Robert M R Tulloh, Jessica Green • Know the anatomy and embryology of the normal heart • Know the anatomy of the commoner types of congenital heart disease • Know the genetic and environmental factors in the aetiology of heart disease • Understand the physiological basis of myocardial function • Understand the pathophysiology of cardiac conditions, including heart failure • Understand how the electrical activity of the heart translates to the ECG • Know the possible cardiac complications of other system disorders
Cardiology
Congenital heart disease is the most common group of structural malformations in children (8 per 1000 live-born infants) and knowledge of the anatomy and physiology of the cardiovascular system is necessary to understand the clinical manifestations, natural history and treatment options of these disorders.
The anatomy of the normal heart
Although conventionally described in terms of ‘right-’ and ‘left-sided’ chambers, in reality the heart does not hang down from the great vessels with the ventricles directly below the atria. Instead, it is tilted forwards and to the left so that the ‘right’ chambers have a more anterior position than the left (Fig. 18.1). This is further complicated by the twisted nature of the ventricular outflow tracts; the aorta, despite emerging from the left ventricle, has its valve in a right-sided and inferior position in relation to the pulmonary valve. In congenitally malformed hearts, when the chambers do not occupy their usual positions, the positional distinction of right and left becomes confusing and is avoided by referring instead to the ‘morphological’ right and left.
The morphological approach to examination of the heart enables easier description of congenital cardiac malformations. The basic mantra is that all hearts are built from three segments – the atria, ventricular masses and arterial trunks with the atrioventricular junction and the ventriculoarterial junction interposing between adjoining segments. A normal heart would therefore be described as situs solitus (i.e. the atria are in the correct orientation), concordant atrioventricular connection and concordant ventriculoarterial connection. Congenital abnormalities can be present in one or more of these segments.
The atria
The right atrium is slightly larger than the left atrium but its walls are thinner. The internal wall of the right atrium is composed of a smooth, posterior portion (into which the vena cava and coronary sinus drain) and a ridge-like, muscular anterior portion with characteristic pectinate muscles distributed down into the vestibule of the tricuspid valve. The left atrium receives the four pulmonary veins into its smooth-walled posterior surface. The atria share a common wall – the atrial septum. This contains a flap valve of the fossa ovalis, which overlaps the atrial walls, so even if not fused, there will be no shunting across the septum, as long as the left atrial pressure exceeds that of the right atrium. The vestibule of the left atrium supports the leaflets of the mitral valve (two cusps) and is smooth.
The ventricles
The ventricles consist of inlet, apical trabecular and outlet components. The shape of the normal right ventricle is determined by the inlet being inferior and to the right of the outlet. The inlet surrounds and supports the leaflets and tension apparatus of the tricuspid valve. The right ventricle also has characteristic coarser trabeculations than those on the left. The pulmonary semilunar valve (three cusps) is supported by a muscular infundibulum. The inlet component of the left ventricle contains and surrounds the mitral valve, which characteristically has no cordal attachments to the ventricular septum. The apical part of the ventricle has fine criss-crossing trabeculations and a smooth septal surface. The three leaflets of the aortic valve are attached in a semilunar fashion but, unlike the pulmonary valve, the hinge lines attach in part to fibrous structures and in part to the muscular walls of the ventricle.
The great vessels
The aorta arises from the middle of the base of the heart and curves upwards to the aortic arch, where it gives rise to the brachiocephalic, the left common carotid and the left subclavian vessels. The three sinuses of Valsalva support the leaflets of the aortic valve and two of these sinuses give rise to the right and left coronary arteries. The pulmonary trunk runs from the pulmonary infundibulum, where its sinuses support the leaflets of the pulmonary valve, to its bifurcation into the right and left pulmonary arteries. In the fetal circulation, the ductus arteriosus extends from the pulmonary trunk into the descending aorta and demarcates the isthmus of the aorta.
Peripheral blood vessels
The vessels of the peripheral circulation are made up of three layers (Fig. 18.2):
• Tunica intima – a single layer of flattened endothelial cells providing a smooth lining.
• Tunica adventitia – outermost, fibrous layer composed of connective tissue.
