” of congenital heart physiology. Resistance is related to pressure and flow as described in the below equation:
The resistance across a vascular bed can be concretely calculated. The most common clinically relevant example is pulmonary vascular resistance. Patients with congenital heart disease or pulmonary vascular disease may have elevation in pulmonary vascular resistance, which can adversely impact circulation and heart function. To calculate pulmonary vascular resistance, the pressure drop from the pulmonary arteries to the left atrium is divided by pulmonary blood flow (Qp) to obtain a value in units. For example, a patient with a mean pulmonary artery pressure of 15 mm Hg and a left atrial pressure of 9 mm Hg, with a Qp of 3 L/min/m2, has a pulmonary vascular resistance of 2 U/m2.
Normal pulmonary vascular resistance is less than 3 U/m2. Systemic vascular resistance normally covers a wider range, usually from 10 to 30 U/m2. The ratio of pulmonary to systemic vascular resistance is usually less than 0.3. High pulmonary resistance, or a high ratio of resistance, denotes abnormal pulmonary vasculature. It often represents increased risk in patients with congenital heart disease or pulmonary hypertension and results in higher risk of death in severely affected patients.
Cardiac catheterization can be performed to evaluate the effects of pharmaceutical therapy. An example of this use of catheterization is monitoring changes in pulmonary vascular resistance during the administration of nitric oxide or prostacyclin in a child with primary pulmonary hypertension.
Angiography
In the past, angiography was a mainstay of the initial diagnostic methods for congenital heart disease. It is still used for diagnostic purposes in selected cases, but currently is used more frequently to plan interventions or evaluate postsurgical anatomy that is poorly seen by noninvasive methods. Injection of contrast liquid via a well-positioned catheter can illuminate detailed intracardiac and intravascular anatomy more clearly than any other method. Cardiac function can be observed, and anatomic abnormalities may be easily identified. In a growing number of centers, three-dimensional reconstruction of angiograms can provide exquisite delineation of cardiac and vascular structures.
Interventional Cardiac Catheterization
Various procedures are commonly performed in the catheterization laboratory that can improve or cure congenital malformations. Lesions that result in abnormal flow near or within the heart can be occluded, such as a patent ductus arteriosus, atrial septal defect, or ventricular septal defect. Obstruction of heart valves can be addressed through balloon valvuloplasty. Intervention may also be performed on vascular obstruction through angioplasty or stent placement in pulmonary arteries or the aorta. Systemic and pulmonary veins can be modified in a similar fashion, unfortunately with often minimal success in the latter. Devices are now available to allow patients to undergo replacement of failing heart valves without open heart surgery, and an increasing armamentarium of devices are becoming available for treatment of other defects and abnormal vasculature.
With the progression of improved noninvasive imaging, fewer diagnostic cardiac catheterization studies are performed today. The number of interventional procedures, however, is on the rise. Although the risks of cardiac catheterization are very low for elective studies in older children (< 1%), the risk of major complications in distressed or small patients is higher. Interventional procedures, particularly in unstable babies and children, increase these risks further. Increased use of registries is currently being employed to better understand efficacy rates and risks of these procedures, with the hope of optimizing the care of infants and children in the catheterization laboratory.
Backes CH et al: Low weight as an independent risk factor for adverse events during cardiac catheterization of infants. Catheter Cardiovasc Interv 2013 Feb 22 [doi: 10.1002/ccd.24726].
Berman DP et al: The use of three-dimensional rotational angiography to assess the pulmonary circulation following cavo-pulmonary connection in patients with single ventricle. Catheter Cardiovasc Interv 2012 Nov 15;80(6):922–930.
PERINATAL & NEONATAL CIRCULATION
At birth, two events affect the cardiovascular and pulmonary system: (1) the umbilical cord is clamped, removing the placenta from the maternal circulation; and (2) breathing commences. As a result, marked changes in the circulation occur. During fetal life, the placenta offers low resistance to blood flow. In contrast, the pulmonary arterioles are markedly constricted and there is high resistance to blood flow in the lungs. Therefore, the majority of blood entering the right side of the heart travels from the right atrium into the left atrium across the foramen ovale (right-to-left shunt). In addition, most of the blood that makes its way into the right ventricle and then pulmonary arteries will flow from the pulmonary artery into the aorta through the ductus arteriosus (right-to-left shunt). Subsequently, pulmonary blood flow accounts for only 7%–10% of the combined in utero ventricular output. At birth, pulmonary blood flow dramatically increases with the fall in pulmonary vascular resistance and pressure. The causes of prolonged high pulmonary vascular resistance include physical factors (lack of an adequate air-liquid interface or ventilation), low oxygen tension, and vasoactive mediators such as elevated endothelin peptide levels or leukotrienes. Clamping the umbilical cord produces an immediate increase in resistance to flow in the systemic circuit.
