Introduction
The goals of neonatal intensive care include the preservation of cardiorespiratory function and optimal tissue oxygen delivery, prevention of cerebral injury, and ensuring survival with normal neurodevelopment. While respiratory distress and the need for assisted ventilation are common in neonates and often appear pulmonary in origin, clinicians should remember that the heart and lungs are interdependent. Disturbance in one system may rapidly spread to the other, disrupting the balance between tissue oxygen supply and demand, and leading to hypoxia and cell death. Interventions aimed at improving one system may have paradoxically detrimental effects on the other or on the developing brain.
Cardiorespiratory maladaptation in the perinatal period and during postnatal critical illness is dependent on both gestational age at birth and the cascade of subsequent end-organ maldevelopment that follows disturbed preterm or term delivery. Physiologic and technological advances have facilitated the near-routine survival of infants previously considered to be at the limit of viability, though frequently with significant morbidity. However, the optimal approach to hemodynamic monitoring and cardiovascular decision making in critically ill neonates remains inadequately understood. The primary challenge is a lack of knowledge about the influence and pathophysiology of neonatal cardiovascular health on acute and chronic end-organ function. It is further complicated by the dynamic relationship between systemic and pulmonary hemodynamics and cellular metabolism. This chapter provides a pathophysiologic approach to the hemodynamic assessment and management of circulatory disorders of the ventilated neonate, incorporating high-quality evidence and highlighting areas for further research. In addition to the material in this chapter, the management of cardiovascular problems and pulmonary hypertension is also described in Chapter 32 , Chapter 33 .
Cardiovascular Care in the Delivery Room
Transitional Cardiovascular Physiology
At birth, dramatic and rapid changes in cardiovascular function and blood flow occur because of the initiation of lung aeration and removal of the placental circuit. Immediate neonatal hemodynamic and metabolic stability requires three critical adaptations.
Lung aeration triggers an increase in pulmonary blood flow (PBF): Lung aeration results in a rapid decrease in pulmonary vascular resistance (PVR) and increase in PBF to 10 to 20 times resting fetal levels. Pulmonary vasodilation occurs via several mechanisms, including increased oxygenation, enhanced activity of vasodilator agents, and changes in surface tension at the alveolar air–liquid interface that reduce perivascular tissue pressures and facilitate lung recoil.
Central blood flow patterns are significantly altered: At birth, the increases in PBF and arterial oxygen tension, concomitant with clamping of the umbilical cord, result in major alterations in the blood flow across fetal channels. Ductus venosus flow, which is of placental origin via the umbilical vein, decreases markedly after umbilical cord clamping (UCC). Removal of the low-resistance placental circuit results in increased systemic vascular resistance and reduced systemic venous return to the right heart. Simultaneously, increased PBF results in increased pulmonary venous return to the left atrium. Increased left atrial pressure and decreased right atrial pressure displaces the flap of the foramen ovale over the fossa, abolishing the right-to-left atrial flow, although a residual left-to-right atrial shunt may transiently persist.
The postnatal reduction in PVR and increase in systemic vascular resistance (SVR) result in reversal of the fetal right-to-left ductus arteriosus shunt, which becomes balanced by 5 min of age, mostly left to right by 10 to 20 minutes, and entirely left to right by 24 hours of age in healthy term neonates.
Increase in combined ventricular output: Right ventricular output (RVO) increases as the reduction in PVR and abolition of the right-to-left foramen ovale shunt lead to all systemic venous return moving through the right ventricle. The obligatory respiratory work and thermoregulation after delivery result in a tripling in oxygen demand in newborns relative to fetal levels. In addition, the left ventricle, which supplied oxygen to the brain, heart, and upper body during fetal life, must now supply oxygen to the entire body. The result is a near tripling of left ventricular output, which occurs because of increased heart rate and stroke volume. In contrast to the fetal left ventricle, which has a limited capacity to increase output, the left ventricle at birth is able to dramatically increase output because of increased β-adrenergic receptor activity and because it is no longer constrained by a pressure-loaded right ventricle.
