Bronchopulmonary Dysplasia
EPIDEMIOLOGY
Chronic lung disease (CLD) of the newborn, also known as bronchopulmonary dysplasia (BPD), is the most common CLD in early infancy.1 The incidence of BPD varies between newborn care centers, reflecting differences in patient population and infant management practices.2–4 Recent publications reported a BPD incidence of 68% in very low birth weight (401- to 1500-g) infants born prior to 29 weeks’ gestation or 77% in infants born at less than 32 weeks’ gestation with a birth weight below 1 kg.3,5,6 The latest studies in Europe reported a BPD rate of up to 25% in infants below 32 weeks’ gestational age (GA).2 The overall incidence in the United States is approximately 10–15,000 cases per year.7 The incidence of BPD is inversely related to GA, varying from 80% or more among the most immature infants at 24 weeks’ gestation to less than 5% among infants greater than 32 weeks’ gestation.2,3,8 Infants who are born very prematurely often require prolonged assisted ventilation to treat acute respiratory failure caused by primary surfactant deficiency (ie, respiratory distress syndrome, RDS), sustained or recurrent apnea, or infections. BPD also can develop in term infants who are treated with long-term mechanical ventilation for respiratory failure from meconium aspiration, bacterial or viral pneumonia, lung hypoplasia, or cardiopulmonary malformations.
Although the rate of severe BPD was found to decrease between 1994 and 2002, the overall incidence did not change.7 The incidence of long-term sequelae remained unchanged or increased among the most immature infants,9 presumably because of a significant reduction of mortality rates.
PATHOPHYSIOLOGY
Definitions
Bronchopulmonary dysplasia, as a pulmonary disease following mechanical ventilation of hyaline membrane disease, was first described 1967 by Northway et al.10 In 1989, the Bureau of Maternal and Child Health and Resources Development proposed the following diagnostic criteria for BPD: (1) Requirement for positive pressure ventilation during the first 2 weeks of life, (2) minimum requirement of ventilation for 3 days, (3) clinical signs of respiratory compromise persisting beyond 28 days of age, (4) requirement for supplemental oxygen beyond 28 days of age to maintain a PaO2 of greater than 50 torr, and (5) chest radiographs showing characteristic lung abnormalities (diffuse bilateral densities, often associated with areas of hyperinflation). More recently, the definition has been modified to specify a need for supplemental oxygen for greater than 28 days or beyond 36 weeks’ postmenstrual age (PMA), accompanied by characteristic radiographic abnormalities.
This and subsequent modifications of the earlier definition were initiated by substantial changes in the BPD patient cohort, where application of different ventilation regimens and other significant changes in treatment procedures yielded not only an increased survival rate in the most immature infants but also a different appearance of lung injury, with the characteristic arrest of lung development at a very early stage. This form of the disease was called “new BPD.”
In 2000, a workshop on BPD sponsored by the National Institutes of Health (NIH) proposed an extensive definition that uses O2 dependency at 36 weeks’ postconceptual age (failure to maintain normal O2 saturation when breathing room air) and defined mild, moderate, and severe disease (Table 29-1). This definition “corrects” for the degree of immaturity in the moderate and severe stages of the disease as the definition refers to 36 weeks’ postconception.1 A study investigating the predictive validity of the severity-based, consensus definition of BPD by identifying a spectrum of risk for adverse pulmonary and neurodevelopmental outcomes in early infancy supported the more refined definitions of BPD grades according to need for supplemental O2 or positive pressure breathing at 36 weeks’ PMA.6
Table 29-1 Degrees of Bronchopulmonary Dysplasiaa
Pathophysiologic Findings
A variety of experimental and clinical studies have shown that BPD is characterized by a decrease in alveolarization accompanied by a decrease in small-vessel development.11 Structural and molecular abnormalities of the lung in BPD include increased smooth muscle in small pulmonary arteries and airways, decreased numbers of terminal respiratory units (alveoli and alveolar septa), and a corresponding increase in alveolar size. When compared to cases treated in the presurfactant era, the amount of interstitial fibrosis is substantially less and tends to be more diffuse in the new BPD, associated with a partial-to-complete arrest in alveolarization12 (Table 29-2; Figures 29-1 and 29-2).
