Fig. 62.1
The presenting screen of a computer decision support tool prepared for pediatric modes of ventilation. Specific tools are within the software including the oxygenation tables for various levels of PaO2 or SpO2 and PEEP, ventilation tables for various levels of pH, VT and PIP, chest film assessment for the lung injury score, nomograms for acid-base balance, predicted body weights from 0 to 19 1/2 years of age, and the application of ulna length for prediction of height and thus ideal weight in children who are either obese or have failed to thrive or have contractures
While a computer-based protocol has been developed for adult ARDS, large differences between adult and pediatric critical care bring into question the practice of extrapolating adult evidence regarding ventilator management with computer protocols to children with ALI/ARDS. In particular, pediatric practice may tolerate only smaller (more granular) changes of 0.05 in the fraction of inspired oxygen, rather than the 0.1 increments in the adult ARDSNet protocol, as suggested by our analysis of large datasets from both Vanderbilt Children’s Hospital and Children’s Hospital Los Angeles (Khemani et al. 2009a). Also, the ranges of pH used in the ARDSNet protocol for changes in ventilator rate and tidal volume may be too broad for many pediatric intensivists. There are also differences in how predicted body weight from height/length is calculated for determination of tidal volumes in lung protective volume-controlled modes, with pediatricians needing to deal with failure to thrive, contractures, and obesity, with only the latter being of real importance to adult practitioners. It is probable that measurement of ulna length will prove to be the best and safest predictor of height and the subsequent percentile for age used to calculate weight and thence tidal volume over the entire pediatric age range (Gauld et al. 2003; von Ungern-Sternberg et al. 2009). The adult protocol calls for recording the set volume delivered by the ventilator, whereas pediatric practice is to measure the tidal volumes directly, but has not been standardized to either in the ventilator or at the endotracheal tube (ETT), with large differences recorded between the two (Al-Majed et al. 2004; Rosen et al. 1993; Stenson et al. 1998). Finally, adult protocol management of ALI is based on A/C volume control, a mode rarely used by pediatric intensivists who seem to prefer pressure-regulated modes.
Decision support tools (paper or electronic) have been shown to improve the quality of medical care (Shortliffe and Cimeno 2006), reduce errors (Slonim and Pollack 2005), and improve patient outcomes (Blaser et al. 2007). However, paper-based tools can be difficult to follow accurately, leading to low adherence rates—30 % compliance in a pediatric ventilator study with a paper protocol (Randolph et al. 2002) compared with 94 % in an adult ventilator management study with a computer-based protocol (Morris et al. 1994). Computers can assist clinicians by standardizing descriptors and procedures, by consistently performing calculations, by incorporating complex rules with patient data, and by capturing data relevant to decision making (Clemmer 2004; Morris 2003; Morris et al. 1996). Computer-based protocols can contain extensive detail, while at the same time protecting the user from complexity and information overload. These tools aim to ensure flexible but consistent, evidence-based clinician decisions for equivalent patient states. Management of mechanical ventilation is an iterative treatment protocol encompassing many individual decisions that must be made over the course of a patient’s treatment, usually by multiple practitioners. Replicable ventilator management decisions would help decrease practice variability and would likely directly shorten the length of mechanical ventilation for children in pediatric ICUs (Jouvet et al. 2007). While the ARDS network has developed computer-based mechanical ventilation protocols for adults with ALI and ARDS, it is unlikely that a computer protocol for ventilator management developed in the adult ICU can simply be deployed in the pediatric setting. The protocol must be translated to ensure that it accurately reflects unique aspects of pediatric practice; and it must be evaluated in a rigorous, formal manner to ensure that it is safe, effective, and appropriately sized.
A computer-based pediatric mechanical ventilation protocol, adapted from published ARDS Network Oxygenation and Ventilation tables (ARDSNet 2000), has been created for use in pediatric trials of ALI and ARDS. The ventilation modes have been expanded from volume control to include PC, PRVC, and high-frequency oscillatory ventilation (HFOV—theoretically the most lung protective of all modes, which has never been properly tested in pediatric ALI/ARDS with an adequately powered study) (Fig. 62.2).