Delayed Cord Clamping
The shared fetal–placental circulation is abruptly divided at the time of UCC. Compared with delayed UCC, immediate UCC prior to the onset of ventilation results in a 30% to 50% reduction in cardiac output due to the combined effects of decreased systemic venous return and increased left ventricular afterload. Establishing ventilation prior to UCC maintains cardiac output by preserving systemic venous return and ventricular preload while allowing PBF to increase.
Very preterm infants, in whom low left ventricular output in the first hours of life is associated with increased intraventricular hemorrhage (IVH), may benefit from placental transfusion strategies. Delayed UCC and umbilical cord milking reduce mortality, IVH, hypotension, and the need for blood transfusion in this population, with a limited number of studies supporting its use in extremely low birth-weight infants. The optimal duration of delayed UCC in very preterm infants is unknown, although clinical trials have utilized durations of 30 to 120 seconds.
Approach to the Care of the Neonate with Compromised Oxygenation
Physiology
Impaired oxygenation occurs as a result of hypoventilation, ventilation–perfusion mismatch, impairment in the diffusion of oxygen across the alveolar–capillary membrane, or intra- or extrapulmonary vascular shunts that either reduce pulmonary arterial blood flow or permit mixing of deoxygenated blood into the systemic circulation. The etiologies of compromised systemic oxygenation can be broadly classified as cardiac or pulmonary in origin. Congenital heart lesions include ductal-dependent PBF (e.g., tricuspid atresia) or obligate mixing lesions (e.g., total anomalous pulmonary venous return). Pulmonary disease comprises lesions of both the airways and parenchyma (e.g., pneumonia, pneumothorax, pleural effusion) and the vasculature (pulmonary hypertension).
Pulmonary Hypertension
Pulmonary hypertension (PH) is characterized by a sustained elevation of pulmonary artery pressure (PAP), defined as a mean PAP >25 mm Hg when measured by right-heart catheterization or pulmonary artery peak systolic pressure >35 mm Hg when measured by echocardiography. Mean PAP is influenced by PBF, PVR, and pulmonary capillary wedge pressure (PCWP):
mPAP = [ PVR × PBF ] + PCWP

Persistent Pulmonary Hypertension of the Newborn
Persistent pulmonary hypertension of the newborn (PPHN) is characterized by severe hypoxemic respiratory failure presenting shortly after birth because of inadequate postnatal reduction in PVR. Persistently elevated PVR may be due to neonatal maladaptation (e.g., asphyxia, meconium aspiration syndrome, sepsis), pulmonary maldevelopment (e.g., chronic fetal hypoxia, premature closure of the ductus arteriosus), or pulmonary underdevelopment (e.g., pulmonary hypoplasia, congenital diaphragmatic hernia). The pathophysiology of circulatory derangements in PPHN represents the common final pathway of the effects of excessive right ventricular (RV) afterload (of which PVR is the primary contributor), irrespective of the etiology of PPHN.
Decreased PBF and RV function are the interrelated primary aberrations that culminate in hemodynamic instability ( Fig. 14-2 ). Unlike the thicker-walled and elliptical left ventricle, which is well-equipped to overcome increased afterload, the right ventricle is thin-walled and crescent-shaped, designed to function as a flow generator accommodating the entire systemic venous return to the heart. High PVR results in decreased RV systolic ejection time, stroke volume, and PBF. The right ventricle may adapt by increasing myocardial contractility (known as a homeometric adaptation), but if this adaptation fails, RV dilatation occurs, causing bowing of the interventricular septum into the left ventricle, impairing LV filling, and reducing LV function by disrupting the elliptical configuration that is optimal for LV myocardial performance. The resulting decrease in LV preload, stroke volume, and systemic blood pressure potentiates further negative systolic interaction and right ventricle–arterial uncoupling. Contractile dysfunction of the right ventricle further impairs LV function by virtue of their shared myocardial fibers, a phenomenon termed “ventricular interdependence.” As systemic blood pressure decreases and right atrial pressure (RAP) increases (secondary to elevated RV pressure), right coronary perfusion pressure decreases, resulting in RV ischemia.

