Data regarding US outpatient pediatric antimicrobial prescriptions show a decreased number of antimicrobial prescriptions in 2010 compared to 2002. The most frequently prescribed antimicrobials are amoxicillin, azithromycin, amoxicillin-clavulanate, and cefdinir. However, neither azithromycin nor cefdinir is recommended as first line agent for the most common pediatric conditions, suggesting that these agents may be overprescribed.
Cultures should be obtained in potentially serious infections. Empiric antimicrobial therapy may be initiated and then modified according to the patient’s response and the culture results. Often several equally safe and efficacious antimicrobials are available. In this circumstance, the relative cost and ease of administration of these choices should be considered.
Other important considerations include the patient’s age, immune status, and exposure history. Neonates and young infants may present with nonspecific signs of infection, making the differentiation of serious disease from mild illness difficult. In older children, clinical diagnosis is more precise, which may permit therapy to be avoided or allow use of a narrower-spectrum antibiotic. Immune deficiency may increase the number and types of potential infecting organisms that need to be considered, including organisms that are usually avirulent, but in the altered host may cause serious infections that are difficult to treat. An abnormal immune response may also diminish the severity of the clinical signs and symptoms of infection, leading to underestimation of the severity of illness. The exposure history may suggest the greater likelihood of certain types of infecting organisms. This history includes exposures from family members, classmates, and day care environments, and exposure to unusual organisms by virtue of travel, diet, or contact with animals.
Final important considerations are the pace and seriousness of the illness. A rapidly progressive and severe illness should be treated initially with broad-spectrum antimicrobials until a specific etiologic diagnosis is made. A mildly ill outpatient should receive treatment preferentially with narrow-spectrum antimicrobials.
Antimicrobial susceptibility, antimicrobial families, and dosing recommendations are listed in Tables 39–2 to 39–6.
Table 39–2. Susceptibility of some common pathogenic microorganisms to various antimicrobial drugs.
Table 39–3. Groups of common antibacterial agents.
Table 39–4. Guidelines for use of common parenteral antibacterial agentsa in children age 1 month or older.
Table 39–5. Guidelines for use of common oral antibacterial agents in children age 1 month or older.
Table 39–6. Guidelines for use of selected antimicrobial agents in newborns.
ANTIMICROBIAL SUSCEPTIBILITY TESTING
Cultures and other diagnostic material must be obtained prior to initiating antimicrobial therapy—especially when the patient has a serious infection, initial attempts at therapy have failed, a patient has an unsual exposure, or multiagent therapy is anticipated. Whenever cultures identify the causative organism, therapy can be narrowed or optimized according to susceptibility results. Antimicrobial susceptibility testing should be done in a laboratory using carefully defined procedures (as defined by the Clinical and Laboratory Standards Institute).
There are several ways to test antimicrobial susceptibility. Identification of an antibiotic-destroying enzyme (eg, β-lactamase) implies resistance to β-lactam–containing antimicrobial agents. Tube or microtiter broth dilution techniques can be used to determine the minimum inhibitory concentration (MIC) of an antibiotic, which is the amount of antibiotic (in mcg/mL) necessary to inhibit the organism under specific laboratory conditions. Disk susceptibility testing (also performed under carefully controlled conditions) yields similar results. The E-test is a standardized test for some organisms that correlates well with MICs. Clinical laboratories usually define antimicrobial susceptibility (susceptible, intermediate, and resistant) in relation to levels of the test antibiotic achievable in the blood or another body fluid (cerebrospinal fluid [CSF] or urine).
Organisms are usually considered susceptible to an antibiotic if the MIC of the antibiotic for the organism is significantly lower than levels of that agent achieved in the blood using appropriate parenteral dosages. This assumption of susceptibility should be reconsidered whenever the patient has a focus of infection (eg, meningitis, osteomyelitis, or abscess) in which poor antibiotic penetration might occur, because the levels of antibiotic in such areas might be lower than the MIC. Conversely, although certain organisms may be reported to be resistant to an antibiotic because sufficiently high blood concentrations cannot be achieved, urine concentrations may be much higher. If so, a UTI might respond to an antibiotic that would not be adequate for treatment of septicemia.
Thus, antimicrobial susceptibility testing, although a very important part of therapeutic decision making, reflects assumptions that the clinician must understand, especially for serious infections. Ultimately the true test of the efficacy of therapy is patient response. Patients who do not respond to seemingly appropriate therapy may require reassessment, including reconsidering the diagnosis, reculturing, and repeat susceptibility testing, to determine whether resistant strains have evolved or superinfection with a resistant organism is present. Antimicrobial therapy cannot be expected to cure some infections unless additional supportive treatment (usually surgical) is undertaken.
ALTERATION OF DOSE & MEASUREMENT OF BLOOD LEVELS
Certain antimicrobial agents have not been approved (and often not tested) for use in newborns. For those that have been approved, it is important to recognize that both dose and frequency of administration may need to be altered (see Tables 39–4 and 39–5), especially in young (7 days or younger) or low-birth-weight neonates (≤ 2000 g).
Antimicrobial agents are excreted or metabolized through various physiologic mechanisms (eg, renal, hepatic). It is important to consider these routes of excretion and alter the antimicrobial dosage appropriately in any patient with some degree of organ dysfunction. (See Chapter 24.) As indicated in Table 39–4, an assessment of renal or hepatic function may routinely be necessary for patients receiving certain drugs (eg, renal function for aminoglycosides, hepatic function for erythromycin or clindamycin); otherwise, harmful drug levels may accumulate. In some circumstances a patient may be receiving multiple drugs where the drug interactions are unknown. If significant organ dysfunction is present, drug clearance may be delayed and dosage modification may be necessary (see detailed descriptions in individual drug information packets), and measurement of drug levels may be indicated.
Serum levels of drugs posing a high risk of toxicity (eg, aminoglycosides) are ordinarily measured. Measurement of other drugs (eg, vancomycin) may be useful in selected circumstances (see the following discussion). For certain serious bacterial infections (eg, bacterial endocarditis), measurement of the serum concentration of an antimicrobial may be important to determine optimal therapy.