The pericardium
The heart and roots of the great vessels are encased in the pericardial sac, which consists of fibrous and serous parts. The outer fibrous part fuses underneath the heart with the central tendon of the diaphragm and also fuses with the ends of the great vessels as they enter or leave, so helping to anchor the heart in its central position. The inner, serous pericardium has two layers: the parietal layer that adheres to the inner surface of the fibrous pericardium; over the great vessels it becomes reflected onto the outer surface of the heart as the visceral layer or epicardium. The adjacent surfaces of the serous pericardium are kept lubricated by pericardial fluid, allowing the surfaces to glide over one another as the heart beats.
Embryology and its relevance to congenital heart disease
The heart is the first organ that functions within an embryo, by the 4th week of gestation, when the nutritional and oxygen requirements of the growing embryo can no longer be met solely by diffusion from the placenta. The embryo is made up of three germ layers: the ectoderm, mesoderm and endoderm. The heart develops predominantly from the mesoderm and is remodelled throughout its development. Its early development is summarized in Figure 18.3. By day 22, coordinated contractions of the heart tube are present. Following fusion, the primitive heart tube develops a series of expansions separated by shallow sulci, forming the heart’s various divisions: the sinus venosus, atrium (future atria), bulbus cordis (future right ventricle), ventricle (future left ventricle) and truncus arteriosus.
Early embryology of the heart
During weeks 4 and 5, the heart forms an ‘S’ shape, demonstrating left to right asymmetry. Looping brings the future left ventricle forward in continuity with the sinus venosus (future left and right atria), whereas the future right ventricle is shifted rightward and in continuity with the truncus arteriosus (future aorta and pulmonary artery). In weeks 5 and 6, the neural crest mesenchymal cells proliferate and migrate through the primordial pharynx and over the aortic arch arteries to reach the outflow tract, the aorticopulmonary septum forms and cleavage of aortic and pulmonary trunk occurs. The primordial pulmonary vein is incorporated into the left atrium from week 5. The vein develops as an outgrowth of the dorsal atrial wall and as the atrium expands, the primordial pulmonary vein and its main branches are gradually incorporated into the vein of the left atrium, forming the four pulmonary veins.
In total anomalous pulmonary venous connections, none of the pulmonary veins connects with the left atrium. They open into the right atrium or into one of the systemic veins or into both. In partial anomalous pulmonary venous connections, one or more pulmonary veins have similar anomalous connections; the others have normal connections.
In weeks 6 and 7, the atrioventricular valves form and a ridge of tissue, the crista terminalis, forms superior to the right valve forming the conduction pathway from the newly identifiable sinu-atrial node to the atrioventricular node. Division of the primordial ventricle starts with formation of a median muscular ridge in the floor of the ventricle near its apex. The median walls of the enlarging ventricles approach one another and fuse to form the primordium of the muscular part of the intraventricular septum.
Later, there is active proliferation of myoblasts in the septum, which increase in size. Until week 7, there is a crescent-shaped interventricular foramen between the free edge of the intraventricular septum and the fused endocardial cushions, which permits communication between the right and left ventricles. It usually closes by the end of the seventh week, as the bulbar ridges fuse with the endocardial cushion. The membranous part of the intraventricular septum is derived from an extension of tissue from the right side of the endocardial cushion to the muscular part of the intraventricular septum. This tissue merges with the aorticopulmonary septum and the thick muscular part of the intraventricular septum. After closure to the interventricular foramen and the formation of the membranous part of the intraventricular septum, the pulmonary trunk is in communication with the right ventricle and the aorta is in communication with the left ventricle.
In weeks 7 and 8, the truncus arteriosus (TA) is divided in two by a spiral process of central septation, forming the truncoconal septum, which results in the formation of the aorta and pulmonary trunk. This septum also grows into the ventricles, where it forms the membranous ventricular septum. Swellings develop at the inferior end of the truncus arteriosus, and these give rise to the semilunar valves.
Truncus arteriosus results from the failure of the truncal ridges and the aorticopulmonary septum to develop normally and divide the truncus arteriosus into the aorta and pulmonary trunk. In this defect, a single arterial trunk, the TA, arises from the heart and supplies the systemic, pulmonary and coronary circulations. A VSD is always present with the TA anomaly and the TA overrides the VSD.