PPHN, in its severe form, is called persistent fetal circulation , reflecting a very high PVR state that is associated with elevated RV and RA pressures, very low PBF, and reduced left-heart and aortic volume and pressure. In this setting, pure pulmonary-to-systemic (i.e., “right-to-left”) shunts occur across the foramen ovale and patent ductus arteriosus (PDA), culminating in the systemic delivery of deoxygenated blood. While a right-to-left shunt across the patent foramen ovale (PFO) and PDA may help offload the pulmonary circulation, prevent RV failure, and support systemic blood flow (albeit with deoxygenated blood), it may also potentiate ongoing hypoxemia by perpetuating the low PBF state.
Comorbid neonatal lung disease, such as pneumonia or meconium aspiration syndrome, is negatively synergistic. The reduced PBF exacerbates preexisting V/Q mismatch, hypoxemia, and acidosis, which induces further pulmonary vasoconstriction and may impair the response to pulmonary vasodilator therapies.
Chronic Pulmonary Hypertension
Chronic PH is a clinically and pathophysiologically distinct entity from acute PH, developing later in life and representing a secondary rise in PVR that is driven by either acquired disease or the residual effects of symptomatic congenital pulmonary disease (e.g., congenital diaphragmatic hernia). Chronic lung disease of prematurity is a common complication among extremely preterm infants and is the most common cause of chronic PH in preterm infants.
Clinical Assessment
The diagnosis and evaluation of acute PPHN has been reviewed in previous chapters, and this section will focus on the circulatory assessment and support of infants with PPHN.
Cyanotic Congenital Heart Disease versus PPHN
In infants with suspected PPHN, alternate diagnoses such as cyanotic congenital heart disease (CHD) should be considered and excluded early. Several clinical signs should raise suspicion of CHD in the setting of severe hypoxemia ( Table 14-1 ). An abnormally shaped cardiac silhouette on a chest radiograph, and a markedly abnormal electrical axis of conduction on an electrocardiogram, may be suggestive of CHD (e.g., extreme right-axis deviation in Ebstein’s anomaly). Echocardiography is the only routinely available method of definitive evaluation, and assessment is mandatory when infants with presumed PPHN fail to demonstrate improvement in hypoxemia as would be expected with resuscitative measures and pulmonary vasodilator therapies, or if extracorporeal membrane oxygenation is being considered. Delayed diagnosis of cyanotic CHD presenting with cardiovascular collapse and end-organ dysfunction is associated with increased mortality.
Clinical Sign | Significance and Physiology |
---|---|
Reverse differential cyanosis (preductal oxygen saturation is >10% lower than postductal) | Indicates right-to-left shunt of oxygenated blood across PDA (e.g., TGA, TAPVD) |
Murmur | Critical valvular obstruction or coarctation |
Oxygen saturation that is minimally variable with increases/decreases in FiO 2 | Indicates reduced or relatively fixed PBF and the absence of a labile pulmonary vascular bed |
Onset of severe hypoxemia after the first 12 h of life | Ductal-dependent PBF lesions presenting at time of closure of the ductus arteriosus |
Diminished amplitude of lower limb pulses OR blood pressure in the lower limbs (postductal) >10-20 mm Hg lower than in the right arm (preductal) | Critical coarctation of the aorta |
The hyperoxia test may be helpful to distinguish between PPHN and cyanotic CHD in neonates with hypoxemic respiratory failure. A rise in PaO 2 >80 to 120 mm Hg above baseline after 100% oxygen administration, or an absolute PaO 2 >150 mm Hg in 100% oxygen, suggests that cyanotic CHD is unlikely. PaO 2 <50 mm Hg in 100% oxygen is highly suggestive of cyanotic CHD and should prompt the initiation of intravenous prostaglandin E 2 and referral to a pediatric cardiology center. PaO 2 50 to 150 mm Hg in 100% oxygen is equivocal and necessitates further evaluation.
Management
PPHN is a common, challenging clinical scenario that requires management in a tertiary-level neonatal intensive care unit (NICU). The management of PPHN comprises resuscitative measures and general management strategies aimed at optimizing cardiopulmonary status and targeted, judicious use of pulmonary vasodilators and vasoactive medications to optimize PBF and systemic oxygen delivery.