Certain drug interactions may require modification of drug dosage or other therapeutic alterations. For example, rifampin stimulates the metabolism of warfarin, progesterone, prednisone, and anticonvulsants by stimulating the cytochrome P450 (CYP450) metabolic pathway. Dosage adjustments or alternative medications may be necessary to avoid significant adverse events. Another common example is erythromycin, which may inhibit the metabolism of theophylline, resulting in toxic theophylline levels. Although many drug interactions are known and well documented, it may be difficult to predict interactions that result from a combination of four, five, or more different medications. A high level of suspicion regarding adverse clinical events should be maintained.
THE USE OF NEW ANTIMICROBIAL AGENTS
New antibiotics are introduced frequently, often with claims about unique features that distinguish these usually more expensive products from existing compounds. Often these drugs share many properties with existing drugs. The role that any new antimicrobial will play can only be determined over time, during which new or previously unrecognized side effects might be described and the clinical efficacy established. Clinical trials may not be confirmed in the larger number of patients subsequently treated in practice. Because this may take many years, a conservative approach to using new antibiotics seems fitting, especially because their costs are often higher, and appropriate antimicrobial choices for most common infections already exist. It is appropriate to ask if a new antimicrobial has been proved to be as effective as (or more effective than) the current drug of choice, and whether its side effects are comparable (or less common) and its cost reasonable. The withdrawal of moxalactam and trovafloxacin due to unexpected serious side effects, which were not anticipated despite extensive premarket testing, highlight the caution necessary before using new antimicrobials. The heavy marketing of new cephalosporins and fluoroquinolones, which are very similar to existing drugs, is typical of the difficulty in evaluating antimicrobials.
The development of new antibiotics is important as a response to the emergence of resistant organisms and for treatment of infections that are clinically difficult to treat (eg, viruses, fungi, and some resistant bacteria). Fortunately, these infections are either rare or (usually) self-limited in immunocompetent hosts.
PROPHYLACTIC ANTIMICROBIAL AGENTS
Antimicrobials can be used to decrease the incidence of postoperative infections (Table 39–7). A dose of an antimicrobial given 30 minutes to 2 hours prior to surgery can reduce postoperative wound infection. The goal is to achieve high levels in the serum at the time of incision and by this means—along with good surgical technique—to minimize viable bacterial contamination of the wound. During a lengthy procedure, a second dose may be given. No evidence exists that multiple subsequent doses of antimicrobials confer additional benefit. The antimicrobial(s) used for prophylaxis are directed toward the flora that most commonly cause postoperative infection at a given anatomic site. Gram-positive cocci, such as S aureus that colonize the skin, are usually targeted, and a first-generation cephalosporin (eg, cefazolin) is a cost-effective choice. Third-generation cephalosporins and other broad-spectrum agents are more expensive and offer less benefit because they are less active than cefazolin against S aureus. Cefoxitin and cefotetan, which have activity against anaerobic bacteria, are useful for procedures, such as colorectal surgery, although cefazolin has been effective for most gynecologic patients. In colorectal surgery, orally administered antimicrobials such as neomycin and erythromycin may be as effective as parenteral antimicrobials.
Table 39–7. Dosing aminoglycosides in newborns.
In hospitals where the predominant S aureus strains are methicillin resistant or in cases where the patient is allergic to penicillin and cephalosporins, vancomycin can be considered. However, prophylactic vancomycin has caused hypotension at the time of induction of general anesthesia. Frequent use of vancomycin as a prophylactic antimicrobial will contribute to the development of vancomycin-resistant strains such as Enterococcus faecalis. For these reasons, vancomycin should generally not be used for prophylaxis, although it might prove useful for individual patients at extremely high risk.
Endocarditis prophylaxis is recommended for certain dental procedures in high-risk cardiac patients. Patients with prosthetic cardiac values or prosthetic cardiac material during the 6 months following the procedure, unrepaired cyanotic heart disease, children with prior endocarditis, and children with other prosthetic patches, which inhibit endothelialization, should receive prophylaxis. Dental procedures that would trigger prophylaxis are those that involve manipulation of gingival tissue, periapical tissues. Patients with indwelling venous catheters undergoing similar extensive dental procedures should receive prophylaxis. Prophylaxis is not recommended for routine cleanings or orthodontic applications. Prophylaxis is not recommended for other gastrointestinal or genitourinary procedures.
Prophylaxis against infection with group A streptococci reduces the recurrence rate for acute rheumatic fever. Postexposure prophylaxis is given after exposure to pertussis, Haemophilus influenzae type b (Hib) infection (depending on age), meningococcus, gonococcus, tuberculosis (household exposure), plague, aerosolized tularemia or anthrax, and other high-risk infections. Family or close contacts of patients with severe invasive streptococcal disease may benefit from prophylaxis. Silver nitrate, erythromycin, povidone-iodine, and tetracycline are used in ophthalmic preparations for prevention of gonococcal ophthalmia neonatorum. Children with asplenia or sickle cell disease receive prophylactic penicillin to protect against overwhelming S pneumoniae sepsis, usually started immediately with the onset of fever.
Prophylactic antimicrobials are sometimes used for some children at high risk for recurrent UTI, but the rate of infection has not been proven to decrease.
Chemoprophylaxis of children at high risk for influenza but not immunized against influenza should be considered, including some immunocompromised children and unimmunized caregivers of at-risk children and children at high risk for 2 weeks after immunization. Oseltamivir or Zanamivir would be used as each is usually active against influenza A and B.
INITIAL EMPIRIC ANTIMICROBIAL CHOICES FOR SELECTED CONDITIONS
General recommendations for specific conditions are as follows. A specific selection depends on the patient’s age, diagnosis, site of infection, severity of illness, antimicrobial susceptibility of bacterial isolates, and history of drug allergy. Always consult the package insert for detailed prescribing information. Tables 39–2 to 39–6 include further information.
Neonatal Sepsis & Meningitis
The newborn with sepsis may have signs of focal infection, such as pneumonia or respiratory distress syndrome, or may have subtle nonfocal signs. Group B streptococci, Escherichia coli, and other gram-negative rods are commonly encountered. Listeria monocytogenes is now uncommon in many nurseries, but a recent nationwide outbreak demonstrates the pathogenic potential of this organism. Ampicillin and gentamicin (or another aminoglycoside) are preferred. If meningitis is present, many clinicians substitute a third-generation cephalosporin for the aminoglycoside. S pneumoniae is an uncommon cause of meningitis in neonates. However, if the Gram stain shows gram-positive cocci suggesting S pneumoniae, substitution of vancomycin for ampicillin should be considered. In the newborn with cellulitis, S aureus including methicillin-resistant S aureus (MRSA) and group A streptococci are additional considerations. Nafcillin, oxacillin, or a first-generation cephalosporin is usually added. Vancomycin is added if MRSA is common in the geographic area. Omphalitis is often polymicrobial, and Enterococcus species, gram-negative aerobes, and anaerobes may be causative. Clindamycin, ampicillin, and an aminoglycoside or cefotaxime cover the most likely organisms; early surgical intervention is indicated.
