Fig. 15.1
Sagittal T1 + gadolinium and coronal T1 + gadolinium sequences of a left large parietal ATRT
Pathology
As described in Chap. 6, the consistent histologic feature of ATRT is the largely PNET-like expanses of poorly differentiated small round blue cells. Although classically described as having heterogeneous morphologies, including areas containing characteristic “rhabdoid” cells with eccentric nuclei, prominent nucleoli, and increased amounts of eosinophilic cytoplasm, it is important to recognize that ATRT frequently does not contain obvious rhabdoid cells, despite the tumor’s name. Recognition of this, as well as the fact that ATRT can occur throughout the neuroaxis, underscores the need to exclude the diagnosis of ATRT in any poorly differentiated CNS neoplasm in infants and children. In that regard, INI1 immunohistochemistry is particularly useful and readily available as a clinical test in many pathology laboratories. In nearly all cases, ATRT cells show loss of INI1 staining while normal cell constituents such as blood vessels and nonneoplastic-infiltrated brain serve as internal positive controls [19]. INI1-negative immunostaining is highly specific for ATRT and, therefore, an efficient diagnostic tool. However, Hasselblat et al. have recently reported a negative INI1 staining in cribriform neuroepithelial tumor (CRINET) [20].
Molecular Biology
The recognition of ATRT as a distinct pathological entity was initially supported by the recurrent association with chromosome 22 abnormalities involving monosomy or deletion/translocation involving the chromosome band 22q11.2 detected by conventional karyotype or fluorescent hybridization in situ (FISH) studies for submicroscopic deletion [21]. Subsequent positional cloning studies of this region lead to the identification of the tumor suppressor gene INI1/hSNF5/BAF47/SMARCB1; one of >10 members of the highly conserved ATP-dependent SWI/SNF chromatin-remodeling complex is required for transcriptional activation of many genes with fundamental roles in cell growth, differentiation, DNA repair, and other cellular processes [22]. Mutations of a gene involved in chromatin remodeling raises the possibility of epigenetic dysregulation as a major mechanism for oncogenesis [23]. However, the function of SMARCB1 and the mechanisms by which its loss leads to the development of rhabdoid tumors remain poorly understood. SMARCB1 is a demonstrated modulator of the cell cycle through downregulation of p16 and increased expression of cyclin D1 leading to a dysregulated CDHK4/Rb/E2F signaling axis [24]. Reintroduction of SMARCB1 into SMARCB1-deficient cell lines leads to cytoskeletal changes and ultimately apoptosis or cellular senescence and accumulation in the G0/G1 phase. The mechanism is believed to involve the loss of EZH2 inhibition by the SMARCB1-deficient SWI/SNF complex leading to derepression of Polycomb targets and aberrant activation of stem cell programs [25]. c-Myc is also dysregulated in ATRT through direct binding of SMARCB1. Loss of SMARCB1 is thought to disrupt c-myc-mediated repression of cyclin D1, further compounding loss of cell cycle control. Such studies have spawned interest in EHZ2 inhibitors as a potential therapeutic avenue. Other researchers have suggested that the loss of SMARCB1 results in chromosomal instability and may also be involved in the DNA damage response [26].
Biallelic alteration of SMARCB1 can be found in up to 94 % of ATRTs; however, a minority of cases has reported homozygous mutations involving another SWI/SNF chromatin-remodeling complex member—the ATPase subunit SMARCA4 (BRG1)—suggesting the usefulness of SMARCA4 antibodies in the diagnosis of the rare BAF47-positive ATRT [27].
Other than alterations in SMARCB1, the ATRT genome is highly stable and largely devoid of other recurrent mutations in other loci. Kieran et al. described the absence of canonical pathway mutations in rhabdoid tumor using a platform of 983 mutations encompassing 115 genes [28]. High-resolution genotyping array studies failed to identify copy number changes outside of chromosome 22. Similarly, a recent exome sequencing study conducted in 2012 by Lee et al. reported an overall somatic mutation rate of only 0.19 mutations/Mb, well below the rates observed for other known pediatric cancers [23]. Global gene expression studies have revealed distinct sub-clusters of ATRT. Birks et al. identified a sub-cluster of ATRT with a high expression of the BMP pathway genes (i.e., BMP4, SOST, BAMBI, MSX2), which correlated with a worse prognosis [29]. An independent study of ten rhabdoid tumors also demonstrated an enrichment of BMP signaling pathway genes suggesting an aberrant differentiation program and disruption of BMP signaling. This suggests the discovery of subgroup-specific treatment strategies will be important for future clinical investigation.
