KEY POINTS
- 1.
Congenital heart diseases (CHDs) are an important cause of morbidity and mortality in young infants. These malformations account for approximately 300,000 deaths yearly.
- 2.
Cardiac septal defects are the most common type of anomalies. Ventricular septal defects (VSDs) are seen most frequently, in 5 to 50 per 1000 live births. Atrial septal defects (ASDs) also common and account for 8% to 10% of all congenital heart defects.
- 3.
Atrioventricular canal (AVC) defects are commonly associated with Trisomy 21 and heterotaxy, although patients with normal chromosomes can also show AVC defects.
- 4.
Tetralogy of Fallot is the most frequently seen cyanotic heart defect; it is characterized by pulmonic valve stenosis, VSD, overriding aorta, and right ventricular hypertrophy. Transposition of the great arteries is another important cyanotic defect.
- 5.
Aortic stenosis and interrupted arch are important anomalies in the left outflow tract. With improved cardiac imaging, many infants with hypoplastic left heart syndrome are now being recognized prenatally and being treated with timely, appropriate intensive care services.
- 6.
Further work is needed to determine the predisposing molecular defects that increase the risk of congenital cardiac defects. There is room for improvements in cardiovascular support and management. However, some of most complex defects have limited therapeutic options and need cardiac transplantation.
Congenital heart diseases (CHDs) are an important cause of morbidity and mortality in the neonatal period and beyond. Despite major advances in the medical and surgical treatment, CHDs pose a significant global health and economic burden and account for approximately 300,000 deaths yearly. In this chapter, we have focused on the prevalence, anatomic details, and pathophysiology of the most frequently seen congenital cardiac diseases (defects).
Septal Defects
Atrial Septal Defects
Prevalence
Atrial septal defects (ASDs) account for 8% to 10% of all congenital heart defects and are about twice as common in females as in males. , With the advent of echocardiography and increasing access to healthcare, the prevalence has increased over time, especially with increasing age of the patient population.
Embryology and Anatomy
The septation of the atrium begins during the fourth week of life when the septum primum grows inferiorly in the direction of the endocardial cushion. The failure of septum primum attachment and fusion with the endocardial cushion leaves an opening called the ostium primum. Additionally, in the mid to superior portion of the septum primum, tissue reabsorption occurs, creating an opening called the ostium secundum opening. These result in two defects in the septum primum: (1) the ostium primum, located inferiorly in the septum just above the endocardial cushion, and (2) the ostium secundum, located superiorly and in the center of the septum. , To the right of the septum primum, due to an anterosuperior folding of the atrium, a second septum grows inferiorly, named the septum secundum. , This septum consists of a superior and inferior limb. The inferior limb of the septum secundum grows inferiorly and completes the septation of the lower part of the septum by fusing with the endocardial cushion closing the ostium primum. The superior limb of the septum secundum has a more muscular ridge and covers the ostium secundum incompletely. This incomplete covering of the foramen secundum and adhesion by the septum secundum results in the fetal communication between the right and left atrium called the foramen ovale. , The superior ridge of the foramen ovale is called the limbus of fossa ovalis and is the inferior ridge of the superior septum secundum.
Secundum ASD is the most common form of ASD, accounting for 75% of all ASDs, followed by primum ASD, accounting for 20% of all ASDs. Less common types of ASD are sinus venosus and coronary sinus ASDs. Fig. 37.1 shows schematic figures of various types of ASDs.

Although most ASDs occur sporadically, a mother with an ASD has an 8% to 10% chance of having a child with ASD. Inherited mutations in genes encoding cardiac transcription factors and sarcomeric proteins have been reported as an underlying cause for familial recurrence of nonsyndromic CHD in humans, in particular cardiac septal defects. Holt-Oram syndrome, an autosomal dominant syndrome that is a result of a TBX5 mutation, is the most common syndrome associated with an ASD. Holt-Oram syndrome is characterized by varying degrees of thumb hypoplasia, absent metacarpals, and radius. Heterozygous mutation in the NKX2.5/CSX transcription factor is associated with familial cases of ASD. , This NKX2.5 mutation is also associated with first-degree heart block, which may progress to complete heart block. Therefore all babies with ASD should have a baseline electrocardiogram to evaluate for heart block. A dominantly inherited form of ASD is found with a missense mutation in an alpha-myosin heavy chain locus on chromosome 14q12. This myosin heavy chain protein is a structural protein found in high concentrations during atrial septation.