Sepsis in an Infant
S pneumoniae and Neisseria meningitidis are most commonly encountered in infants. Hib infection may occur in unimmunized children. A third-generation cephalosporin is appropriate. Intermediate-level penicillin and cephalosporin resistance in S pneumoniae usually do not cause therapy to fail unless meningitis or another difficult-to-treat infection, such as endocarditis or osteomyelitis, is present.
Occult bacteremia may be encountered in young infants with high fever. Prior to immunization with vaccines effective against Hib, persistent bacteremia and complications including meningitis were seen in approximately 50% of children infected with occult Hib bacteremia. With widespread use of Hib vaccine, Hib is a very uncommon cause of occult bacteremia in young children with fever. Similarly, prior to the introduction of the conjugate pneumococcal vaccine, S pneumonia bacteremia was demonstrated in 3%–5% of infants aged 3–36 months with fever of 39°C or greater, no identified source for fever on examination, and white blood cell count (WBC) of 15,000/mm3. The risk of bacteremia was 6%–10% in younger children with fever greater than 40°C and WBC of 20,000/mm3 or higher. The risk of developing meningitis due to persistent S pneumoniae is estimated at 3% of those who are known to be bacteremic. The risk of S pneumoniae bacteremia and its complications is significantly reduced in children immunized with the conjugate pneumococcal vaccines. In clinical trials and subsequent national surveillance for the incidence of disease, the 7-valent pneumococcal vaccine reduced the incidence of invasive pneumococcal diseases by approximately 90%. Studies are underway with the newer 13 valent pneumococcal vaccines. Observation without antimicrobials, but with appropriate plans for follow-up examinations, is appropriate for most febrile young children who are fully immunized.
Nosocomial Sepsis
Many bacterial pathogens can cause infection in hospitalized patients. Recent local experience is usually the best guide to etiologic diagnosis. For example, some intensive care units experience frequent infections due to Enterobacter cloacae, whereas in other units Klebsiella pneumoniae is the most common nosocomial isolate. Initial therapy should include antibiotics effective for MRSA and resistant Pseudomonas aeruginosa if these are frequent isolates. E faecalis is a common cause of nosocomial bacteremia in patients with central catheters, particularly in units where cephalosporins are heavily used. Coagulase-negative staphylococci are commonly isolated from patients with indwelling central catheters. In seriously ill patients, when the local experience suggests that Enterococcus species or coagulase-negative staphylococci are common, the initial regimen should include vancomycin. Because Enterococcus species and coagulase-negative staphylococci commonly cause fever without significant morbidity or mortality, initial regimens without vancomycin are appropriate, with adjustment of treatment after susceptibility is known. If P aeruginosa or other resistant gram-negative rods are common, ceftazidime or cefepime should be included in initial therapy. Candida is a common isolate from blood cultures from immunocompromised or seriously ill patients in the intensive care unit. Empiric antifungal therapy is initiated in some high-risk patients.
Meningitis
Bacterial meningitis in neonates is usually caused by infection with group B streptococci, E coli, other gram-negative rods, or L monocytogenes. A combination of ampicillin and a third-generation cephalosporin is started initially. In an infant or older child, S pneumoniae or N meningitidis are the most common isolates. Hib is uncommon now because of widespread immunization. Increasingly, S pneumoniae with resistance to a third-generation cephalosporin (MIC > 2 mcg/mL) may occur in 3%–5% of isolates.
In bacterial meningitis, peak CSF antimicrobial concentrations 10 or more times greater than the MIC of the organism are desirable, but this may be difficult to achieve if organisms are resistant. The therapeutic problem is complicated if dexamethasone, which reduces the entry of some antimicrobials into the CSF, is also given. Thus, initial therapy of bacterial meningitis in an older child should include vancomycin and a third-generation cephalosporin. Alternatively, meropenem has also been successful. A lumbar puncture should be considered 24–48 hours after the start of therapy to assess the sterility of the CSF. Rifampin should be added if the Gram stain or cultures of CSF are positive on repeated lumbar puncture, if the child fails to improve, or if an organism with a very high MIC to ceftriaxone is isolated. The optimal therapy of highly resistant S pneumoniae meningitis is not well established.
Meningitis in a child with a ventriculoperitoneal shunt is most commonly caused by coagulase-negative staphylococci, many of which are methicillin-resistant, or Corynebacterium species, which are resistant to many antimicrobials. In many of these patients who are not seriously ill, therapy should be postponed while awaiting the appropriate shunt fluid for Gram stain and culture. Seriously ill patients should initially be given vancomycin and a third-generation cephalosporin, because S aureus and gram-negative rods are also possible and can cause serious infection.
Urinary Tract Infection
E coli is the most common isolate from the urinary tract. Outpatients with symptoms of lower urinary tract disease or with mild illness can be given ampicillin, cephalexin, or trimethoprim-sulfamethoxazole (TMP-SMX). Local experience and resistance rates should guide initial therapy. In selected patients with pyelonephritis, outpatient therapy is effective using parenteral aminoglycosides or ceftriaxone once per day. Oral cefixime and amoxicillin-clavulanate can be used in place of ceftriaxone for outpatient therapy. Ciprofloxacin has been approved by the US Food and Drug Administration for therapy of urinary tract infection (UTI) in children older than 1 year, but it should be reserved for complicated cases. For hospitalized patients with UTI and suspected bacteremia, ampicillin and gentamicin or a third-generation cephalosporin is appropriate. Gram stain should be used to guide the initial choice. For patients with known or suspected resistant organisms, such as P aeruginosa, or for patients with urosepsis, an aminoglycoside and ceftazidime, cefepime, or ticarcillin may be started. Unit-specific data on typical bacterial species and their patterns of susceptibility should guide the antimicrobial choice for nosocomial UTIs.