As breathing commences, the Po2 of the small pulmonary arterioles increases, resulting in a decrease in pulmonary vascular resistance. Increased oxygen tension, rhythmic lung distention, and production of nitric oxide as well as prostacyclin play major roles in the fall in pulmonary vascular resistance at birth. The pulmonary vascular resistance falls below that of the systemic circuit, resulting in a reversal in direction of blood flow across the ductus arteriosus and marked increase in pulmonary blood flow.
Functional closure of the ductus arteriosus begins shortly after birth. The ductus arteriosus usually remains patent for 1–5 days. During the first hour after birth, a small right-to-left shunt is present (as in the fetus). However, after 1 hour, bidirectional shunting occurs, with the left-to-right direction predominating. In most cases, right-to-left shunting disappears completely by 8 hours. In patients with severe hypoxia (eg, in the syndrome of persistent pulmonary hypertension of the newborn), pulmonary vascular resistance remains high, resulting in a continued right-to-left shunt. Although flow through the ductus arteriosus usually is gone by 5 days of life, the vessel does not close anatomically for 7–14 days.
In fetal life, the foramen ovale serves as a one-way valve shunting blood from the inferior vena cava through the right atrium into the left atrium. At birth, because of the changes in the pulmonary and systemic vascular resistance and the increase in the quantity of blood returning from the pulmonary veins to the left atrium, the left atrial pressure rises above that of the right atrium. This functionally closes the flap of the foramen ovale, preventing flow of blood across the septum. The foramen ovale remains probe patent in 10%–15% of adults.
Persistent pulmonary hypertension is a clinical syndrome of full-term infants. The neonate develops tachypnea, cyanosis, and pulmonary hypertension during the first 8 hours after delivery. These infants have massive right-to-left ductal and/or foramen shunting for 3–7 days because of high pulmonary vascular resistance. Progressive hypoxia and acidosis will cause early death unless the pulmonary resistance can be lowered. Postmortem findings include increased thickness of the pulmonary arteriolar media. Increased alveolar Po2 with hyperventilation, alkalosis, paralysis, surfactant administration, high-frequency ventilation, and cardiac inotropes can usually reverse this process. Inhaled nitric oxide selectively dilates pulmonary vasculature, produces a sustained improvement in oxygenation, and has resulted in improved outcomes.
In the normal newborn, pulmonary vascular resistance and pulmonary arterial pressure continue to fall during the first weeks of life as a result of demuscularization of the pulmonary arterioles. Adult levels of pulmonary resistance and pressure are normally achieved by 4–6 weeks of age. It is at this time typically that signs of pulmonary overcirculation associated with left-to-right shunt lesions (VSD or atrioventricular septal defect [AVSD]) appear.
Konduri GG, Kim UO: Advances in the diagnosis and management of persistent pulmonary hypertension of the newborn. Pediatr Clin North Am 2009;56:579–600, Table of Contents [PMID: 19501693].
Rudolph AM: The fetal circulation and congenital heart disease. Arch Dis Child Fetal Neonatal Ed 2010;95(2):F132–F136 [PMID: 19321508].
HEART FAILURE
Heart failure (HF) is the clinical condition in which the heart fails to meet the circulatory and metabolic needs of the body. The term congestive heart failure is not always accurate, as some patients with significant cardiac dysfunction have symptoms of exercise intolerance and fatigue without evidence of congestion. Right and left heart failure can result from volume or pressure overload of the respective ventricle or an intrinsic abnormality of the ventricular myocardium. Causes of right ventricular volume overload include an ASD, pulmonary insufficiency, or anomalous pulmonary venous return. Left ventricular volume overload occurs with any left-to-right shunting lesion (eg, VSD, PDA), aortic insufficiency, or a systemic arteriovenous malformation. Causes of right ventricular failure as a result of pressure overload include pulmonary hypertension, valvar pulmonary stenosis, or severe branch pulmonary artery stenosis. Left ventricular pressure overload results from left heart obstructive lesions such as aortic stenosis (subvalvar, valvar, or supravalvar) or coarctation of the aorta. Abnormalities of the right ventricular myocardium that can result in right heart failure include Ebstein’s anomaly (atrialization of the right ventricle) and arrhythmogenic right ventricular dysplasia (a genetic disorder where the right ventricular myocardium is replaced by fat). Abnormalities of the left ventricular myocardium are more common and include dilated cardiomyopathy, myocarditis, or hypertrophic cardiomyopathy. As a result of elevated left atrial pressure and impaired relaxation of the left ventricle, left heart failure can lead to right heart failure. Other causes of HF in infants include AV septal defect, coronary artery anomalies, and chronic atrial tachyarrhythmias. Metabolic, mitochondrial, and neuromuscular disorders with associated cardiomyopathy present at various ages depending on the etiology. HF due to acquired conditions such as myocarditis can occur at any age. Children with HF may present with irritability, diaphoresis with feeds, fatigue, exercise intolerance, or evidence of pulmonary congestion (see Table 20–1).