Resuscitation and General Management
In infants with mild hypoxic respiratory failure, stabilization and amelioration may frequently be achieved by the timely initiation of optimal intensive care support, without the need for inotropes or pulmonary vasodilator therapies. Some infants with mild PPHN secondary to hypoxic–ischemic encephalopathy, respiratory distress syndrome, or sepsis may achieve stability with noninvasive ventilation. However, infants with more significant disease typically require endotracheal intubation and invasive mechanical ventilation, which permits the administration of higher peak and mean airway pressures to facilitate alveolar recruitment and ventilation.
Several physiologic and therapeutic targets for ventilated infants with PPHN may lower PVR and should be employed when the etiology of PPHN is suspected or proven to be due to elevated PVR and unrelated to increased PCWP or PBF. Mean airway pressure should be titrated to achieve alveolar recruitment and maintain lung volumes at functional residual capacity, which is the point of maximum pulmonary compliance and minimal PVR ( Fig. 14-3 ). Acidemia should be corrected, initially by targeting normal (but not low) PaCO 2 and by optimizing the systemic delivery of oxygen to minimize ischemia and associated lactic acidosis, with the goal of achieving a normal serum pH. In neonates with adequate assisted ventilation (defined as a near-normal PaCO 2 ) the administration of a slow intravenous infusion of sodium bicarbonate may augment serum pH and reduce PVR, though the clinical benefits of exogenous alkali administration remain unproven. Oxygen supplementation should be titrated to target PaO 2 of 60 to 80 mm Hg, which minimizes PVR and avoids the deleterious effects of hyperoxia. Agitation and work of breathing precipitate an acute rise in PVR and therefore adequate sedation/analgesia should be administered, with neuromuscular blockade for severely affected infants.

Specific Pulmonary Vasodilator Therapies and the Role of Targeted Neonatal Echocardiography
Infants with severe hypoxemic respiratory failure (HRF) may have insufficient improvement after the initiation of invasive mechanical ventilation, and the oxygen index (OI) should be calculated to quantify the derangement in oxygenation.
Oxygen Index = Mean Airway Pressure ∗ FiO 2 ∗ 100 Pa O 2
Infants with HRF and OI>25 may benefit from inhaled nitric oxide (iNO), which has been demonstrated to reduce the composite outcome of death or extracorporeal membrane oxygenation (ECMO). The earlier initiation of iNO for OI 15 to 25 improves oxygenation but without improvement in mortality or the need for ECMO. In units in which access to iNO and high-frequency ventilation is limited, oral sildenafil improves oxygenation and reduces mortality in term infants with HRF.
For preterm infants with HRF, the potential benefits of routine iNO are less clear and a targeted approach to administration is required. A recent meta-analysis of individual patient data from international trials found that iNO conferred no improvement in mortality, bronchopulmonary dysplasia (BPD), or severe neurologic events compared with placebo. The lack of benefit of iNO in preterm infants may reflect the heterogeneity of clinical disease in the study population, in which pulmonary parenchymal disease and less reversible etiologies of increased PVR are more common. Nitric oxide may be beneficial in highly selected preterm infants with refractory HRF and echocardiography-proven PH. Observational studies have associated iNO with improvement in oxygenation and neonatal outcomes in preterm infants with pulmonary hypoplasia from prolonged exposure to oligohydramnios after second-trimester premature rupture of the fetal membranes.
Echocardiography should be performed in any infant with severe HRF with a suboptimal response to iNO, both to exclude CHD and to characterize the hemodynamic derangement ( Tables 14-2 and 14-3 ). First, the severity of PH should be assessed, using quantitative and qualitative measures and assessment of the ductal and atrial shunts. Second, the effects of elevated PVR on ventricular (RV and LV) myocardial performance, outputs, and dilatation should be evaluated. Targeted neonatal echocardiography (TNE) may help guide the administration of second-line pulmonary vasodilators whose use is primarily supported by physiologic principles.