Germ-line mutations of SMARCB1 have been reported in up to 20–35 % in ATRT, but the true incidence of constitutional SMARCB1 mutations is not known [30, 31]. Patients with germ-line mutations are predisposed to rhabdoid tumor of the brain, kidney, and soft tissues and may present with multiple synchronous tumors. These children tend to be less than 1 year of age at the time of diagnosis and carry a worse prognosis [31]. In the vast majority of the cases, these SMARCB1 germ-line mutations are sporadic, but familial cases of ATRT have been reported [27]. Germ-line mutation of SMARCA4 has also been implicated in familial form of ATRT [32]. The pedigree analysis of this affected family supports an autosomal dominant inheritance with incomplete penetrance. The identification of asymptomatic carrier parents in this reported family highlights the need for systematic testing of all newly diagnosed ATRT patients for germ-line mutations [15].
Therapeutic Management
ATRTs are typically associated with a grim prognosis with estimated median survival times ranging from 8 to 16 months [2, 8, 9, 13, 14]. Because of the rarity of these tumors and a history of having been treated nonspecifically with other malignant tumors, there is no current standard of care for these tumors.
While in the early years of recognition of the entity, a significant number of patients only received palliative treatment and rapidly succumbed to their disease, in the most recent era, more aggressive and multimodality approaches have emerged and may be associated with a trend toward improved outcome [11]. These multimodality strategies variously include surgery, conventional chemotherapy, high-dose chemotherapy with hematopoietic stem cell support, intrathecal chemotherapy, and radiotherapy. The specific role of each individual modality, however, has not yet been clearly defined.
Surgery
There is strong evidence to show that complete tumor resection is associated with a more favorable outcome [6, 9, 33]. In the series reported by Chi et al., patients who underwent gross total resection (GTR) of their tumors had a 2-year OS of 91 ± 9 %, while those who underwent less than a GTR had a median OS of 18 months [11]. Similarly, in the Canadian series, patients for whom GTR was achieved had a 2-year OS of 60 ± 12.6 % compared to 21.7 ± 8.5 % for those whose tumors were less than completely resected. The invasive and vascular nature of these tumors can limit the possibility of initial GTR, and the rates of GTR reported in various series range from 30 to 68 % [7, 8, 11, 13]. With the goal of potentially maximizing the benefit of surgery, treatment on the recently closed Children’s Oncology Group (COG) clinical trial for ATRT, the first national cooperative group trial exclusively for patients with ATRT, included a recommendation for a second operation after two courses of chemotherapy for those cases where GTR of disease was not possible at diagnosis.
Conventional Chemotherapy
ATRT responds to chemotherapy, but the isolated use of conventional-dose chemotherapy alone, as used in the “baby-brain” strategies of the 1990s, failed to cure most patients. In the second-generation Pediatric Oncology Group (POG) “baby-brain” protocol 9233/9234, complete response to chemotherapy was achieved in 21 % of the evaluable patients, but the median survival time was only 6.7 months and none of the 36 patients survived (Douglas Strother personal communication). Using similar chemotherapy, the Children’s Cancer Group (CCG) 9921 protocol achieved a 29 % 5-year survival for its ATRT patients [34]. Based on several case reports of successful treatment using conventional chemotherapy according to the Intergroup Rhabdomyosarcoma Study protocol III (IRS-III) for parameningeal rhabdomyosarcoma [35], the group from Dana-Farber developed a specific protocol for ATRT using a modified IRS-III regimen which utilized doxorubicin and dactinomycin that are not typically used in other pediatric brain tumor protocols. It also included intrathecal chemotherapy and radiation; therefore, the distinct role of these particular drugs is not known. Nonetheless, use of this approach has been associated with a 58 % objective response rate prior to radiation [11].
High-Dose Chemotherapy
In the most recent decade, high-dose chemotherapy and hematopoietic stem cell rescue (HSCR) have been reported in relatively small cohorts of patients [33, 36, 37]. The most common regimen used single cycle of high-dose carboplatin, thiotepa, and etoposide or 3 cycles of high-dose carboplatin and thiotepa. Ginn et al. compiled 33 cases published of patients treated with high-dose chemotherapy strategy. Fourteen of them also received radiation therapy at some point in their treatment. Overall, 20 patients were reported alive at a median follow-up time of 50 months (5–105) [38]. In the Canadian registry, 9 of 18 patients treated with high-dose chemotherapy were alive at a median follow-up time of 41 months. High-dose chemotherapy was associated with a significant survival benefit with a 2-year OS of 47.9 ± 12.1 % versus 27.3 ± 9.5 % for the conventional chemotherapy group (p = 0.036) [8].