Development of the venous system
At week 4, three systems of paired veins drain into the primordial heart:
• Cardinal veins – carry poorly oxygenated blood from the body of the embryo, becomes the caval system
• Umbilical system – carries well-oxygenated blood from the placenta, involutes after birth
The umbilical veins run on each side of the liver and carry well-oxygenated blood from the placenta to the sinus venosus. As the liver develops, the umbilical veins lose their connection with the heart and empty into the liver. The right umbilical vein disappears during the 7th week, leaving the left umbilical vein as the only vessel carrying well-oxygenated blood from the placenta to the embryo. A large venous shunt – the ductus venosus – develops within the liver and connects the umbilical vein with the inferior vena cava and so forms a bypass through the liver, enabling most of the blood from the placenta to pass directly to the heart without passing through the capillary networks of the liver.
The inferior vena cava (IVC) forms from a series of changes in the primordial veins of the trunk that occur as blood returning from the caudal part of the embryo is shifted from the left to the right side of the body. The IVC is composed of four main segments, hepatic, pre-renal, renal and post-renal, each derived from different venous structures. Because of the many transformations that occur during the formation of the IVC and superior vena cava (SVC), variations in their adult form occur, the most common variant is a persistent left SVC, which drains into the right atrium through the enlarged coronary sinus. Less commonly, the IVC may have its abdominal course interrupted, so blood draining from the lower body returns to the heart through the azygos system.
Development of the arterial system
As the pharyngeal arches form during the 4th and 5th weeks, they are penetrated by arteries (aortic arches), which arise from the aortic sac and terminate in the dorsal aortae. Initially, the paired dorsal aortae run through the entire length of the embryo but they soon fuse to form a single dorsal aorta, just caudal to the pharyngeal arches. During weeks 6 to 8, they are transformed into the adult arterial arrangement of carotid, subclavian and pulmonary arteries.
Initially, there are approximately thirty branches of the dorsal aorta. However, with increasing gestation, most of these branches disappear whilst some fuse together and persist. In the neck they join to form the vertebral arteries, in the thorax they form the intercostal arteries, the lumbar arteries in the abdomen, the common iliac arteries and the sacral arteries in the sacrum. The unpaired ventral branches of the dorsal aorta supply the yolk sac, allantois and chorion. The vitelline arteries pass to the yolk sac and later the primordial gut, which forms from the incorporated part of the yolk sac. Three vitelline arteries remain: the coeliac trunk supplying the foregut, superior mesenteric artery to the midgut and the inferior mesenteric artery to the hindgut.
The paired umbilical arteries pass through the connecting stalk (later umbilical cord) and become continuous with vessels in the chorion. The umbilical arteries carry poorly oxygenated blood to the placenta. Proximal parts of the umbilical arteries become the internal iliac arteries, and superior vesical arteries whereas distal parts obliterate after birth and become the medial umbilical ligaments.
Because of the many changes involved in transformation of the embryonic pharyngeal arch system into the adult arterial pattern, there are a number of irregularities that may result from the persistence of parts of aortic arches that usually disappear (e.g. right arch of the aorta, double aortic arch), or from disappearance of parts that normally persist (e.g. anomalous right subclavian artery).
Fetal circulation and transitional changes after birth
These are described in Chapter 10, Perinatal medicine.
Persistent ductus arteriosus (PDA)
This is the second commonest form of congenital heart disease, accounting for approximately 10% in term infants. Failure of involution of the ductus arteriosus by failure of contraction of its muscular wall after birth is the primary cause of patency. It may occur as an isolated anomaly or in association with cardiac defects. Large differences between aortic and pulmonary blood pressures can result in increased blood flow through the ductus arteriosus preventing normal constriction. Such pressure differences may be seen in coarctation of the aorta, transposition of the great arteries or pulmonary stenosis and atresia.