Pulmonary Artery Resistance and Pressure | Left-Heart Function and Systemic Circulation | Right-Heart Function and Pulmonary Blood Flow |
---|---|---|
Quantitative: Estimation of RVSp from peak velocity of TR jet or PDA Doppler Semiquantitative: Monitor progress using serial measurements of Doppler-derived time intervals characteristic of systolic blood flow in MPA—pulmonary artery ejection time to pulmonary artery acceleration time. Qualitative: Estimation of RVSp relative to systemic systolic pressure—interventricular septal curvature and pattern of blood flow across PDA and PFO | LV preload: S ubjective assessment of LV chamber size and Doppler of pulmonary venous inflow and mitral valve inflow in diastole LV contractility: Quantitative assessment
Systemic blood flow: Quantitative measurement of LVO and stroke distance in the ascending aorta | RV contractility: Quantitative assessment
Pulmonary blood flow: Quantitative measurement of RVO and stroke distance in the MPA |
Vasopressin may improve oxygenation in infants with refractory severe HRF and hypotension despite iNO therapy, especially in infants with decreased PBF as evidenced by exclusively right-to-left shunts across the foramen ovale and ductus arteriosus. The mechanism of action of vasopressin has not been fully elucidated, but may involve a reduction in PVR/SVR ratio and improved LV filling, resulting in improved PBF, myocardial performance, and LV output (LVO). Vasopressin administration should be avoided in infants with cardiogenic shock due to primary LV dysfunction, as its potent systemic vasoconstrictive effects (and increased LV afterload) may result in reduced LVO. Although vasopressin administration results in increased renal blood flow, its use may be associated with hyponatremia due to free water retention, an effect that resolves upon discontinuation.
Intravenous low-dose epinephrine is an alternative to vasopressin and provides inotropic support for infants with myocardial dysfunction; however, its effect on the PVR/SVR ratio is unpredictable and it has been associated with lactic acidosis.
Infants with severe RV dysfunction and dilatation whose ductus arteriosus is closed or restricted may benefit from an intravenous infusion of prostaglandin E 2 (PGE 2 ) to restore ductal patency and reduce RV afterload. However, PGE 2 should be used cautiously in the setting of diastolic hypotension because of its systemic vasodilatory effects, which may decrease systemic venous return, exacerbate coronary hypoperfusion, and precipitate acute circulatory collapse.
Intravenous milrinone, a phosphodiesterase-3 inhibitor with lusitropic, inotropic, and systemic vasodilatory effects, has been associated, in case series and nonrandomized studies, with improved oxygenation in term and preterm infants with PH and RV dysfunction. Use of milrinone should be avoided in infants with diastolic hypotension and with hypoxic–ischemic encephalopathy undergoing therapeutic hypothermia, in whom reduced milrinone metabolism may result in profound hypotension.
Approach to the Infants with Compromised Systemic Hemodynamics
Physiology
A low systemic blood flow (SBF) state is defined as having inadequate ventricular output to meet tissue oxygen consumption. Delivery of oxygen (DO 2 ) also comprises measures of oxyhemoglobin saturation and the arterial tension of oxygen; however, cardiac output is the most important contributor to DO 2 in neonates in the absence of profound anemia or severe hypoxemia.