Patent Foramen Ovale
The patent foramen ovale (PFO) is a normal anatomic communication in the atrial septum that allows oxygenated blood to flow from the placenta via the ductus venosus through the PFO to the left atrium (LA) during fetal life. The septum primum and the septum secundum are developed normally. The foramen ovale is anatomically located in the fossa ovalis. Immediately after birth, with an increase in pulmonary venous return to the LA and lowering of the pulmonary vascular resistance (PVR), the pressure in the LA exceeds that of the right atrium (RA), resulting in the septum primum being pushed toward the septum secundum and closing the PFO like a flap, leading to functional closure of the PFO. Over time the septum primum adheres to the superior limbus of the septum secundum, causing permanent anatomic closure of the PFO.
Secundum ASD
A secundum defect is a defect of the septum primum within the fossa ovalis, with a normally developed septum secundum. The defect size may vary from a few millimeters to 2 to 3 cm, with significant defects resulting from an almost complete absence of the septum primum.
In infants, secundum defects less than 5 mm in size have an 80% to 90% chance of closing spontaneously. The chance of spontaneous closure decreases with age, and defects in children older than 5 years are more likely to increase in size.
Primum ASD
A primum ASD results from the septum primum failing to attach to the endocardial cushion and results in a defect located just above the atrioventricular valves anterior and inferior to the fossa ovalis.
Sinus Venosus Defect
A sinus venosus defect is a defect between the posterior-superior wall of the RA and one or both of the right pulmonary veins. This defect results in a left-to-right shunt from the right pulmonary vein to the RA. Physiologically, this left-to-right shunt is similar to the left-to-right shunting over an ASD. However, anatomically, a sinus venosus defect is not an ASD because there is no direct shunting over the atrial septum from the LA to the RA.
Coronary Sinus Defect
A coronary sinus defect results from “unroofing” of the coronary sinus, which wraps posteriorly around the heart, creating a channel for left atrial blood to enter the coronary sinus and empty into the RA.
Pathophysiology
Fig. 37.2 shows a brief summary of the pathophysiology of ASDs. A defect in the atrial septum allows for mixing of oxygenated and deoxygenated blood. The maximum shunt across the atrial septum occurs mainly during diastole when both atrioventricular valves are open, with the atria emptying into the ventricles. The compliance of the ventricles in turn will mainly determine the degree of shunting across the ASD. In a neonate, the right ventricle (RV) is more active, dominant, and muscular compared with the left ventricle (LV) because it has been functioning primarily as the systemic ventricle in utero, working against a relatively high PVR. As the PVR naturally falls with postnatal transition, the RV becomes less muscular with improved compliance. This results in an increase in left (systemic) to right (pulmonary) shunting postnatally during this expected transition. Thus the atrial-level shunting can be used indirectly as a marker for both RV and LV compliance in critically ill neonates. By comparing the flow pattern of the atrial-level shunt, one can assess changes in ventricular compliance.

Ventricular Septal Defects
Prevalence
Ventricular septal defects (VSDs) are the most common type of congenital heart disease diagnosed in neonates and infants. The incidence of VSDs ranges between 5 and 50 per 1000 live births.
Embryology and Anatomy
The ventricular septum anatomically separates the RV and LV. The RV comprises cardiomyocytes that originate from the secondary heart field and require the transcription factor Hand2 to develop. The LV develops from cardiomyocytes originating from the primary heart field, requiring the transcription factor Hand1 to develop.
The ventricular septum develops between days 27 and 37. Septation occurs as a result of three mechanisms: (1) Tissues from opposite ends expand and grow toward each other and ultimately fuse; (2) one end of a cavity grows actively toward the opposite side of the lumen; and (3) the two expanding portions of one end of the heart merge, creating a wall. However, this mechanism will never lead to full septation of the cavity. VSD is commonly seen in trisomy 13, 18, and 21 and Holt-Oram syndrome ( TBX5 mutation). There is an association between maternal marijuana and cocaine use, as well as paternal exposure to paint stripping, and VSD in the offspring. There are several ways in which the anatomic locations of VSDs are classified and named. This chapter will use the most common clinical division of inlet, outlet, perimembranous, and apical trabecular (muscular) VSDs.