Table 29-2 Pathophysiologic Characteristics of “Old” and “New” Bronchopulmonary Dysplasia (BPD)
FIGURE 29-1 Histologic characteristics of “old” bronchopulmonary dysplasia. Infants not treated with surfactant display the “classic” features of long-standing healed bronchopulmonary dysplasia (LSHBPD), acini with severe alveolar septal fibrosis (top) adjacent to those with little or no septal fibrosis (bottom) (hematoxy and eosin; original magnification ×20). (Reproduced with permission from Husain AN, Siddiqui NH, Stocker JT. Pathology of arrested acinar development in postsurfactant bronchopulmonary dysplasia. Hum Pathol. 1998;29(7):710–717.)
FIGURE 29-2 Histologic characteristics of “new” bronchopulmonary dysplasia (BPD). In the latest histological manifestation of BPD, surfactant-treated infants develop little or no alveolar septal fibrosis. The acini, however, display expanded, simplified alveolar ducts, saccules, and alveoli (hematoxy and eosin; original magnification ×25). (Reproduced with permission from Husain AN, Siddiqui NH, Stocker JT. Pathology of arrested acinar development in postsurfactant bronchopulmonary dysplasia. Hum Pathol. 1998;29(7):710–717.)
Different processes were found to have an impact on lung development through alteration of growth factor signaling, apoptosis, and profound changes to the extracellular matrix (ECM). Figure 29-3 gives an overview of the pathophysiologic processes that contribute to the development of BPD. Injury to the lungs of preterm infants who later present with BPD takes places in the canalicular to the saccular stages of lung development, long before the general presence of defined alveolar structures as seen in term infants (Figure 29-4). Inflammatory processes are considered crucial events for the initiation of BPD. The condition may be associated with chorioamnionitis before birth, systemic infection after birth, or pulmonary accumulation of activated neutrophils, which has been described in RDS, aspiration syndromes, pneumonia, and prolonged application of positive pressure ventilation.13 The characteristic influx of neutrophils into the lung is accompanied by increased numbers of macrophages in the course of the disease.14–16 Deficiency of antioxidant enzymes and inhibitors of proteolytic enzymes render the very immature infant’s lungs especially vulnerable to the effects of toxic oxygen metabolites and proteases released by activated neutrophils and macrophages.17–20 Pulmonary inflammation and the associated increase in protease activity then leads to the degradation of lung elastin in infants with BPD, manifested as increased urinary excretion of desmosine, a breakdown product of the mature elastic fiber.21–23
FIGURE 29-3 Bronchopulmonary dysplasia (BPD) pathophysiology. Pathological processes in the development of BPD as identified by clinical and experimental studies. HIF, hypoxia inducible factor; IL, interleukin; MMP, matrix metalloproteinase; PDGFR, platelet-derived growth factor receptor; TGF, transforming growth factor; TNF, tumor necrosis factor; VEGFA, vascular endothelial growth factor A; VEGFR, vascular endothelial growth factor receptor.
FIGURE 29-4 Stages of lung development. Progress from canalicular to alveolar stage in lung development. Upper row: Histological findings in preterm infants. Middle row: Schematic of lung budding to the formation of alveoli. Lower row: Corresponding age of fetus (p.c. postcongestional). The red arrow indicates the time of injury for the preterm lung in infants later developing BPD. (Adapted with permission from Whitsett JA, Wert SE, Trapnell BC. Genetic disorders influencing lung formation and function at birth. Hum Mol Genet. 2004;13.)