Bacterial Pneumonia
Bacterial pneumonia in newborns generally should be treated with the same antimicrobial choices as sepsis. Infants and older children are frequently infected with S pneumoniae. Ampicillin and amoxicillin are effective in most patients eligible for outpatient therapy.
Previously healthy children admitted to the hospital with uncomplicated pneumonia can be treated successfully with amoxicillin, ampicillin, or penicillin.
Children with inadequate immunization, children with high-level pneumococcal resistance (penicillin MIC ≥ 4.0 mg/mL), and children who are critically ill may benefit from a third-generation cephalosporin.
Children with culture-positive paraneumonic effusions should be treated based on the culture and susceptibility report. Children with small or culture-negative parapneumonic effusions should be treated based on the severity of illness.
A rapidly progressive pneumonia, with pneumatoceles or large empyema, may be due to MRSA or group A streptococci, Hib, or another gram-negative rod. Vancomycin should be used in addition to a third-generation cephalosporin in these instances.
Children aged 6 years and older more frequently have infection with Mycoplasma pneumoniae or Chlamydia pneumoniae. Azithromycin is usually preferred for initial empiric therapy.
Skin & Soft Tissue Infections
S aureus and Streptococcus pyogenes are the most common causes of skin and soft tissue infections (SSTIs) in children. Community-acquired MRSA infections are common in many communities and complicate clinical decision making. Culture and susceptibility testing of abscesses, cellulitis, and more serious SSTIs is very important for optimal clinical management. Children with cellulitis more commonly have infection with group A streptococci, and empiric outpatient therapy with cephalexin or dicloxacillin is preferred. Children with small (< 5 cm) abscesses usually are effectively treated with incision and drainage without an antimicrobial. Outpatient therapy of large (> 5 cm) abscesses includes incision and drainage and empiric clindamycin or TMP-SMX, depending on local susceptibility patterns. However, in many communities, 50% or more of MRSA isolates are also resistant to clindamycin, fluoroquinolones, and erythromycin. Knowledge of the local resistance pattern of community-acquired MRSA is helpful in choosing initial antimicrobial therapy. Adequate cultures, the thoroughness of drainage procedures, and careful outpatient follow-up are needed to ensure optimal outcomes. Group A streptococcal infections are not adequately treated with TMP-SMX.
SPECIFIC ANTIMICROBIAL AGENTS
PENICILLINS
Aminopenicillins
Penicillin remains the drug of choice for streptococcal infections including acute pharyngitis, acute rheumatic fever prophylaxis, syphilis, oral anaerobic infections, dental infections, N meningitidis infection, leptospirosis, rat-bite fever, actinomycosis, and infections due to Clostridium and Bacillus species (Table 39–8). For oral therapy of minor infections, amoxicillin or ampicillin is usually equivalent. For systemic therapy, aqueous penicillin G is preferable. For treatment of streptococcal pharyngitis, some experts recommend a first-generation cephalosporin. Amoxicillin is preferred therapy for pneumonia and otitis media due to S pneumoniae. Amoxicillin is preferred for oral therapy of Lyme disease in children. For dog or cat bites, where Pasteurella multocida is commonly encountered, amoxicillin-clavulanate provides good coverage of Pasteurella as well as Staphylococcus. An alternative is separate prescriptions for penicillin and an antistaphylococcal drug such as cephalexin, or clindamycin and TMP-SMX in penicillin-allergic patients. For human bites, amoxicillin-clavulanate provides adequate therapy for Eikenella corrodens and other mixed oral aerobes and anaerobes. The β-lactamase inhibitor sulbactam combined with ampicillin is given parenterally for human and animal bites, and some other infections due to organisms from the oral flora in which mixed aerobic and anaerobic bacteria may be resistant to ampicillin due to β-lactamase production.
Table 39–8. Antimicrobial prophylaxis and preferred prophylactic agents: selected conditions and pathogens.a
Penicillinase-Resistant Penicillins
S aureus is usually resistant to penicillin and amoxicillin owing to penicillinase production. Nafcillin, oxacillin, methicillin, and first- and second-generation cephalosporins are stable to penicillinase and are usually equivalent for intravenous therapy. Methicillin is associated with more frequent interstitial nephritis. Oxacillin and methicillin are renally excreted, whereas nafcillin is excreted through the biliary tract. These properties are occasionally considered in children with renal or liver failure. Cost should usually be the deciding factor for choosing an agent. Often both S aureus and S pyogenes are suspected initially (eg, in cellulitis or postoperative wound infections). The penicillinase-resistant penicillins (PRPs) and first- and second-generation cephalosporins are efficacious for most streptococcal infections, although penicillin remains the drug of choice.
MRSA is an increasingly common and serious community-acquired infection in children and may cause nosocomial infection. MRSA infections are also resistant to all of the PRPs and cephalosporins. Vancomycin is effective against MRSA and coagulase-negative staphylococci. S aureus infections range in severity from minor infections treated on an outpatient basis to life-threatening infections. Severe infections due to MRSA are a serious concern in many communities. It is important to culture and determine antimicrobial susceptibility of suspected S aureus infections. In communities with frequent MRSA infections in children, initial therapy of seriously ill children should include vancomycin. MRSA may also be resistant to macrolides and clindamycin by alteration in the bacterial 23s-ribosomal RNA. Many strains reported as clindamycin-susceptible and erythromycin-resistant are truly resistant to clindamycin. This inducible resistance to clindamycin may be detected in erythromycin-resistant MRSA that demonstrates a D-zone in disk susceptibility testing to clindamycin. Community-acquired MRSA is more likely to be susceptible to TMP-SMX, clindamycin, and tetracyclines than hospital-acquired infections.
For outpatient therapy, cloxacillin, dicloxacillin, and first- or second-generation cephalosporins are usually equally effective for infections due to susceptible S aureus. Cost may determine the choice between drugs.