Treatment of Heart Failure
The therapy of HF should be directed toward the underlying cause as well as the symptoms. Regardless of the etiology, neurohormonal activation occurs early when ventricular systolic dysfunction is present. Plasma catecholamine levels (eg, norepinephrine) increase causing tachycardia, diaphoresis, and, by activating the renin-angiotensin system, peripheral vasoconstriction and salt and water retention. There is no gold standard diagnostic or therapeutic approach to HF in children. Treatment must be individualized and therapies should be aimed at improving cardiac performance by targeting the three determinants of cardiac performance: (1) preload, (2) afterload, and (3) contractility.
Inpatient Management of Heart Failure
Patients with cardiac decompensation may require hospitalization for initiation or augmentation of HF therapy. Table 20–12 demonstrates intravenous inotropic agents used to augment cardiac output and their relative effect on heart rate, systemic vascular resistance, and cardiac index. The drug used will depend in part on the cause of the HF.
Table 20–12. Intravenous inotropic agents.
A. Inotropic and Mechanical Support
1. Afterload reduction
A. MILRINONE—This phosphodiesterase-3 inhibitor increases cyclic adenosine monophosphate, thereby improving the inotropic state of the heart. In addition to a dose-dependent increase in cardiac contractility, milrinone is a systemic and pulmonary vasodilator and thus an effective agent in both right and left ventricular systolic dysfunction. Milrinone reduces the incidence of low cardiac output syndrome following open-heart surgery. The usual dosage range is 0.25–0.75 mcg/kg/min.
B. NITROGLYCERIN—Nitroglycerin functions primarily as a dilator of venous capacitance vessels and causes a reduction of right and left atrial pressure. Systemic blood pressure may also fall, and reflex tachycardia may occur. Nitroglycerin is used to improve coronary blood flow and may be especially useful when cardiac output is reduced because of coronary under-perfusion following congenital heart surgery. The usual intravenous dosage range is 1–3 mcg/kg/min.
2. Enhancement of contractility
A. DOPAMINE—This naturally occurring catecholamine increases myocardial contractility primarily via cardiac β-adrenergic activation. Dopamine also directly acts on renal dopamine receptors to improve renal perfusion. The usual dose range for HF is 3–10 mcg/kg/min.
B. DOBUTAMINE—This synthetic catecholamine increases myocardial contractility secondary to cardiospecific β-adrenergic activation and produces little peripheral vasoconstriction. Dobutamine does not usually cause marked tachycardia, which is a distinct advantage. However, the drug does not selectively improve renal perfusion as does dopamine. The usual dose range is essentially the same as for dopamine.
3. Mechanical circulatory support—Mechanical support is indicated in children with severe, refractory myocardial failure secondary to cardiomyopathy, myocarditis, or following cardiac surgery. Mechanical support is used for a limited time while cardiac function improves or as a bridge to cardiac transplantation.
A. EXTRACORPOREAL MEMBRANE OXYGENATION (ECMO)—ECMO is a temporary means of providing oxygenation, carbon dioxide removal, and hemodynamic support to patients with cardiac or pulmonary failure refractory to conventional therapy. The blood removed from the patient via a catheter positioned in the venous system (eg, superior vena cava or right atrium) passes through a membrane oxygenator and then is delivered back to the patient through a catheter in the arterial system (eg, aorta or common carotid artery). Flow rates are adjusted to maintain adequate systemic perfusion, as judged by mean arterial blood pressure, acid-base status, end-organ function, and mixed venous oxygen saturation. The patient is monitored closely for improvement in cardiac contractility. Risks are significant and include severe internal and external bleeding, infection, thrombosis, and pump failure.