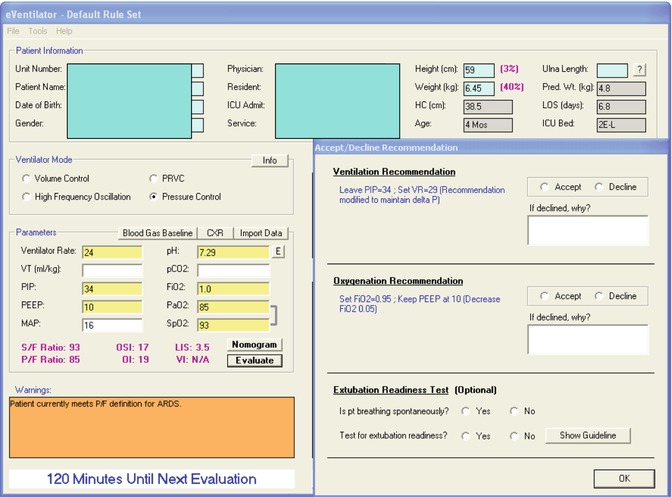
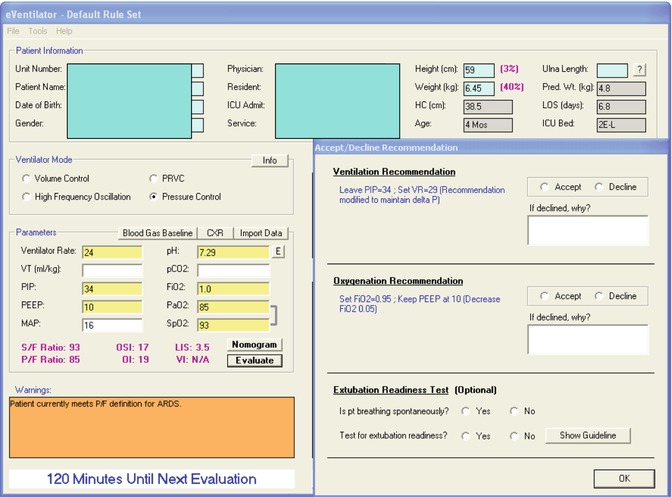
Fig. 62.2
Examples of recommendations and calculations provided to the bedside clinicians by the computer decision support for an infant with ARDS from adenovirus pneumonia, on Pressure Control mode ventilation
The tables have been refined iteratively, to reflect the consensus of a number of pediatric critical care physicians and investigators from the National Institutes of Health funded Collaborative Pediatric Critical Care Research Network and the Pediatric Acute Lung Injury and Sepsis Network. However, such protocols need to be tested to divine whether they describe current pediatric lung protective practices and evaluate whether the adapted protocol is in line with this practice. This can be done by comparing ventilator protocol recommendations to actual changes in mechanical ventilation derived from databanks of ventilation, oxygenation, and blood gas data collected over recent years while applying such lung protective strategies. Areas with high variability or in which clinical practices are not in line with the protocol will likely have the largest potential impact to change and standardize clinical practice (Khemani et al. 2010). Such areas include the time between inspired oxygen decreases appropriate for improving oxygenation, time, and magnitude of PEEP increases to limit excessive inspired oxygen increases and investigating whether it is better to target a specific tidal volume (e.g., PRVC mode) with the inherent risks of over- or underinflation as opposed to limiting pressures (e.g., PC mode) where tidal volume is allowed to vary with the degree of lung injury (Khemani et al. 2009b).
In a retrospective study (Khemani et al. 2010) of the years 2000–2207 from a single institution (Children’s Hospital Los Angeles), data were extracted for 402 children on pressure-control ventilation with PaO2/FiO2 (P/F) ratio <300. Approximately 2,700 arterial blood gas values and 6,000 ventilator settings were analyzed against this pediatric computer protocol for PC mode. Changes to PEEP, FiO2, PIP, and ventilator rate recommended by the protocol were compared to actual changes. The median P/F ratio was 140, with 24.4 % mortality. The computer protocol recommended decreasing FiO2 for 54.3 % of clinician decisions; however, FiO2 was actually decreased in only 29.3 % of these decisions. In the mid (55–68 mmHg) and low (<55 mmHg) PaO2 ranges, the computer protocol recommended more PEEP than was used in actual practice, 42 and 67 % of the time, respectively. Changes to PIP or VR after an ABG were consistent with the protocol 42 % of the time, but the most common clinical decision was no change to either PIP or VR (46 %). This was true even when pH was >7.45 and PIP was ≥30 cmH2O. Changes in ventilation were made on average every 4 h. It is not unrealistic to believe that decision support protocols utilizing noninvasive markers of oxygenation and ventilation and which provide reminders to caregivers at set time intervals can speed up this process and decrease the time of exposure to high pressures, volumes, and inspired oxygen concentrations.