Based on location, VSDs are classified into 4 subtypes ( Fig. 37.3 ):

Perimembranous VSDs
Perimembranous VSDs are sometimes also referred to as central VSDs and are located in the membranous part of the ventricular septum. Many of these defects touch the fibrous continuity region between the anterior mitral valve and the aortic valve. Often these VSDs can be restrictive because they are partially covered by accessory tricuspid valve (TV) tissue. Complications in the form of aortic valve regurgitation can occur if the right or noncoronary cusp prolapses into the VSD. In anticipation of surgery, it is always important to know where the conduction system runs in relation to the defect. In perimembranous VSD, the bundle of His passes just behind and below the VSD (along the posterior-inferior margins of the VSD).
Outlet VSDs
Outlet VSDs are located in the outflow portion of the tripartite RV and are associated with an absence or hypoplasia of the conal septum. The conal septum is a muscular structure that surrounds the pulmonary artery (PA) and defines the right ventricular outflow tract (RVOT). Because these defects are associated with the muscular conal septum, lack of and hypoplasia of that muscular septum changes the relative position between the PA, septum, and aorta. Therefore outlet VSDs are also referred to as malalignment VSDs, where anterior malalignment is seen in tetralogy of Fallot (TOF) and posterior malalignment is seen in interrupted aortic arch (IAA).
Inlet VSDs
Inlet VSDs are posteriorly located just below the atrioventricular valves. These are the types of VSDs seen in atrioventricular canal defects.
Muscular VSDs
Muscular VSDs are located within the muscular ventricular septum and have muscular borders all around. Their location often classifies them within the muscular septum and in relation to the moderator band. Midseptal VSDs are posterior to the septal band, anterior VSDs are anterior to the septal band, and the inferior muscular VSDs are deep in the septum close to the diaphragmatic surface.
Pathophysiology
The hemodynamic effect of the VSD is determined by the size of the defect and the relative resistance of the pulmonary versus the systemic hemodynamic systems ( Fig. 37.4 ).

Large VSDs
In the immediate neonatal period, the PVR is relatively high and limits the degree of left-to-right flow over a large nonrestrictive VSD. However, as the PVR physiologically decreases during the first several days of life, the left-to-right shunt progressively increases. This increase in blood shunting from left to right circulation results in more blood going to the pulmonary vascular bed and an increase in the blood volume coming back to the left side of the heart from the lungs. The pulmonary pressures can approximate systemic levels secondary to pulmonary overcirculation. The increased pulmonary venous return subsequently results in left ventricular hypertrophy, an adaptive measure to increase output and decrease ventricular wall tension. This left ventricular hypertrophy lowers the LV compliance and increases the left ventricular end-diastolic pressure (LVEDP). The decrease in LV compliance and increase in LVEDP results in left atrial dilatation and left atrial and pulmonary venous hypertension. Untreated, this will over time result in an increase in the hydrostatic pressure in the pulmonary capillary bed and the development of neonatal heart failure symptoms by the accumulation of pulmonary interstitial fluid.
Small VSDs
Small VSDs usually have restrictive pressures and limit the left-to-right shunting once the PVR drops in the neonatal period. The left-to-right volume load is much less, and the compensatory mechanisms described for large VSDs do not typically develop. The pulmonary vascular flow and the pulmonary vascular pressures remain low, and the risk of developing pulmonary vascular disease in turn is lower. There is, of course, a clinical spectrum of VSDs between the pathophysiology of a large nonrestrictive VSD and a small restrictive VSD. Therefore every VSD warrants close monitoring of clinical status and follow-up. The hemodynamic assessment of VSDs can be challenging in preterm infants and neonates with other morbidities, requiring extended hospitalization and prolonged ventilation. Notable long-term complications after VSD repair may include double chamber RV, subaortic membrane resulting in left ventricular outflow tract (LVOT) stenosis, and aortic insufficiency secondary to valve prolapse.