Following increased remodeling of the ECM, lungs of infants with BPD exhibit increased abundance and abnormal distribution of elastin in the pulmonary parenchyma and circulation, with increased pulmonary expression of tropoelastin (Figure 29-5)24,25. Changes to the structural integrity of the ECM potentially affect its function as a scaffold for the formation of new alveoli and capillaries. The release of various cytokines, activation of transcription factors (eg, nuclear factor kappa B [NF-kB]), and signaling of growth factors such as transforming growth factor (TGF) β lead to a characteristic increase in apoptosis.26 The process of deficient alveolarization is characteristically associated with the presence of dysmorphic capillaries and an altered pattern of angiogenic growth factors (eg, a reduced pulmonary expression of vascular endothelial growth factor [VEGF] and VEGF receptors).27–29 These changes are accompanied by diminished endothelial nitric oxide synthase (eNOS) and soluble guanylate cyclase (sGC) in lung blood vessels and airways,30,31 contributing to the development of pulmonary hypertension and affecting lung lymphatic drainage.32,33 Relevant factors in vascular abnormalities are depicted in Figure 29-6.
FIGURE 29-5 Extracellular matrix remodeling in bronchopulmonary dysplasia. Left: En face section through the lung septal wall of a 28-week postconceptional age (PCA) infant on mechanical ventilation at 31 days of life. The fibers appear generally coarser, but the pattern is disorganized, with spiraling fibers. Right: En face section through the septal wall of a 24.4-weeks’ gestational age infant who died at a PCA of 28.8 weeks. The secondary collagen fibers are disorganized, tortuous, and thickened (arrow) (section 40 μm thick; Gomori reticulum stain; ×100 oil). (Reproduced with permission from Thibeault DW, Mabry SM, Ekekezie II. Collagen scaffolding during development and its deformation with chronic lung disease. Pediatrics. 2003;111(4 Pt 1):766–776.)
FIGURE 29-6 Vascular abnormalities in bronchopulmonary dysplasia (BPD). Characteristic factors indicating impaired vessel development in BPD.
The Clinical Picture
Clinically, BPD is characterized by persistent oxygen requirement accompanied by varying signs of respiratory distress, including tachypnea, nasal flaring, and use of accessory muscles with intercostal and substernal retractions, grunting, and cyanosis. Impaired respiratory gas exchange (ie, hypoxemia with need for supplemental O2 and alveolar hypoventilation with resultant hypercapnia) leads to a mismatch of ventilation and perfusion.3534 The increased lung vascular resistance, typically associated with impaired responsiveness to inhaled nitric oxide (iNO) and other vasodilators, can progress to reversible or sustained pulmonary hypertension and right heart failure.32,33 Lung function at term shows increased respiratory tract resistance and hyperreactive airways,35 which can manifest as episodic bronchoconstriction and cyanosis. Diminished lung compliance, tachypnea, and increased minute ventilation and work of breathing are accompanied by increased lung microvascular filtration pressure that may lead to interstitial pulmonary edema.
Risk Factors
Risk factors for the development of BPD as identified by clinical and experimental studies bear their impact on the structure and function of the immature lung beyond the background of genetic susceptibility (Figure 29-7). Factors directly related to preterm birth, such as the presence of a patent ductus arteriosus (PDA) or the immaturity of the immune system, rank next to independent pre- and postnatal risk factors such as gender or growth retardation. Studies in twins and preterm infants with similar environmental risk factors suggested that genetic susceptibility may play a role in the pathogenesis of the disease.36–38 After controlling for covariates, genetic factors accounted for 53% of the variance in liability for BPD in one study investigating twin preterm infants.39 Furthermore, male preterm infants are at a higher risk for the development of long-term impairment, including BPD,40 and hormonal regulation has been discussed as an underlying cause.41 With respect to prenatal determination of pulmonary outcome, intrauterine growth retardation increases the risk of BPD 3- to 4-fold,15,42–44 most likely through alteration of growth factor signaling and impaired alveolar and vascular growth.45 In addition, the fetal inflammatory response syndrome as well as the full picture of amnion infection with histologic signs of chorioamnionitis are known to increase the risk of BPD.13,46–48
FIGURE 29-7 Risk factors for the development of bronchopulmonary dysplasia (BPD). ECM, extracellular matrix.