B. VENTRICULAR ASSIST DEVICES—Use of ventricular assist devices is increasing in children as device development has progressed. These devices allow for less invasive hemodynamic support than ECMO. A cannula is usually positioned in the apex of the ventricle and removes blood from the ventricle using a battery-operated pump. Blood is then returned to the patient through a separate cannula positioned in the aorta or pulmonary artery, depending on the ventricle being supported. Biventricular support can be done if necessary. Ventricular assist carries lower risk of pump failure than ECMO, but the risk of infection, thrombosis, and bleeding complications remains.
Outpatient Management of Heart Failure
A. Medications
1. Afterload-reducing agents—Oral afterload-reducing agents improve cardiac output by decreasing systemic vascular resistance. Angiotensin-converting enzyme (ACE) inhibitors (captopril, enalapril, and lisinopril) are first-line therapy in children with HF requiring long-term treatment. These agents block angiotensin II–mediated systemic vasoconstriction and are particularly useful in children with structurally normal hearts but reduced LV myocardial function (eg, myocarditis or dilated cardiomyopathies [DCMs]). They are also useful in ameliorating mitral and aortic insufficiency and have a role in controlling refractory HF in patients with large left-to-right shunts in whom systemic vascular resistance is elevated.
2. β-Blockade—Although clearly beneficial in adults with HF, a randomized, controlled study of the use of a β-blocker (carvedilol) in children with HF did not demonstrate any significant improvement compared to placebo. However, β-blockers may still be useful adjunctive therapy in some children with refractory HF already taking ACE inhibitors. In the setting of HF, excessive circulating catecholamines are present due to activation of the sympathetic nervous system. Although beneficial acutely, this compensatory response over time produces myocardial fibrosis, myocyte hypertrophy, and myocyte apoptosis that contribute to the progression of HF. β-Blockers (eg, carvedilol and metoprolol) antagonize this sympathetic activation and may offset these deleterious effects. Side effects of β-blockers are significant and include bradycardia, hypotension, and worsening HF in some patients.
3. Diuretics—Diuretic therapy may be necessary in HF to maintain the euvolemic state and control symptoms related to pulmonary or hepatic congestion.
A. FUROSEMIDE—This rapid-acting loop diuretic may be given intravenously or orally. It removes large amounts of potassium and chloride from the body, producing hypochloremic metabolic alkalosis when used chronically. Electrolytes should be monitored during long-term therapy.
B. THIAZIDES—Thiazides are distal tubular diuretics used to complement furosemide in severe cases of HF.
C. SPIRONOLACTONE—Spironolactone is a potassium-sparing aldosterone inhibitor. It is used frequently in conjunction with furosemide or thiazides for its enhanced diuretic function. Because it spares potassium, supplemental potassium may be avoided. Spironolactone has benefit in adults with HF regardless of its diuretic effect as aldosterone is associated with the development of fibrosis, sodium retention, and vascular dysfunction. This effect has not been proven in children.
4. Digitalis—Digitalis is a cardiac glycoside with a positive inotropic effect on the heart and an associated decrease in systemic vascular resistance. The preparation of digitalis used in clinical practice is digoxin. Large studies in adult patients with HF have not demonstrated decreased mortality of HF with digoxin use, but treatment is associated with reduced hospitalization rates for HF exacerbations. There are no controlled studies in children.
A. DIGITALIS TOXICITY—Any dysrhythmia that occurs during digoxin therapy should be attributed to the drug until proven otherwise. Ventricular bigeminy and first-, second-, or third-degree AV block are characteristic of digoxin toxicity. A trough level should be obtained if digoxin toxicity is suspected.
B. DIGITALIS POISONING—This acute emergency must be treated without delay. Digoxin poisoning most commonly occurs in toddlers who have taken their parents’ or grandparents’ medications. The child’s stomach should be emptied immediately by gastric lavage even if several hours have passed since ingestion. Patients who have ingested massive amounts of digoxin should receive large doses of activated charcoal. In advanced heart block, atropine or temporary ventricular pacing may be needed. Digoxin immune Fab can be used to reverse potentially life-threatening intoxication. Antiarrhythmic agents may be useful.
5. Fluid restriction—Fluid restriction is rarely necessary in children with HF due to the effectiveness of diuretics and the tendency of infants and children with HF to self-regulate intake. Ensuring adequate caloric intake to promote growth is a more important goal in children with HF.