Atrioventricular Canal Defects
Prevalence
Atrioventricular canal (AVC) defects are commonly associated with trisomy 21 and heterotaxy. Patients with normal chromosomes can also develop AVC defects, but it is 1000 times more common in neonates with trisomy 21, with about 50% of all patients with AVC defects having trisomy 21. In patients with heterotaxy syndrome, the AVC defect association is mostly with right atrial isomerism and asplenia rather than left atrial isomerism and polysplenia. AVC defects can also be seen with higher prevalence in patients with Ellis–Van Creveld syndrome.
Embryology and Anatomy
In the embryonic heart, one valve connects the atrium to the ventricle, which is called the common atrioventricular (AV) valve. This one common valve is divided by muscle tissues that grow toward each other on opposite sides of the common AV valve. This muscle tissue is called the endocardial cushion. Under normal development, the endocardial cushion divides the common AV valve into the left-sided mitral and right-sided TVs. Failure of the endocardial cushions to grow toward each other and fuse to form the AV septum results in one common AV connection. It is believed to be a defect in a cell adhesion molecule that results in the failure of the endocardial cushion to approximate and ultimately fuse to divide the common AV valve. The common AVC defect classically consists of a primum ASD, an inlet VSD, and a common AV valve consisting of five leaflets.
In a normal heart, the AV septum and the hinge points of the AV valves make it such that the mitral valve is always superior to the TV, which defines the AV septum. With a failure to form the AV septum, the left-sided AV valve is displaced downward, placing the right and left sides on the same level. Having the right and left AV valves on the same level is a pathognomonic finding of an AVC defect. Another feature specific to AVC defects is an elongated LVOT. This characteristic shape of the LVOT in AVC defects is often called a “gooseneck” deformity. The elongated LVOT is why a late complication of AVC repair is progressive subaortic stenosis. Fig. 37.5 shows the anatomic abnormalities in AV canal defects.

Classifications of the AV Canal
Complete AV Canal
The complete form of AV canal has an atrial-level defect and an intraventricular defect. Typically, the entire membranous septum is deficient, resulting in shunting at both an atrial and ventricular level. There is also just one common AV valve with five leaflets. It has one anterior and posterior bridging leaflet extending the entire length of the valve. There are two lateral leaflets and one right-sided anterior leaflet, which represent what would have been the anterior tricuspid leaflet.
Intermediate AV Canal
There is a division of the right- and left-sided AV valves but a large primum ASD, and a significant VSD component persists.
Transitional AV Canal
A transitional AV canal is the formation of left- and right-sided AV valves with a large primum ASD but a small VSD component.
Partial AV Canal
A partial AV canal has division of the right and left AV valves and no VSD component, just a primum ASD. Anatomically, the right and left AV valves are not normal in the intermediate, transitional, and partial canals. They are on the same level, and there is a variable degree of tissue connecting the anterior and posterior bridging leaflets.
The Rastelli classification is based on how and mostly where the anterior bridging leaflet is attached and where it divides between the left superior leaflet and the right superior leaflet ( Fig. 37.6 ).
-
Type A: The anterior bridging leaflet is divided at the level of the ventricular septum and has chordal attachments to the septum. This is the most common form of AV valve in AVC defects and is primarily found in patients with trisomy 21.
-
Type B: The anterior bridging leaflet is not divided at the level of the ventricular septum and continues over to the RV, where it is attached with chordae to a papillary muscle located on the right side of the septum. This is the least common form of AVC defect.
-
Type C: The anterior bridging leaflet extends over into the RV, is not attached to the ventricular septum, and is attached to chordae extending to papillary muscles located on the free wall of the RV. This type of AV valve is most commonly found in TOF and AVC defects.

The pathophysiology of AV canal defects is summarized in Fig. 37.7 . A panoramic view of left-to-right shunting lesions is shown in Fig. 37.8 .


Outflow Septation and Conal Defects
Tetralogy of Fallot
TOF is historically defined by its four distinct findings: pulmonic valve (PV) stenosis, VSD, overriding aorta, and right ventricular hypertrophy. These characteristics of TOF are all a result of the unique VSD found in TOF alone. Therefore the four features result from a single primary defect (sometimes referred to as monology of Fallot)—the anterior malalignment cono-ventricular VSD. This malalignment of the conal septum results in a wide communication between the RV and LV.