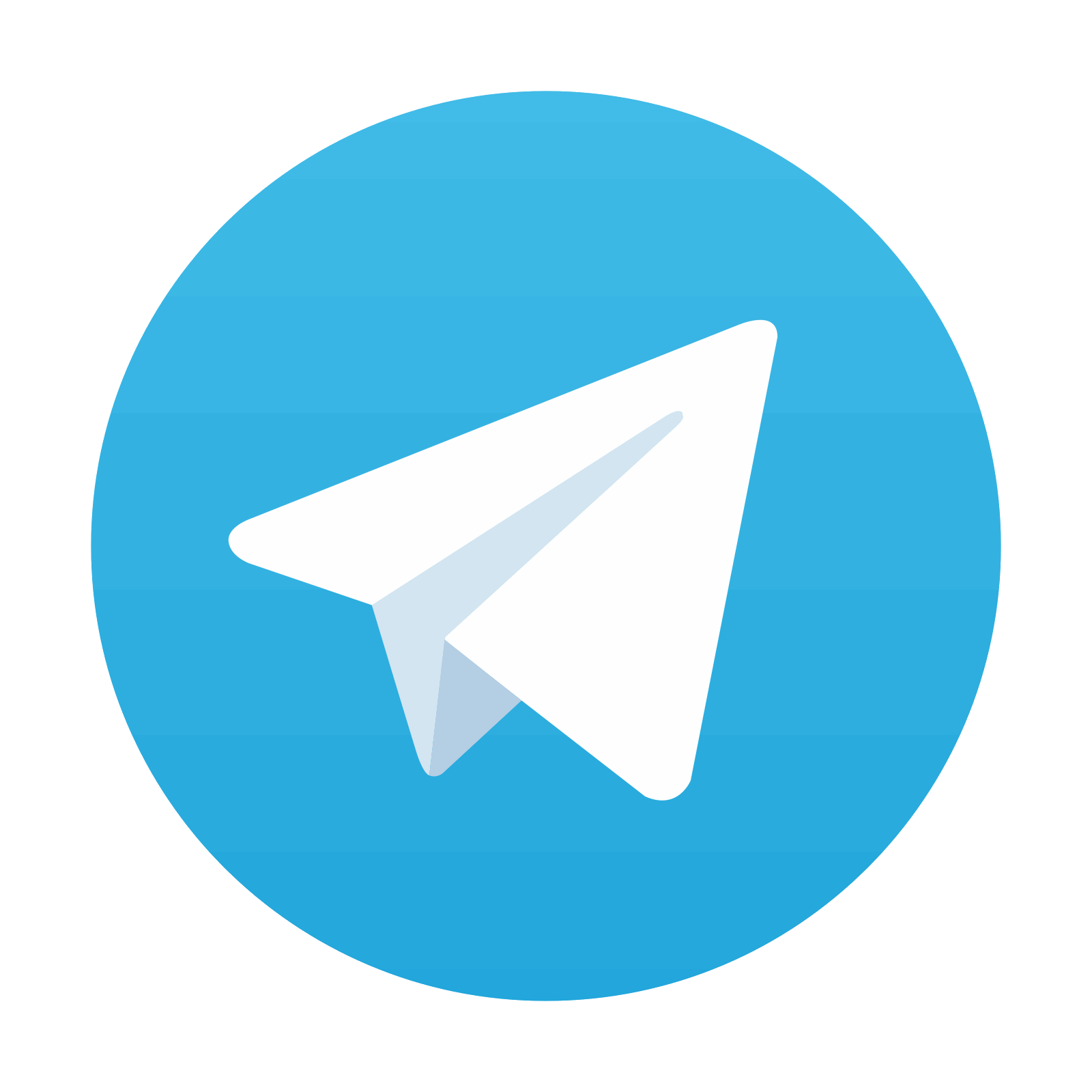
Stay updated, free articles. Join our Telegram channel

Full access? Get Clinical Tree
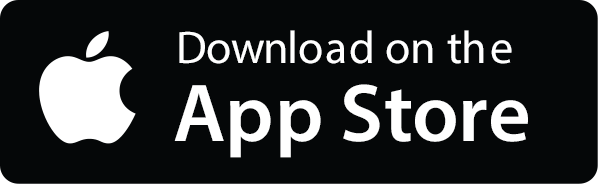
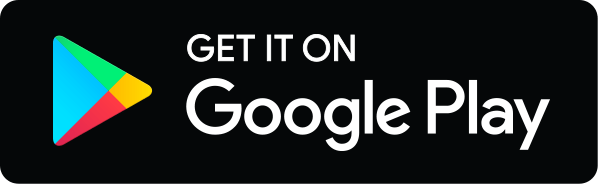