After birth, the presence of congenital and nosocomial systemic or pulmonary infections is an important risk factor for the development of the disease.49,50 Prolonged mechanical ventilation using large tidal volumes and high inflation pressures together with oxygen toxicity related to the magnitude and duration of exposure to supplemental oxygen are major risk factors for the development of BPD.51,52 The development of pulmonary complications (eg, air leaks, interstitial emphysema, and pneumothoraces) further increases the risk.51
Clinical conditions (eg, gastroesophageal reflux) leading to aspiration of gastric contents and associated aspiration pneumonitis, incorrect placement of the endotracheal tube or improper delivery of surfactant, airway obstruction from mucous plugs, and prolonged airway sucking need to be monitored closely as they may add to the overall risk by inducing acute lung injury and nonuniform lung expansion. Furthermore, excessive fluid and salt intake, the presence of a PDA as well as myocardial dysfunction increase the risk for BPD through induction of lung edema.53–57 Poor nutritional support, vitamin A deficiency, and insufficient adrenal and thyroid hormone release in the very premature infant contribute to the development of the disease.58–60 Table 29-3 gives an overview of relevant risk factors for the development of BPD.
Table 29-3 Risk Factors for the Development of Bronchopulmonary Dysplasia (Increase in Relative Risk, RR)a
DIFFERENTIAL DIAGNOSIS
The differential diagnosis for BPD mainly comprises interstitial lung diseases (ILDs) in children, which have been categorized in a new classification system.61 Figure 29-8 is an overview of the diseases that have to be considered. Neonatal CLD accounts for many cases in the group of pulmonary growth abnormalities that reflect deficient alveolarization. Chromosomal disorders leading to defective lung growth, as well as pulmonary hypoplasia associated with birth defects such as diaphragmatic hernia, have to be included in the differential diagnosis. In the group of surfactant dysfunction disorders, lung diseases, caused by mutations in the surfactant protein C and the adenosine triphosphate (ATP) binding cassette transporter A3 (ABCA3) genes, both necessary for surfactant metabolism, have been shown to cause the picture of alveolar proteinosis and desquamative interstitial pneumonia (DIP).62–64 Besides these, neuroendocrine cell hyperplasia of infancy and pulmonary interstitial glycogenosis, although of undefined etiology, need to be included in diagnostic considerations.61 When recurrent infections occur, special diagnoses concerning the immunocompromised host, including most common opportunistic infections, need to be taken into account. Furthermore, several disorders that masquerade as ILD, such as congenital heart diseases, arterial hypertensive vasculopathy, or congenital lymphatic disorders, need to be considered.
FIGURE 29-8 Classification system of diffuse lung disease in children. The study cohort was composed of patients under the age of 2 years who had a diagnostic lung biopsy during the designated 5-year interval; exclusion criteria are listed. The clinical–pathologic classification scheme is detailed with numbers of cases and specific entities identified within each category. Some unusual entities not seen are also listed, but there are clearly other entities associated with diffuse lung disease not seen in this cohort that have not been listed. (Reproduced with permission from Deutsch GH, Young LR, Deterding RR, et al. Diffuse lung disease in young children: application of a novel classification scheme. Am J Respir Crit Care Med. 2007;176(11):1120–1128.)
The lack of the typical history, including extreme prematurity followed by mechanical ventilation, the presence of a suspect family history of (undiagnosed) pulmonary diseases, or signs for additional malformations, should lead to consideration of other differential diagnoses.