The RVOT obstruction seen in TOF is highly variable in both its severity and location. The obstruction is frequently found at many levels, starting from the superior muscular lip of the VSD to the narrow subvalvar infundibular region, with a frequently thickened, often bicuspid and stenotic pulmonary valve and supravalvar stenosis being common. Various degrees of anatomic abnormalities resulting in stenosis of the pulmonary vasculature tree are frequently present. This multilevel obstruction to pulmonary blood flow is very characteristic of TOF.
Figs. 37.9 and 37.10 show a typical and a simplified version of the anatomy of TOF lesions, respectively. Fig. 37.11 highlight the pathophysiology of these anomalies, and the changes seen during cyanotic spells.



The clinical spectrum of TOF based on anatomy can be very broad, from VSD with almost no RVOT obstruction or PV stenosis to variable degrees of pulmonary valve stenosis. Occasionally, PV can be so stenotic that the pulmonary circulation may become dependent on systemic to pulmonary blood flow over the ductus. The takeoff of the left pulmonary artery is often stenotic because it has a variable degree of ductal tissue that can result in narrowing and anatomic distortion. TOF is commonly associated with a right aortic arch, meaning the arch crosses the right bronchus in 25% of cases, often with mirror image branching. The first branch is the left-sided brachiocephalic artery, which divides into the left subclavian and left carotid arteries. Approximately 7% of patients with TOF will have abnormal origin and course of the coronary arteries. The coronary artery anatomy must be thoroughly understood before surgery to minimize the risk of damage to the coronary arteries, especially because 4% of patients may have a left anterior descending artery originating from the right coronary artery, which in turn crosses the RVOT in the exact area where a transannular patch may be placed.
Because TOF with pulmonary atresia has no anterograde blood flow through the RVOT, its only source of pulmonary blood flow is through major aortopulmonary collaterals (MAPCAS) or a patent ductus arteriosus (PDA). The right and left pulmonary arteries can be confluent or nonconfluent if the collateral feeds a confluent main pulmonary artery, the “seagull” sign classic when contrast is injected in the aorta or collateral. MAPCAS are structured and function more like systemic arteries compared with pulmonary arteries; they are frequently stenotic and can be reactive. MAPCAS refers to multiple collaterals feeding the pulmonary tree and one single systemic artery feeding a pulmonary segment leading to a unifocal blood supply.
Transposition of the Great Arteries
Prevalence
The prevalence of dextro-transposition of the great arteries (D-TGA) is 4.7 per 10,000 live births and accounts for 3% of all congenital heart disease and 20% of cyanotic heart disease. It is more common in males and is generally not associated with any specific chromosomal anomalies or syndromes. It is associated with heterotaxy—in particular, right isomerism. D-TGA has also been associated with infant of a diabetic mother (IDM), maternal use of vitamin A and ibuprofen, and exposure to organic solvents and pesticides.
The pathogenesis of D-TGA results from failure of the conal tissue below the pulmonary valve to develop and failure of the conal tissue below the aortic valve to regress. This results in failure to position the pulmonary valve anteriorly and committed to the RV as it migrates during the RV and RVOT muscle growth. The most commonly associated cardiac lesion in D-TGA is a VSD. D-TGAs are frequently characterized as either with an intact ventricular septum (IVS) or with a VSD. Most D-TGAs will not have a VSD and have a normal aortic arch. A D-TGA with an accompanying VSD is at risk of having various degrees of LVOT obstruction, often associated with a malalignment VSD, pulmonary valve stenosis, septal hypertrophy, subpulmonary membrane, or accessory mitral valve tissue. The anatomical changes in D-TGA are shown in Fig. 37.12 .

The coronary arteries in D-TGA originate from the anteriorly positioned aorta and the sinuses facing the pulmonary valve. There are several described variations of the coronary arteries in D-TGA. The most common coronary artery pattern is the left anterior descending artery from the left-facing sinus giving rise to the left circumflex and the right coronary artery originating from the right-facing sinus. The next most common pattern is the circumflex coming off the right coronary artery (RCA) with an otherwise normal anatomic path.