Anti-Pseudomonas Penicillins
Ticarcillin, mezlocillin, and piperacillin are active intravenously against streptococci, ampicillin-susceptible enterococci, H influenzae, gram-negative rods (including more resistant gram-negative rods such as Enterobacter, Proteus, and P aeruginosa), and gram-negative anaerobes such as Bacteroides fragilis. P aeruginosa is inherently resistant to most antimicrobials, and high levels of these drugs are usually required. The combination of ticarcillin or piperacillin and an aminoglycoside is synergistic against P aeruginosa and many other enteric gram-negative rods. Ticarcillin in a fixed combination with clavulanic acid has activity against β-lactamase–producing strains of Klebsiella, S aureus, and Bacteroides. Piperacillin is more active in vitro against many gram-negative enteric infections and may be advantageous in some circumstances, but it is not approved in children. Piperacillin-tazobactam is another combination antimicrobial and β-lactamase inhibitor that has enhanced activity against many β-lactamase producers.
Antipseudomonal penicillins cause the same toxicities as penicillin and therefore are usually very safe. Carbenicillin, ticarcillin, and piperacillin contain large amounts of sodium, which may cause problems for some patients with cardiac or renal disease.
GLYCOPEPTIDE AGENTS
Vancomycin and teicoplanin are glycopeptide antimicrobial agents active against the cell wall of gram-positive organisms. Only vancomycin is licensed in the United States. Vancomycin is useful for parenteral therapy of resistant gram-positive cocci such as penicillin- and cephalosporin- resistant S pneumoniae, MRSA, methicillin-resistant coagulase-negative staphylococci, and ampicillin-resistant enterococci. Vancomycin is also used orally for therapy of colitis due to Clostridium difficile, although it should not be used as the drug of first choice.
The empiric use of vancomycin has increased tremendously over the last decade. As a result, vancomycin-resistant enterococci (VRE) and coagulase-negative staphylococci have become problems, particularly in inpatient units, intensive care units, and oncology wards. S aureus with increased MICs to vancomycin has been reported in the United States and Japan. This resistance is of concern because of the inherent virulence of many S aureus strains. Vancomycin use should be monitored carefully in hospitals and their intensive care units. It should not be used empirically when an infection is mild or when other antimicrobial agents are likely to be effective. Vancomycin should be stopped promptly if infection is found to be due to organisms susceptible to other antimicrobials.
Rapid infusion of vancomycin is associated with the “red man syndrome,” characterized by diffuse red flushing, at times pruritus, and occasionally tachycardia and hypotension. As a result, vancomycin is infused slowly over 1 hour or longer in some cases. Diphenhydramine or hydrocortisone (or both) may also be used as premedication.
Measurement of peak-and-trough serum vancomycin concentrations is not necessary in most clinical situations, because the levels achieved with standard dosing are usually predictable and nontoxic. Measurement of serum concentrations is helpful in patients with abnormal or unpredictable renal function; in those with altered volume of distribution, as occurs in nephrotic syndrome or shock; and in those receiving higher-dose therapy (eg, for meningitis or other difficult-to-treat infections). For patients receiving antimicrobials for weeks to months, weekly monitoring of clinical signs and symptoms and of urinalysis, creatinine, and complete blood count will allow detection of toxicity.
OXAZOLIDINONES
Linezolid is the first drug in this class of antimicrobials which have a distinct new mechanism of action; they bind to the 50s-ribosomal RNA subunit and prevent initiation of protein synthesis. Because of this unique mechanism, there is no cross-resistance with other classes of antimicrobials. The in vitro development of resistance has also been uncommon.
Linezolid is active against aerobic gram-positive organisms, including streptococci, staphylococci, enterococci, and pneumococci. Because linezolid is active against gram- positive organisms resistant to other antimicrobials (eg, MRSA, methicillin-resistant coagulase-negative staphylococci, VRE, and penicillin-resistant S pneumoniae), it is useful for these difficult-to-treat infections.
Linezolid is safe and well tolerated in children. Gastrointestinal symptoms are the most commonly encountered side effect. Neutropenia and thrombocytopenia have been reported, and linezolid should therefore be used with monitoring in patients at increased risk for these problems or in patients receiving therapy for 2 weeks or longer. Linezolid is an inhibitor of monoamine oxidase (MAO) and should not be used in patients taking MAO inhibitors, or in patients taking phenylpropanolamine or pseudoephedrine.
Linezolid should be used only for infections due to a proven gram-positive pathogen that is known or strongly suspected to be resistant to other available agents.
QUINUPRISTIN-DALFOPRISTIN
Quinupristin and dalfopristin are two antimicrobials of the streptogramin class, which individually are bacteriostatic, but in combination are synergistic and bacteriocidal. These drugs are combined in a fixed ratio of 70:30, known as Synercid. Streptogramins inhibit protein synthesis by binding to the 50s-ribosomal subunit. The streptogramins were discovered many years ago, but interest has increased only recently due to the activity of these agents against some very difficult-to-treat gram-positive infections.
The quinupristin-dalfopristin combination has activity against staphylococci, streptococci, pneumococci, and some enterococci. Quinupristin-dalfopristin is primarily indicated for serious infections due to vancomycin-resistant Enterococcus faecium and MRSA. Quinupristin-dalfopristin is not active against E faecalis and, therefore, differentiation of these strains from E faecium is important prior to initiating therapy.
Quinupristin-dalfopristin is not approved for therapy in children younger than 12 years. Nonetheless, therapy has been initiated in some pediatric patients seriously ill with difficult-to-treat infections due to resistant organisms.
Arthralgias and myalgias have at times been severe in adult patients. Other significant side effects include elevated bilirubin and inflammation at intravenous sites.
Quinupristin-dalfopristin is a significant inhibitor of CYP-450 3A4 and, therefore, must be used with caution in patients receiving drugs metabolized by this mechanism (eg, clarithromycin, itraconazole, erythromycin, and many others).
CEPHALOSPORINS
Cephalosporin agents make up a large and often confusing group of antimicrobials. Many of these drugs are similar in antibacterial spectrum and side effects and may have similar names. Clinicians should learn well the properties of one or two drugs in each class. Cephalosporins are often grouped as “generations” to signify their similar antimicrobial activity. First-generation cephalosporins such as cefazolin intravenously and cephalexin orally are useful mainly for susceptible S aureus infection and UTI due to susceptible E coli. Second-generation cephalosporins, such as cefuroxime intravenously and cefprozil and cefuroxime orally, have somewhat reduced, but acceptable, activity against gram-positive cocci, and greater activity against some gram-negative rods compared with first-generation cephalosporins. They are active against H influenzae and Moraxella catarrhalis, including strains that produce β-lactamase capable of inactivating ampicillin. Third-generation cephalosporins have substantially less activity against gram-positive cocci, such as S aureus, but greatly augmented activity against aerobic gram-negative rods. Cefotaxime and ceftriaxone are examples of intravenous drugs, whereas cefpodoxime and ceftibuten are representative oral drugs. Cefepime is often described as fourth-generation cephalosporins because of its broad activity against gram-positive and gram-negative organisms, including P aeruginosa. Cefepime is stable to β-lactamase degradation and is a poor inducer of β-lactamase. Cefepime will be most useful for organisms resistant to other drugs.