Genetic and environmental factors in the aetiology of heart disease
Only a small percentage of congenital heart disease cases have identifiable causes:
• Primary genetic factors (e.g. chromosomal abnormalities, single gene abnormalities): 10%
• Environmental factors (e.g. chemicals, drugs, viruses, maternal disease (Table 18.1)): 3–5%
Table 18.1
Prenatal maternal factors associated with cardiac disease in the neonate
Prenatal factor | Associated defect |
Diabetes mellitus | LVOT obstruction, hypertrophic cardiomyopathy, TGA, VSD |
Maternal phenylketonuria | VSD, ASD, PDA, coarctation of the aorta |
SLE | Heart block, pericarditis, endomyocardial fibrosis |
Rubella | PDA, branch pulmonary stenosis |
Alcohol | Pulmonary stenosis, VSD, ASD, tetralogy of Fallot |
Aspirin | PPHN |
Lithium | Ebstein anomaly |
Coxsackie B infection | Myocarditis |
Diphenylhydantoin | Aortic stenosis, pulmonary stenosis |
• Genetic–environmental interactions (i.e. multi-factorial): 85%
The recurrence risk of cardiovascular anomalies varies from 1–4% and is higher with the commoner lesions, e.g. the recurrence risk for VSD is 3% whereas Ebstein anomaly is 1%. With two affected first-degree relatives, the risk is tripled. Table 18.2 lists the syndromes associated with cardiac lesions.
Table 18.2
Syndromes associated with cardiac lesions
Syndrome | Lesion |
Trisomy 21/ Down’s syndrome | Up to 50% will have congenital heart disease. VSD commonest followed by AVSD. Pulmonary vascular disease develops early. |
Turner’s syndrome XO | Bicuspid aortic valve – 33% Coarctation of the aorta |
Noonan’s syndrome (PTPN11 gene on chromosome 12) | Pulmonary stenosis, ASD, hypertrophic cardiomyopathy |
Williams syndrome (deletion of genes from the long arm of chromosome 7) | Supravalvular aortic stenosis, branch pulmonary artery stenosis |
DiGeorge syndrome (22q11.2 deletion) | Aortic arch abnormalities, truncus arteriosus, pulmonary atresia, tetralogy of Fallot, familial VSD |
CHARGE (CHD7 gene on chromosome 8) | Tetralogy of Fallot, VSD, AVSD, double outlet right ventricle |
VACTERL (abnormalities in structures derived from embryonic mesoderm; no known genetic or chromosomal cause) | Tetralogy of Fallot, VSD, coarctation of the aorta, PDA |
Trisomy 18 – Edwards syndrome | VSD, ASD, PDA, coarctation of the aorta, bicuspid aortic valve, double outlet right ventricle |
Trisomy 13 – Patau syndrome | VSD, ASD, PDA, coarctation of the aorta, bicuspid aortic valve, double outlet right ventricle |
Marfan’s syndrome (FBN1 gene encoding connective protein fibrillin-1 on chromosome 15) | Aortic aneurysm, aortic regurgitation, mitral regurgitation |
Ehlers–Danlos syndrome (defect in synthesis of type I or III collagen) | Aortic root dilation |
Tuberous sclerosis (TSC1 on chromosome 9 or TSC2 on chromosome 16; code for tumour suppressor genes hamartin and tuberin, respectively; autosomal dominant) | Cardiac rhabdomyoma |
Cardiovascular physiology
The heart is an electrically controlled and chemically driven mechanical pump. In order for the heart to function as a pump, its chambers must be filled with sufficient amounts of blood before each contraction. The heart fills passively but due to its limited pressure–volume compliance, it offers its own impediment to filling. By filling passively, the heart pumps out blood at a rate determined by the rate of blood coming to it. The venous return is the amount of blood flowing into the right atrium per minute from the systemic circulation. It is driven by:
The volume ejected by each ventricle (about 70 mL in the adult) is the stroke volume; with a heart rate of over 70 beats per minute over 5 litres of blood are pumped out of each ventricle every minute – this is the cardiac output. The cardiac output refers to the volume pumped out by either the right or left ventricle, not the total amount pumped by both. The cardiac output will vary with changes in stroke volume or heart rate and is governed by the central equation:

The heart is able to modify its cardiac output by adjusting the number of contractions per unit of time and by moderating the volume of blood pumped by each contraction (stroke volume). These mechanisms are brought about through a number of intrinsic biophysical pathways as well as extrinsic control.