DO 2 = Cardiac output × Arterial O 2 concentration [ SV × HR ] [ 1 . 34 × Hb × Sa O 2 ] + [ Pa O 2 × 0 . 003 ]
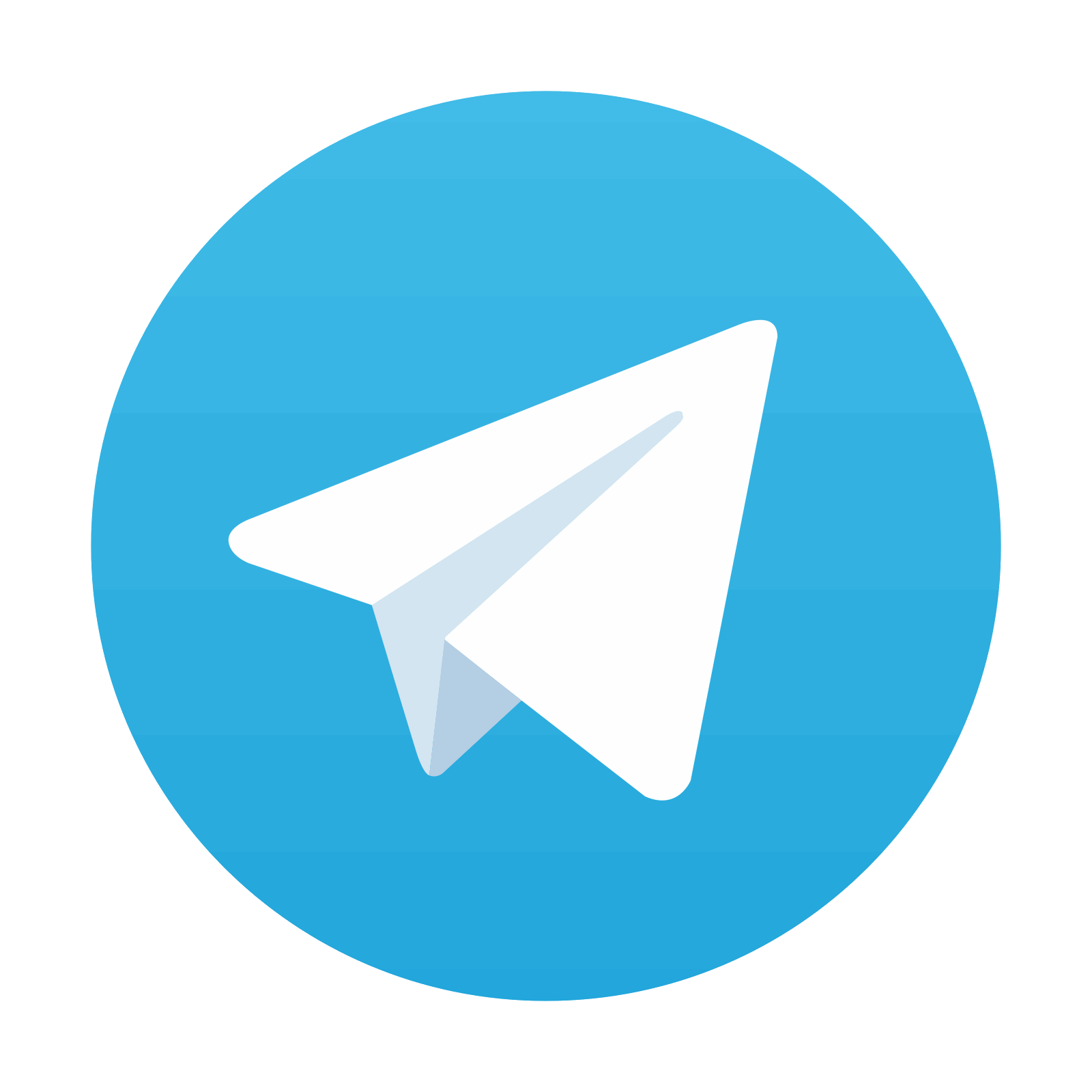
Stay updated, free articles. Join our Telegram channel

Full access? Get Clinical Tree
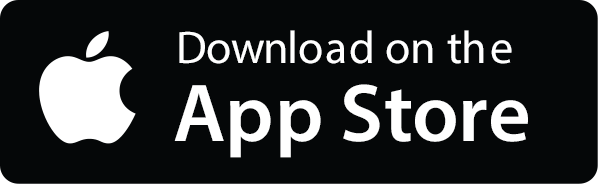
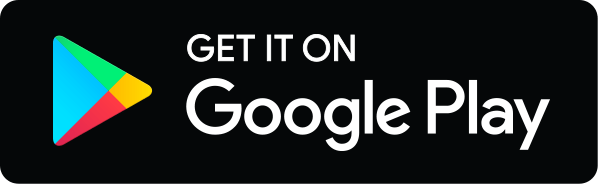