DIAGNOSTIC TESTS
The diagnosis of BPD is based on pulmonary function at term combined with the characteristic history or preterm birth and ventilator support. Oxygen requirement to maintain oxygen saturation above 85% is accompanied by an increase in pCO2 (partial pressure of carbon dioxide) and bicarbonate in blood gas analyses.65 Besides clinical signs, these measures of gas exchange are the main follow-up parameters. Lung function testing is used in some clinical studies but has not been introduced into the clinical routine yet. Chest radiographic appearance includes the presence of fibrosis/interstitial shadows, cystic elements, and hyperinflation at 7 days of age and was shown to facilitate prediction of outcome of infants born very prematurely.66 In this study, infants who died before discharge differed significantly from the rest with respect to significantly higher scores for cysts. Compared to radiologic findings in the presurfactant era, infants with new BPD show more subtle radiographic abnormalities.67
Findings in high-resolution computed tomography (HRCT) include linear and reticular opacities, areas of architectural distortion, and gas trapping/hyperaeration (Figure 29-9). Depending on the respective BPD definition applied, lung function abnormalities seem to be reflected by changes in chest radiographs or HRCT, showing fibrotic changes/interstitial shadowing to a varying degree, in some studies accompanied by (local) hyperinflation.68–73
FIGURE 29-9 High-resolution computed tomography: findings in bronchopulmonary dysplasia (BPD). Structural changes in the lung of BPD patients. (Used with permission of Prof. Dr. Irwin Reiss, Intensive Care and Department of Pediatric Surgery, ErasmusMC–Sophia Children’s Hospital, Rotterdam, the Netherlands.)
Use of current magnetic resonance imaging (MRI) techniques, as applied in infants with chronic diaphragmatic hernia to estimate lung volume, would allow reducing exposure to radiation but is not part of clinical routine yet.74 Advances in computed tomographic (CT) scanning or the application of newer techniques such as hyperpolarized gas MRI may increase the clinical role for imaging technologies in the diagnostic regimen for BPD.
Right heart function is assessed by echocardiography, and pulmonary pressure can be estimated when tricuspidal insufficiency is present. In severe cases, right heart catheter procedures might be indicated. The degree and reversibility of lung vascular resistance can be estimated by testing the responsiveness to iNO and other vasodilators such as oxygen.32,33
Regarding potential differential diagnoses, the workup needs to include genetic consultation, probable lung biopsy or lavage under critical consideration of the clinical picture, the patient history, and findings from imaging technologies.
MANAGEMENT
The best approach for preventing or reducing the severity of BPD is to adopt strategies that minimize or avoid conditions that likely contribute to lung injury.
Prenatal Management
Pre- or perinatal management critically affects not only the rate of preterm delivery but also the development of severe long-term sequelae, including BPD.47 Antenatal glucocorticoid treatment is a major stimulant of lung maturation. Preterm infants whose mothers received a complete and timely course of antenatal steroids (ie, 2 doses of betamethasone more than 24 hours prior to birth, with the last dose given no earlier than 7 days before birth) had a significantly reduced risk of developing BPD.75 A benefit of this treatment was also shown for incomplete courses of glucocorticoids, and stratification by gender and birth weight at 1 kg showed a benefit of therapy in all strata except that of extremely low birth weight male infants.75 A meta-analysis in 2000 did not find enough evidence for the prevention of BPD.76 With respect to repetitive doses of antenatal steroids, a meta-analysis recommended considering another course of treatment in women at risk of preterm birth 7 or more days after an initial course, in view of the neonatal benefits.77,78 Although these neonatal benefits were associated with a small reduction in size at birth, up to current evidence no significant harm—or benefit—of the treatment regimen was observed in early childhood.
Prenatal infection/inflammation significantly contributes to the development of BPD, indicating careful consideration of either prolongation of pregnancy under maternal antibiotic and tocolytic treatment or the decision for preterm delivery.79,80 As experimental studies and clinical findings suggested, strategies such as stimulation of lung growth through infectious agents such as lipopolysaccharides, tolerance of “low-grade” infections, or prevention of severe amnion infection syndrome need to be weighed against each other.81 As markers to determine a “dose” and timeline in management of prenatal infections are missing, careful individual prenatal case management is needed.