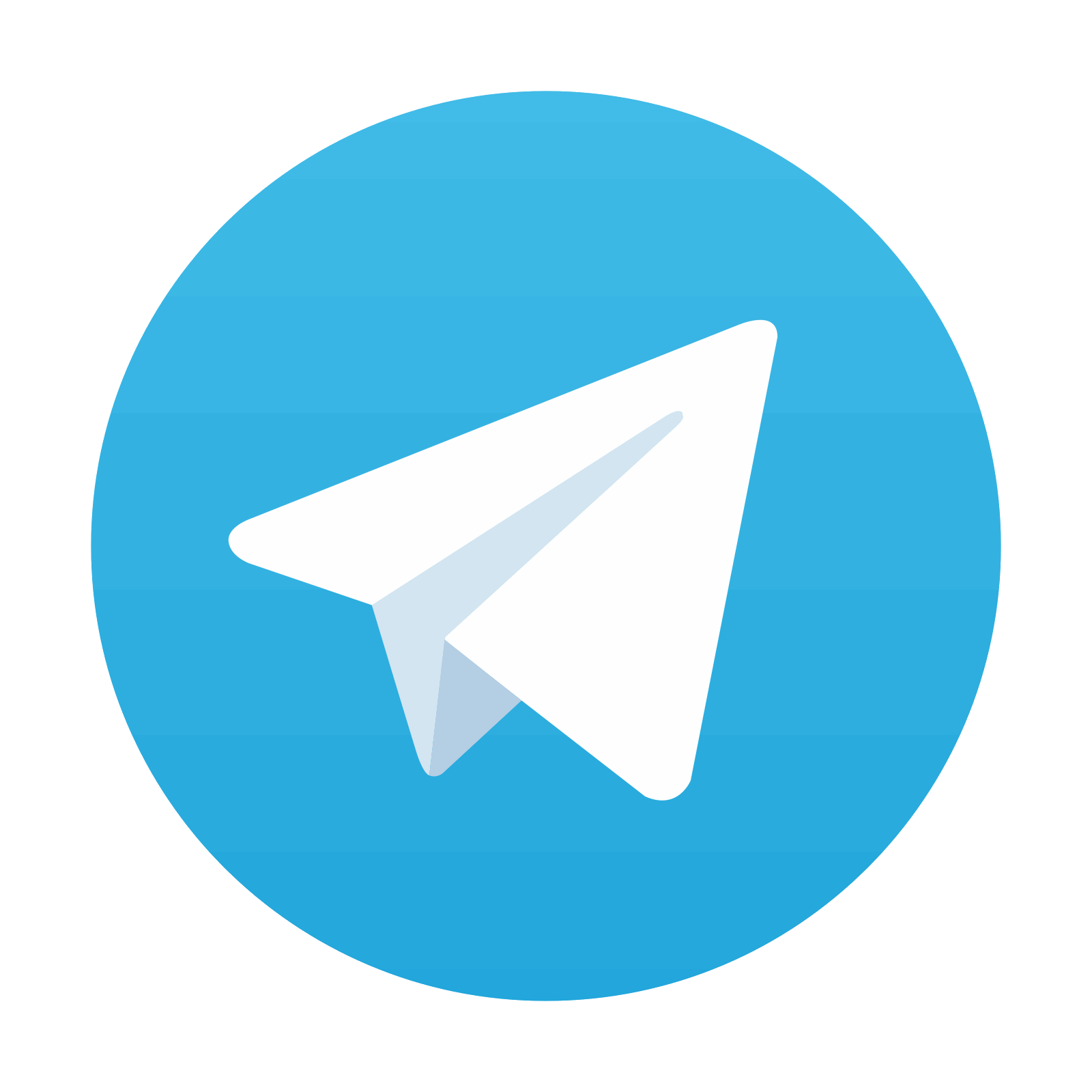
Stay updated, free articles. Join our Telegram channel

Full access? Get Clinical Tree
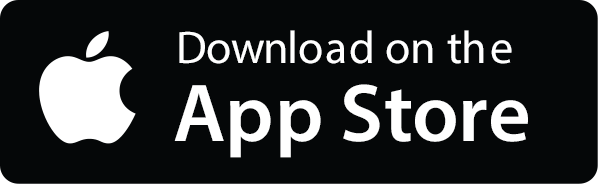
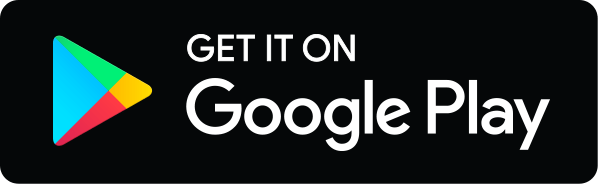