A Note on the Conus
The conus is a muscular structure that in embryology surrounds the base of the truncus. The conus plays an essential role in the septation of the RVOT, and the structure continues to embed the base of the PA and involutes around the aorta. In doing so, it moves the PA anteriorly and slightly to the left of the aorta. The involution of the conal tissue around the aorta allows for the anterior leaflet of the mitral valve and the aorta to be in fibrous continuation. The conus is mainly associated with the RV. Therefore the more conal tissue is located below the great vessel, the more likely that great vessel will be associated with the RV. Congenital heart diseases that result from developmental problems with the conus are TOF, double outlet right ventricle (DORV), truncus with IAA, and D-TGA. In TOF, the conus is mainly associated with the RA; with DORV, there is a variable conus under both the PA and the aorta. There is conal tissue between the aorta and mitral valve. In D-TGA, there is no conus associated with the aorta, and there is no conus under the PA.
Malalignment refers to the position of the outflow septum in relation to the rest of the muscular septum. Anterior malignment means that the superiorly located outflow tract is shifted in an anterior (and often superior) position. The terminology here is essential as the anterior malalignment VSD is uniquely associated with TOF and DORV, whereas a posterior malalignment VSD is associated with an IAA.
Double Outlet Right Ventricle
Prevalence
DORV is usually not associated with any specific genetic anomalies and accounts for less than 1% to 3% of congenital heart defects. Obler et al. reported that a large number of genes have been associated with DORV in both humans and animal models. The different anatomic subtypes seen in specific etiologies suggest several distinct pathogenetic mechanisms for DORV, including impairment of neural crest derivative migration and impairment of normal cardiac situs and looping. The large number of genes associated with DORV in both humans and animal models and the different anatomic subtypes seen in specific etiologies indicate the likelihood of several distinct pathogenetic mechanisms for DORV, including impairment of neural crest derivative migration and impairment of normal cardiac situs and looping.
DORV tends to be described by one of two definitions. One definition is that both the great arteries are 50% or more committed to the RV. The PA will need to be committed to the RV 50% or more than in TOF-like anatomy. Another frequently used definition based on echocardiography is that there is conal tissue located between the aorta and the anterior leaflet of the mitral valve, lacking the classic fibrous continuation. DORV, thus, is a conotruncal defect where the location of the great arteries in relation to each other defines the type of DORV and the physiology. The location of the great arteries depends on the location of the conus as the conus moves its associated great artery anteriorly and superiorly. DORV anatomy can range from anatomy and physiology similar to TOF, where there will be a subaortic VSD, to anatomy and physiology of a TGA where the VSD will likely be in a subaortic location. The VSD is located in the conoventricular septum stretching from the membranous septum into the upper part of the muscular ventricular septum. Pulmonary valve or subvalvular pulmonary stenosis is found in 50% of patients with DORV, and 25% of DORV hearts will have a secundum ASD. The anatomical changes characteristic of DORV are shown in Fig. 37.13 .

Truncus Arteriosus
Truncus arteriosus is the resulting failure of the conus and neural crest cell migration to septate the truncus into the aorta and the main pulmonary artery. This results in a single artery leaving, giving rise to the coronary arteries, the aortic arch with head and neck vessels, and the pulmonary arteries.
Prevalence
Truncus arteriosus is seen with an annual incidence of 7 per 100,000 live births, and although it accounts for less than 1% of all congenital heart lesions, it accounts for 4% of critical congenital heart defects.
The anatomical changes in tricuspid atresia (TA) are shown in Fig. 37.14 ; the lesions are classified based on the anatomy of the origin of the pulmonary arteries off the TA. ,
-
Type 1: Type 1 is the most common type of truncus, accounting for approximately 50%. A short main pulmonary trunk comes off the truncus, giving rise to the left and right pulmonary arteries.
-
Type 2: The second most common form of truncus, type 2 accounts for approximately 40%. There is no main pulmonary trunk, and the right and left pulmonary arteries arise directly from the truncus. In type 2, they arise adjacent to each other.
-
Type 3: Type 3 accounts for approximately 10% of truncus. It is anatomically very similar to type 2, but the right and left pulmonary arteries arise at variable distances and locations from each other.