Although definitive conclusions cannot be made from these series because patient numbers are very low, studies are not randomized, and the use of therapy before and after high-dose consolidation is inconsistent, the results suggest that consolidation with high-dose chemotherapy regimens to these patients is feasible and may provide encouraging survival figures.
Intrathecal Chemotherapy
Because of the young age of children with ATRT, the use of intrathecal chemotherapy for CNS prophylaxis, instead of craniospinal irradiation, has been explored. Methotrexate, with or without cytosine arabinoside, hydrocortisone, or both, has preferentially been used. In the US ATRT registry, 16 patients (38 %) received intrathecal chemotherapy as part of the primary therapy. The repeated use of IT methotrexate via Ommaya reservoir is a key component of the German HIT SKK protocol for infant brain tumors, including ATRT [39]. Similarly the Dana-Farber Cancer Institute ATRT protocol also included iterative injections of IT chemotherapy. The use of IT is one component of these multimodality approaches; the small numbers of patients limit our ability to assess its specific role for disease control. In one meta-analysis of published ATRT cases, Athale et al. reported a benefit in 2-year overall survival for patients treated with IT chemotherapy in combination with RT, compared to patients treated with RT alone or neither RT nor IT chemotherapy [14].
Radiation Therapy
As is the case with all other malignant brain tumors in very young children, the use of RT in ATRT raises serious concerns related to its significant long-term toxicity, primarily in terms of neurocognitive impairment. In the absence of a randomized study specifically addressing the role of radiotherapy, incorporation of radiation into most current strategies has been based on observations from retrospective series that suggest a survival benefit in children treated with some form of radiation. In the registry reported by Hilden, 13 patients (31 %) received radiation. Eight of the 13 survivors received primary radiation with a median survival of 48 months. The median age of irradiated patients was older than that of the entire registry cohort (47 months versus 24 months, respectively) [14]. In the recently updated experience from the St. Jude Children’s Research Hospital, 19 children under 3 years of age received focal (n = 13) or craniospinal (n = 6) radiation, and six were alive without disease at the time of the report. All six survivors received focal radiation only after local progression of disease on chemotherapy. The 4-year PFS and OS rates for the entire cohort were 33.2 % ± 10 % and 53.5 % ± 10 %, respectively. Although treatment regimens were not uniform, nearly all patients younger than 3 years were treated with neoadjuvant, pre-irradiation chemotherapy. With these caveats, the investigators found that delay of radiation was associated with an increased risk of local and distant disease progression [12]. In a cohort of patients with a median age of 4.5 years, Chen et al. also reported increased time between diagnosis and radiation therapy to adversely affect outcome [40].
In contrast, and with many similar limitations of retrospective series, including multiple regimens of chemotherapy and various combinations of treatment modalities used, French investigators found no significant survival benefit for the patients who received adjuvant radiotherapy (16/58). While in their cohort, children younger than 2 years fared significantly worse than older children, the sub-analysis between adjuvant radiation and no radiation failed to show statistical significance (p = 0.06). They hypothesized that the underlying molecular biology of ATRT may be different in infants compared to older children. Germ-line mutation of INI1 seen with higher frequency in younger patients is associated with an earlier onset and more aggressive phenotype and may partially explain the poorer prognosis of the younger age group [13, 41]. Analyses from the Canadian registry showed that cure without radiation was possible. Among 16 patients treated with high-dose chemotherapy and no radiation, 9 (56 %) were long-term survivors at a median follow-up of 64 months [42]. If RT is used to treat ATRT, German data suggest that there is no survival difference between patients treated with radiation at diagnosis and those treated at the time of relapse (3-year OS 43 ± 14 % versus 31 ± 13 %, respectively, (p = 0.314)) [39].
Thus, the benefit of RT for patients with ATRT is still a matter of debate [3, 38]. Optimal doses and volumes of radiation have not been standardized. Nevertheless, radiation has been included in recent therapeutic strategies for ATRT. Given the very young age of the vast majority of these patients and the predominance of relapse at the primary site, priority has been given to focal radiation. Patient with localized disease are treated with focal RT while craniospinal irradiation is recommended for disseminated disease with doses varying with age [11, 43].