Ceftaroline is a new cephalosporin that has expanded activity against MRSA and some Enterococcus faecalis, while retaining the gram-negative activity of third-generation cephalosporins. Ceftaroline does not have sufficient activity against P aeruginosa or Acinetobacter to be useful. Ceftaroline is not approved for use in children younger than 18 years old.
No cephalosporin agent, except ceftaroline, has substantial activity against L monocytogenes, enterococci, or MRSA. The only cephalosporins useful for treating anaerobic infections are cefoxitin and cefotetan, which are second-generation cephalosporins with excellent activity against B fragilis. Ceftazidime and cefepime are cephalosporins with activity against P aeruginosa.
Allergy to β-lactam antimicrobials is reported commonly by parents. Immediate hypersensitivity reactions, including anaphylaxis or hives, most commonly predict IgE-mediated allergy. In contrast, most delayed reactions and nonspecific rashes are likely due to the underlying infection or nonallergic reactions. (See Chapter 38.) Cephalosporins should be used with caution in children with immediate hypersensitivity reactions to penicillins or cephalosporins.
Resistance to cephalosporins is common among aerobic gram-negative rods. The presence of inducible cephalosporinases in some gram-negative rod organisms, such as P aeruginosa, Serratia marcescens, Citrobacter, and Enterobacter, has led to clinical failures because of the emergence of resistance during therapy. Extended-spectrum β-lactamases mediate broad resistance to all penicillins, aminopenicillins, cephalosporins, and monobactams. Carbapenems, fluoroquinolones, or combinations including these drugs are used for serious infections due to gram-negative organisms with these enzymes. Active laboratory-based surveillance is necessary to detect resistance in these gram-negative organisms.
AZTREONAM
Aztreonam is the only monobactam antimicrobial agent approved in the United States. Although it is not approved for use in children younger than age 9 months, there is considerable pediatric experience with its use, including in neonates and premature infants. Aztreonam is active against aerobic gram-negative rods, including P aeruginosa. Aztreonam has activity against H influenzae and M catarrhalis, including those that are β-lactamase producers. Most patients with allergy to penicillin or cephalosporins are not sensitized to aztreonam, except that children with prior reactions to ceftazidime may have reactions to aztreonam because aztreonam and ceftazidime have a common side chain.
Aztreonam delivered by aerosolization by a proprietary nebulization system is approved for therapy of Pseudomonas aeruginosa infection in patients with cystic fibrosis. Safety and efficacy have not been established in children younger than 7 years. Therapy is delivered three times daily for 28 days with a goal to improve respiratory symptoms.
CARBAPENEMS
Meropenem, ertapenem, doripenem, and imipenem are broad-spectrum β-lactam antimicrobials. Imipenem-cilastatin is a combination of an active antibiotic and cilastatin, which inhibits the metabolism of imipenem in the kidneys and thereby results in high serum and urine levels of imipenem. These carbapenems are also active against S pneumoniae, including many penicillin- and cephalosporin-resistant strains. Carbapenems have been used successfully to treat meningitis and may be considered if vancomycin is not tolerated. An increased frequency of seizures is encountered when central nervous system infections are treated with carbapenems. These agents are broadly active against streptococci, methicillin-susceptible S aureus, some enterococci, and gram-negative rods such as P aeruginosa, β-lactamase–producing H influenzae, and gram-negative anaerobes. Ertapenem has less activity against P aeruginosa, Acinetobacter species, and Enterococcus species than meropenem and imipenem. Because carbapenems are active against so many species of bacteria, there is a strong temptation to use them as single-drug empiric therapy. Units that have used carbapenems heavily have encountered resistance in many different species of gram-negative rods.
MACROLIDES & AZALIDES
Erythromycin was once the most commonly used macrolide antimicrobial agent, but now azithromycin and clarithromycin are preferred because of decreased side effects. It is active against many bacteria that are resistant to cell wall–active antimicrobials, and is the drug of choice for Bordetella pertussis, Legionella pneumophila, C pneumoniae, M pneumoniae, and Chlamydia trachomatis infections (in children in whom tetracycline is not an option). Erythromycin is used for outpatient therapy of streptococcal and staphylococcal infections in patients with penicillin allergy. More serious infections due to streptococci and staphylococci are usually treated with penicillins, clindamycin, PRPs, or cephalosporins because of a significant incidence of erythromycin resistance in both species. S pneumoniae resistant to erythromycin and the related macrolides are now frequent in many communities. This limits the utility of macrolide antimicrobials for therapy of pneumonia, otitis media, and sinusitis. Gastrointestinal side effects are common. Interactions with theophylline, carbamazepine, terfenadine, cycloserine, and other drugs may require dosage modifications of erythromycin and clarithromycin. Significant interactions with azithromycin are less common. Erythromycin exposure is associated with pyloric stenosis in newborns, so azithromycin is preferred in most neonates.
Erythromycin is available in many formulations, including the base, estolate, ethyl succinate, and stearate, but is sometimes unavailable due to drug shortages and decreased prescribing of these drugs. Transient hepatic toxicity occurs in adults, but is much less common in children. Erythromycin base and stearate should be taken with meals for best absorption.