Surfactant Replacement and Ventilation Regimen
The introduction of surfactant replacement into the care of preterm infants reduced mortality in very immature infants and considerably changed the picture of BPD. A meta-analysis showed that postnatal treatment with animal-derived surfactant extracts reduced the risk for BPD or death at 28 days of age.82 This can be considered to be the an effect of a reduction in the severity of acute lung disease after premature birth and the resultant need for long-term mechanical ventilation with high peak inflation pressure (PIP). In line with this, early surfactant replacement therapy with extubation to nasal continuous positive airway pressure (NCPAP) was found to be associated with a lower incidence of BPD, less need for mechanical ventilation, and fewer air leak syndromes compared with later selective surfactant replacement and continued mechanical ventilation.83 Furthermore, lower treatment thresholds (forced inspiratory oxygen [FIO2] > 0.45) conferred greater advantage in reducing the incidences of air leaks and BPD.83
To minimize ventilator-induced changes to the developing lung, assisted ventilation with low PIP, relatively short inspiratory times, and sufficient positive end-expiratory pressures (PEEPs) leading to high-frequency and low tidal volumes have been shown to be beneficial for the preterm lung.84,85 A recent meta-analysis of 9 studies investigating the effect of pressure-limited and volume-targeted ventilation showed that volume-targeted ventilation reduced death/BPD, duration of ventilation, pneumothoraces, hypocarbia, and periventricular leukomalacia/severe intraventricular hemorrhage (IVH).51 Adequate heating and humidification of the inspired gas and caution with endotracheal tube suctioning that provokes lung collapse are important steps in the prevention of airway injury.86 Although application of NCPAP directly after birth reduced the incidence of the need for oxygen at 28 days as well as the number of days of ventilation in infants born at 25 to 28 weeks’ gestation,87 this approach did not significantly reduce the rate of death or BPD when compared to primary intubation. However, extubation to nasal intermittent positive pressure ventilation (NIPPV) or NCPAP in the first postnatal week was found to be associated with decreased probability of BPD/death.88