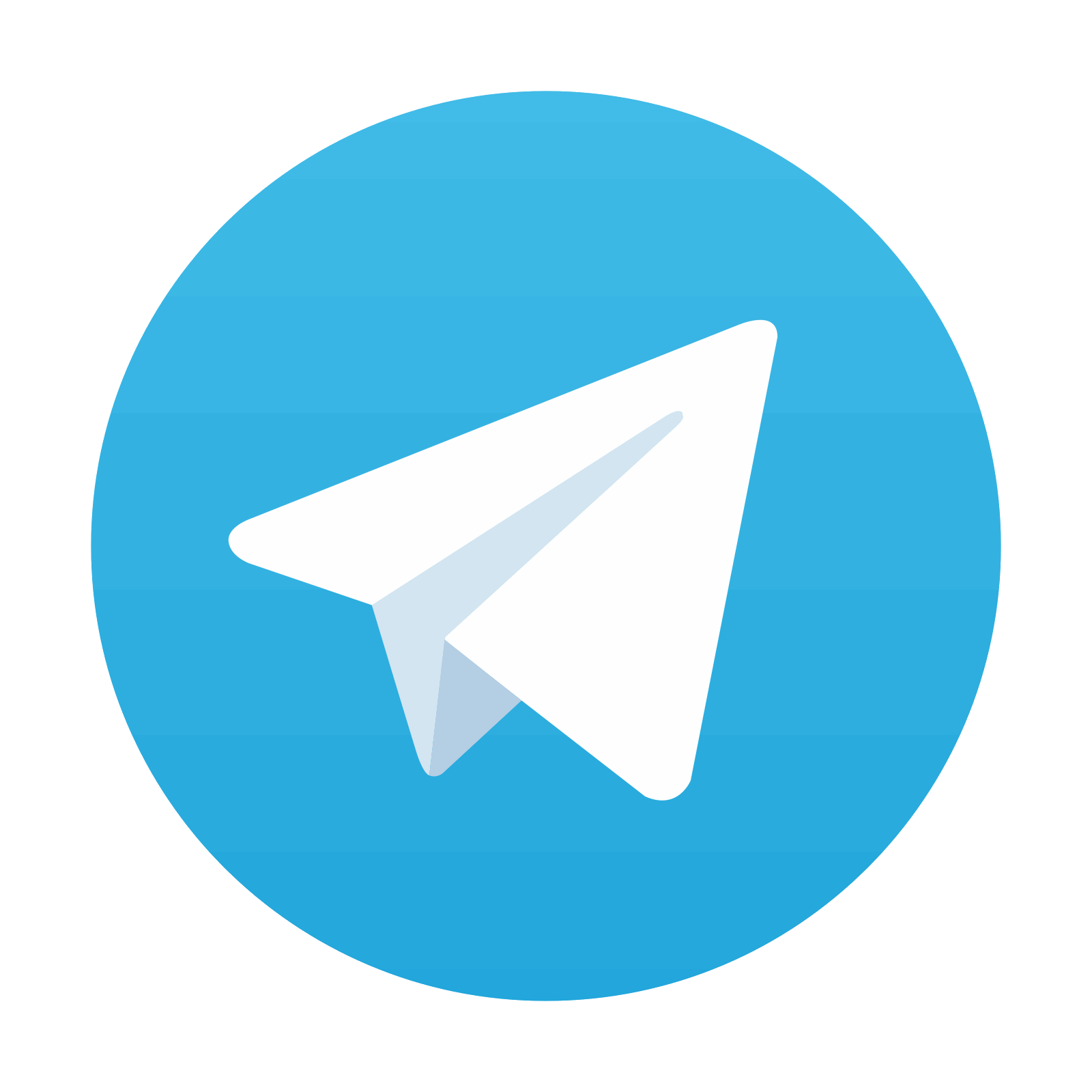
Stay updated, free articles. Join our Telegram channel

Full access? Get Clinical Tree
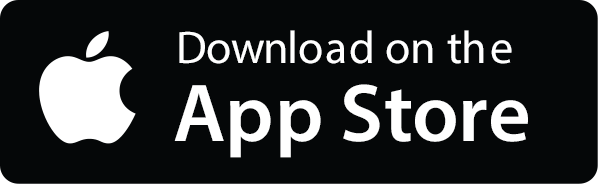
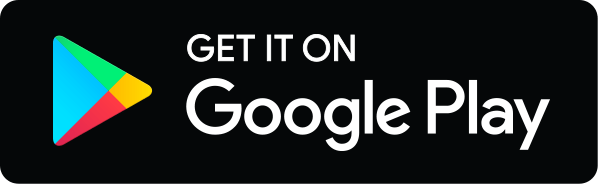