Clarithromycin and azithromycin, macrolide and azalide antimicrobials, respectively, are much less likely than erythromycin to cause nausea, vomiting, and diarrhea. These agents are useful in children who cannot tolerate erythromycin. Clarithromycin is more active than erythromycin against H influenzae, M catarrhalis, and Neisseria gonorrhoeae and is the drug of choice, usually in combination, for some nontuberculous mycobacterial infections. Azithromycin has a prolonged tissue half-life that achieves a prolonged antimicrobial effect. Azithromycin is dosed once daily for 5 days, but must be taken 1 hour before or 2 hours after meals because food interferes with absorption. Although azithromycin is active against H influenzae, some authors report poor eradication of H influenzae from the middle ear. Azithromycin can be used for single-dose therapy of C trachomatis infections. It is beneficial in adolescents when compliance with erythromycin or tetracycline is a concern. Azithromycin is useful for therapy of Shigella and Salmonella infections, including typhoid fever resistant to ampicillin and TMP-SMX. Alternatives include third-generation cephalosporins and fluoroquinolones. Clarithromycin is effective against Lyme disease, but amoxicillin is the drug of choice in young children. Clarithromycin and azithromycin are alternative drugs for toxoplasmosis in sulfonamide-allergic patients and as alternatives to erythromycin in legionellosis. In vitro and limited clinical experience in providing treatment to contacts of pertussis patients suggests efficacy equal to that of erythromycin. Clarithromycin and azithromycin are considerably more expensive than most erythromycin formulations, and for that reason are usually preferred, but they are advantageous by virtue of their twice-daily and once-daily dosing, respectively. Some failures of the newer macrolides have occurred in S pneumoniae sepsis and meningitis, perhaps because of low serum levels despite the high tissue levels achieved. High rates of resistance to macrolides and azalides have been encountered in some communities. The frequent use of azithromycin for respiratory infections and acute otitis media has contributed to selection of resistant strains. Azithromycin is not recommended as first-line therapy for S pneumonia infection.
CLINDAMYCIN
Clindamycin is active against S aureus, some MRSA, S pyogenes, other streptococcal species except enterococci, and both gram-positive and gram-negative anaerobes. Clindamycin or metronidazole is frequently combined with other antimicrobials for empiric therapy of suspected anaerobic or mixed anaerobic and aerobic infections. Empiric use of clindamycin is justified in suspected anaerobic infections because cultures frequently cannot be obtained and, if obtained, may be slow in confirming anaerobic infection. Examples are pelvic inflammatory disease, necrotizing enterocolitis, other infections in which the integrity of the gastrointestinal or genitourinary tracts is compromised, and sinusitis. Clindamycin does not achieve high levels in CSF, but brain abscesses, toxoplasmosis, and other central nervous system infections, where disruption of the blood-brain barrier occurs, can be successfully treated with clindamycin. Clindamycin should be added to regimens for treatment of serious streptococcal and staphylococcal infections, such as necrotizing fasciitis and toxic shock syndrome. Both in vitro and clinical data suggest increased bactericidal killing, and improved outcomes occur with clindamycin. For most oral anaerobes (eg, in a dental abscess), penicillin is more active than clindamycin. Clindamycin has been associated with the occurrence of C difficile–related pseudomembranous colitis. Although diarrhea is a frequent side effect, pseudomembranous colitis is uncommonly due to clindamycin in children.
SULFONAMIDES
Sulfonamides—the oldest class of antimicrobials—remain useful for treatment of UTIs, and for other infections due to E coli and for Nocardia. Although useful for rheumatic fever prophylaxis in penicillin-allergic patients, sulfonamides fail to eradicate group A streptococci and cannot be used for treatment of acute infections.
TMP-SMX is a fixed combination that is more active than either drug alone. Gram-positive cocci, including some S pneumoniae, many staphylococci, Haemophilus, and many gram-negative rods, are susceptible. Unfortunately, resistance to TMP-SMX has become more common. S pneumoniae resistant to penicillin and cephalosporins is often also resistant to TMP-SMX and erythromycin. In communities, where Shigella and Salmonella enteritidis strains remain susceptible, as do most E coli strains, TMP-SMX is very useful for treatment of both UTIs and bacterial dysentery. TMP-SMX is also the drug of choice for treatment or prophylaxis against Pneumocystis jiroveci infection. Myelosuppressive side effects especially anemia limit the use of TMP-SMX in some children infected with HIV.
Sulfonamide is associated with several cutaneous reactions, including urticaria, photosensitivity, Stevens-Johnson syndrome, purpura, and maculopapular rashes. Hematologic side effects such as leukopenia, thrombocytopenia, and hemolytic anemia are uncommon. Common gastrointestinal side effects are nausea and vomiting. The dermatologic and hematologic side effects are thought to be more common and more severe with TMP-SMX than with sulfonamide alone.
TETRACYCLINES
Tetracyclines, which are effective against a broad range of bacteria, are not commonly used in children because alternative effective drugs are available. Many different tetracycline formulations are available. Tetracyclines are effective against B pertussis and E coli and many species of Rickettsia, Chlamydia, and Mycoplasma. Doxycycline or minocycline is the drug of choice for eradication of C trachomatis in pelvic inflammatory disease and nongonococcal urethritis.
Staining of permanent teeth was noted in young children given repeated courses of tetracyclines. As a result, tetracyclines are generally not given to children younger than age 9 years unless alternative drugs are unavailable. A single course of tetracycline does not pose a significant risk of tooth staining. Mucous membrane candidiasis, photosensitivity, nausea, and vomiting are other common side effects. Tetracycline should be taken on an empty stomach, either 1 hour before or 2 hours after a meal. Doxycycline is well absorbed even in the presence of food; administration with food may minimize gastrointestinal side effects. Doxycycline is often preferred because it is better tolerated than tetracycline, and twice-daily administration is convenient.
Tetracycline is used for therapy of rickettsial infections such as Rocky Mountain spotted fever, ehrlichiosis, rickettsialpox, murine typhus, and Q fever; as an alternative to erythromycin for M pneumoniae and C pneumoniae infections; and for treatment of psittacosis, brucellosis, P multocida infection, and relapsing fever.
Tigecycline is a new polyketide antimicrobial that is an analogue of tetracycline and a bacteriostatic inhibitor of protein synthesis. Tigecycline is active against gram-negative aerobes, anaerobes, and many gram-positive cocci including MRSA. It is approved for intravenous therapy of adults with complicated SSTIs and complicated intra-abdominal infections.
AMINOGLYCOSIDES
The aminoglycosides bind to ribosomal subunits and inhibit protein synthesis. They are active against aerobic gram-negative rods, including P aeruginosa. Streptomycin was the first drug in this class, but today it is used only to treat tuberculosis and the occasional cases of plague and tularemia.