Treatment with a respiratory stimulant, specifically caffeine, reduces the risk of apnea and recently was shown to reduce the incidence of BPD.89 Inspired O2 concentrations need to be targeted not only to prevent hyperoxia (ie, O2 saturation above 96% or PaO2 above 80 mm Hg) but also to avoid hypoxemia (O2 saturation < 80% or PaO2 < 50 mm Hg), which causes pulmonary hypertension; increased fluid filtration (lung edema, potentially through lung epithelial sodium transport); increased airway resistance; and inhibition of alveolar and pulmonary vascular growth. A recent meta-analysis and systematic review of all published randomized studies concluded that a low compared with a high saturation reduced the relative risk of BPD in preterm babies by about 4% to 10%.52,90 However, as 2 important trials reported slightly increased mortality rates for the group with low oxygen saturation, O2 saturation between 85% and 89% cannot currently be recommended in immature babies.91 The optimal O2 saturation is presently not known; current practice is to keep the O2 saturation between 85% and 95% and PaO2 between 50 and 80 mm Hg.
Despite a good rationale for the application of permissive hypercapnia (PaCO2 55 or 60 cm H2O, with a pH of 7.25 or greater) to reduce lung injury in preterm infants during mechanical ventilation in the first week of life, a recent report showed an increased rate of BPD in infants with hypercarbia during that period of time.92 Other studies showed no apparent benefit of this approach for infants between 23 and 28 weeks’ gestation.93,94
To reduce oxygen free radical injury, administration of superoxide dismutase was evaluated in infants at risk to develop BPD, but the study failed to detect any benefit of this therapy.95
Fluid Management
Restricting fluid and salt intake and allowing for diuresis and a progressive loss of about 10% body weight in the first week of life has been shown to reduce the risk of BPD.53,54 Excessive fluid and heat losses in the very immature infant should be minimized during the first days after birth by keeping the infant in a warm, humidified chamber.96–
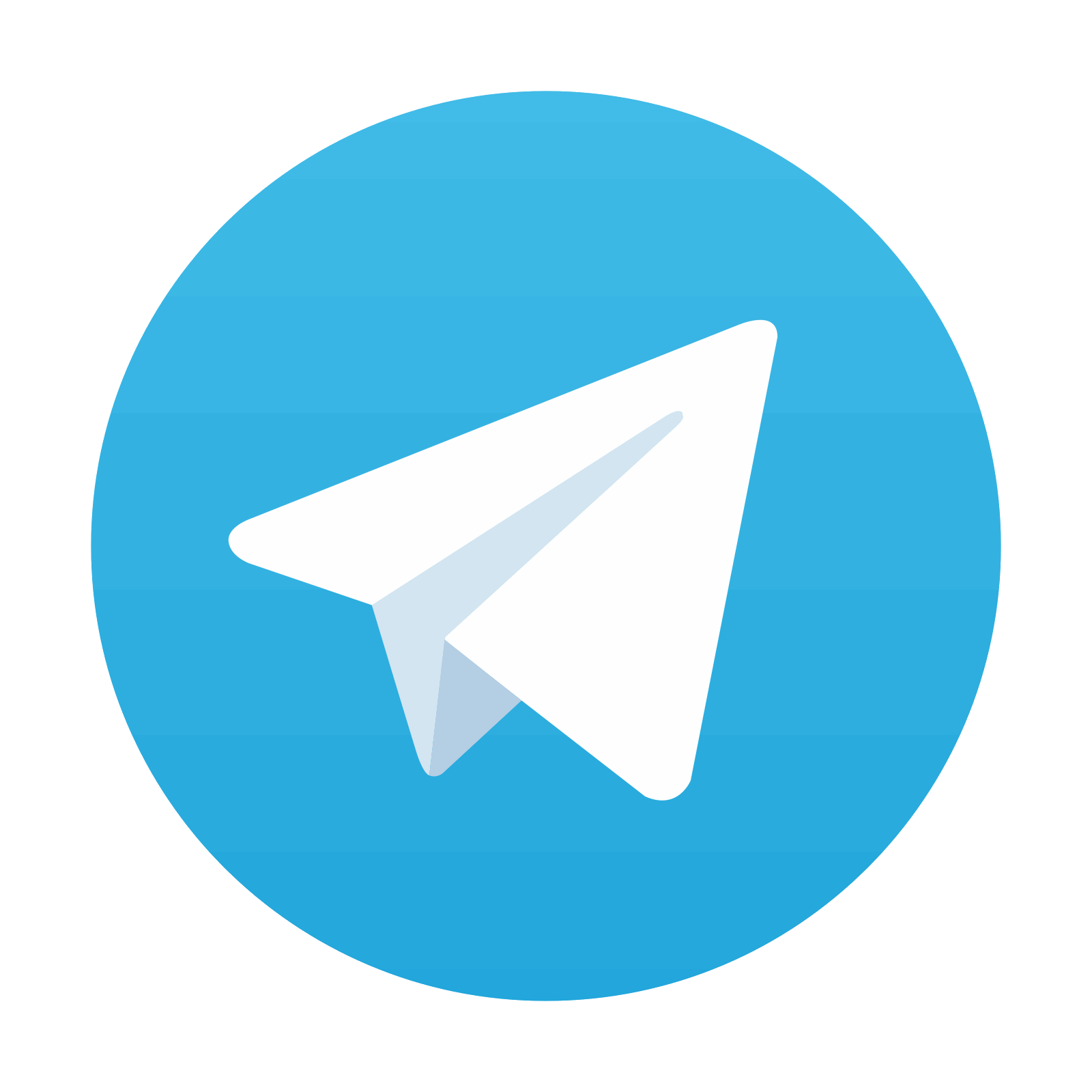
Stay updated, free articles. Join our Telegram channel

Full access? Get Clinical Tree
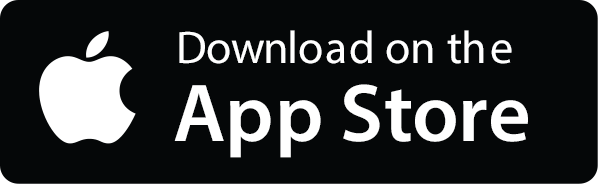
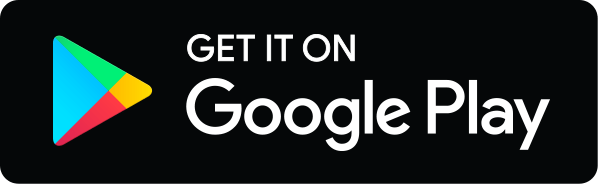