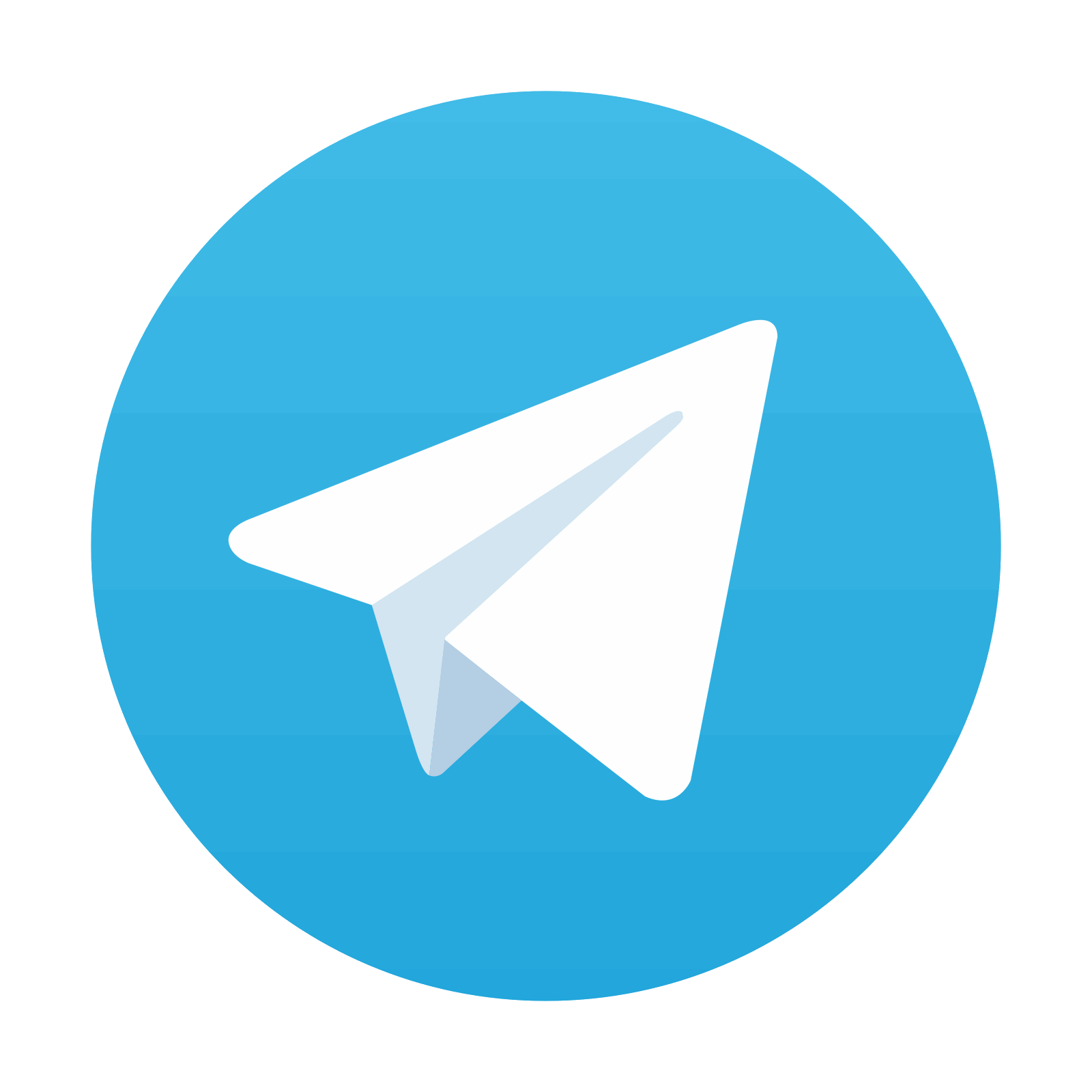
Stay updated, free articles. Join our Telegram channel

Full access? Get Clinical Tree
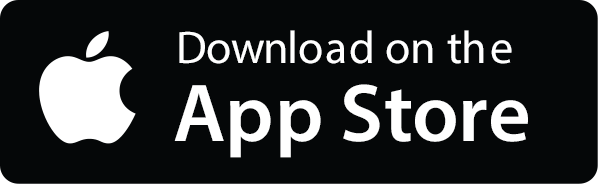
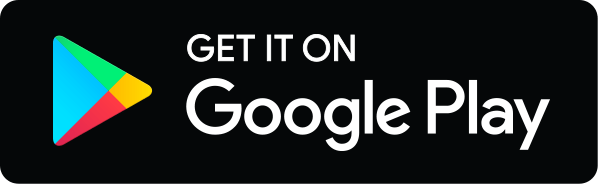