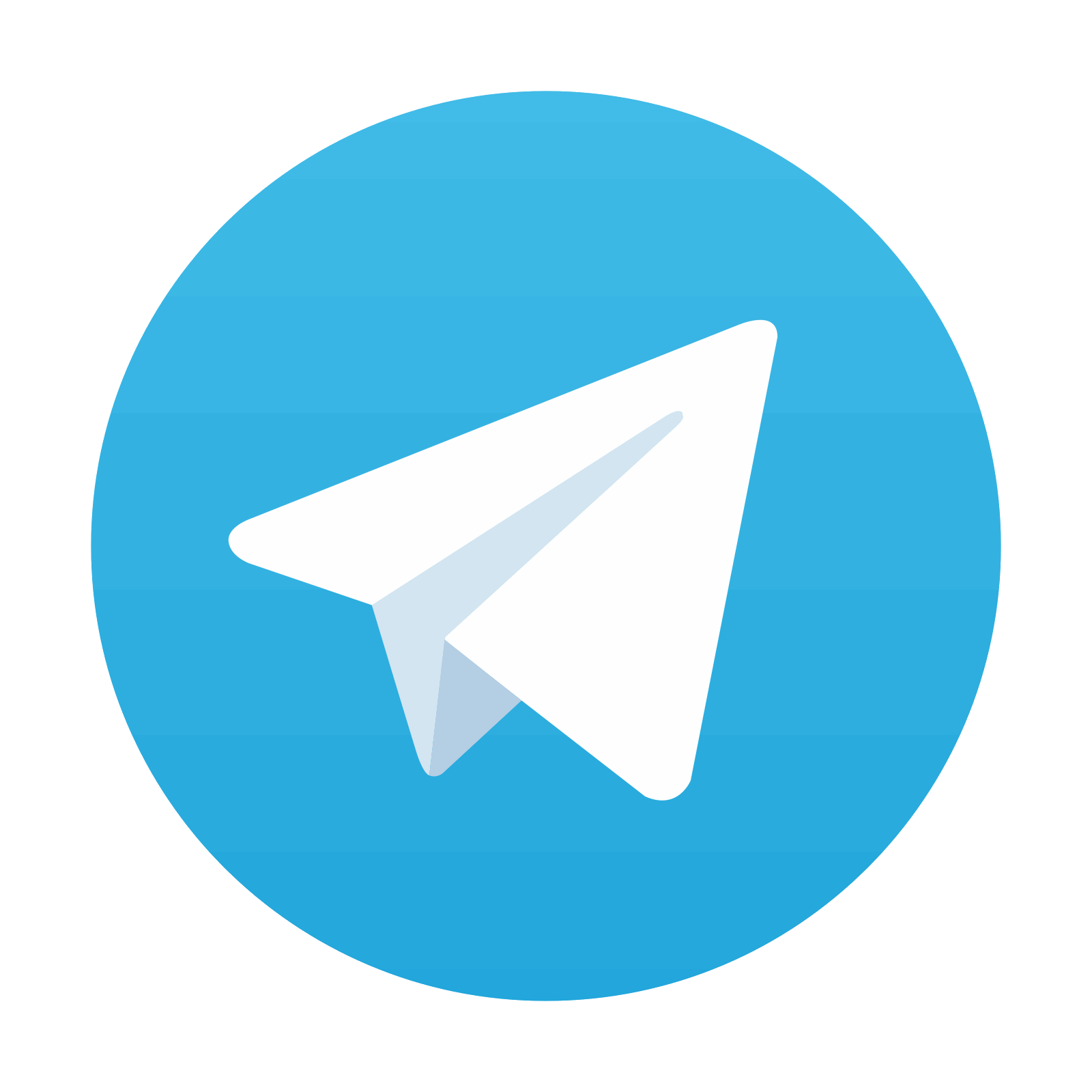
Stay updated, free articles. Join our Telegram channel

Full access? Get Clinical Tree
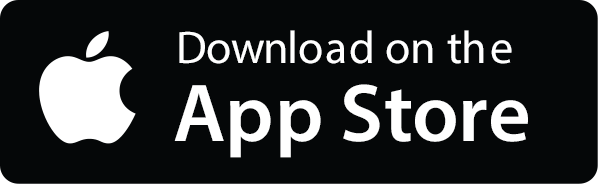
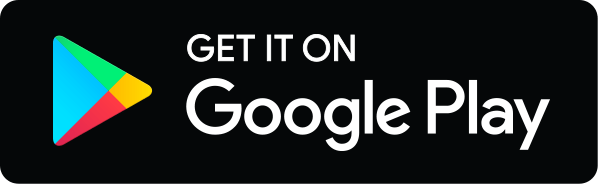