Aminoglycosides are used to treat serious gram-negative infections and are given intravenously or intramuscularly. They are also used to treat pyelonephritis, suspected gram-negative sepsis, and in other settings where P aeruginosa infections are common, such as cystic fibrosis and burns. Aminoglycosides have activity against gram-positive organisms and, combined with penicillin or ampicillin, may achieve synergistic killing of L monocytogenes and group B streptococci. Penicillin, ampicillin, or vancomycin combined with gentamicin is indicated for therapy of serious enterococcal infections, such as sepsis or endocarditis because of more rapid clinical improvement with combined therapy. Aminoglycosides have activity against S aureus, but are always used in combination with other antistaphylococcal antibiotics.
Aminoglycosides are not active in an acidic environment and may not be effective against abscesses. Aminoglycosides diffuse poorly into the CSF and achieve only about 10% of serum concentrations. As a result, a third-generation cephalosporin is preferred for treatment of meningitis.
Aminoglycosides kill bacteria in a concentration-dependent manner. They also have a prolonged suppressive effect on the regrowth of susceptible organisms (postantibiotic effect). These principles have led some investigators to establish guidelines for once-daily dosing of aminoglycosides, using larger initial doses given every 24 hours. Although aminoglycosides are associated with both renal and eighth nerve toxicity, the entry of the drug into renal and cochlear cells is saturable. It therefore was predicted that once-daily dosing would result in less toxicity than traditional twice-daily or three-times-daily dosing. Studies in adult patients confirm that once-daily dosing is as efficacious as traditional dosing and is associated with less toxicity. Although there is extensive experience with dosing intervals of 18–36 hours in premature infants, small total daily doses are customarily used (2.0–2.5 mg/kg per dose of gentamicin or tobramycin). A convenient and cost-effective approach in children is based on the experience with adult patients and uses larger daily doses (4–7 mg/kg per dose every 24 hours). Nevertheless, traditional twice-daily or three-times-daily dosing regimens of aminoglycosides, with monitoring of serum levels, are still widely used. Careful monitoring is necessary, particularly in children with abnormal or changing renal function, premature infants, and infants with rapidly changing volumes of distribution. Aminoglycosides are usually infused over 30–45 minutes, and the peak serum concentration is measured 30–45 minutes after the end of the infusion. A trough serum concentration is measured prior to the next dose. The efficacious and nontoxic serum concentrations for gentamicin and tobramycin are trough less than 2 mcg/mL and peak 5–10 mcg/mL; for amikacin, trough less than 10 mcg/mL and peak 15–25 mcg/mL (see Table 39–4). Aminoglycoside levels and creatinine levels should be measured in children expected to receive more than 3 days of therapy, and repeated weekly in children on long-term therapy, even when renal function is normal and stable.
FLUOROQUINOLONES
Modification of the quinolone structure of nalidixic acid has led to many new compounds called fluoroquinolones, which are well absorbed after oral administration and possess excellent antibacterial activity against resistant gram-negative pathogens. The currently available fluoroquinolones vary in their activity against specific organisms. Fluoroquinolones are active against most of the Enterobacteriaceae, including E coli, Enterobacter, Klebsiella, in some cases P aeruginosa, and many other gram-negative bacteria such as H influenzae, M catarrhalis, N gonorrhoeae, and N meningitidis. Some fluoroquinolones (ofloxacin and levofloxacin) are active against C trachomatis and Mycoplasma. The fluoroquinolones are active against some enterococci, S aureus, MRSA, and coagulase-negative staphylococci. The newer quinolones have good activity against penicillin- and cephalosporin-resistant S pneumonia.
Ciprofloxacin and its otic and ophthalmic preparations are the only fluoroquinolone antimicrobials currently approved for use in children older than 1 year, although fluoroquinolones offer very attractive alternatives to other approved agents. The objection to quinolones is based on the recognition that nalidixic acid and other quinolones cause arthropathy when tested experimentally in newborn animals of many species. The fear that children would also be more susceptible than adults to cartilage injury has not been realized in clinical experience. Both retrospective long-term follow-up studies of children given nalidixic acid and prospective studies of children receiving treatment under protocols with fluoroquinolones have shown similar rates of toxicity compared with adult patients. Arthropathy occurs uncommonly, although tendon rupture is a reported rare, serious complication which occurs more commonly in adults. For these reasons, quinolones should be considered for use in children when the benefit clearly outweighs the risk, when no alternative drug is available, and after discussion with the parents.
Ciprofloxacin is useful for oral therapy of resistant gram-negative UTIs, such as those caused by P aeruginosa. Fluoroquinolones are no longer recommended for single-dose therapy of gonorrhea due to frequent resistance, but ofloxacin and levofloxacin are an alternative therapy for treating Chlamydia infections and pelvic inflammatory disease. Ciprofloxacin, levofloxacin, and ofloxacin are used as therapy of resistant cases of shigellosis. Levofloxacin and ciprofloxacin are usually the drugs of choice for treatment of traveler’s diarrhea. Ciprofloxacin is useful for treatment of P aeruginosa infection in patients with cystic fibrosis, and as therapy for chronic suppurative otitis media. Several quinolones are used as therapy for pneumonia due to Legionella, Mycoplasma, or C pneumoniae, although a macrolide is often preferred, and as prophylactic therapy of meningococcal infection. Ofloxacin and levofloxacin are used for treatment of some cases of Mycobacterium tuberculosis and some atypical mycobacterial infections.
METRONIDAZOLE
Metronidazole has excellent activity against most anaerobes, particularly gram-negative anaerobes, such as Bacteroides and Fusobacterium, and against gram-positive anaerobes such as Clostridium, Prevotella, and Porphyromonas. Gram-positive anaerobic cocci such as Peptococcus and Peptostreptococcus are often more susceptible to penicillin or to clindamycin. Because metronidazole lacks activity against aerobic organisms, it is usually given with one or more other antibiotics. Metronidazole is well absorbed after oral administration and has excellent penetration into the central nervous system. Metronidazole is the drug of choice for bacterial vaginosis and for C difficile enterocolitis. It is active against many parasites, including Giardia lamblia and Entamoeba histolytica.
DAPTOMYCIN
Daptomycin has broad bactericidal activity against gram-positive cocci. Daptomycin is a lipopeptide that binds to bacterial cell membranes, resulting in membrane depolarization and cell death. Daptomycin is active against methicillin- sensitive and -resistant S aureus, S pyogenes, and Streptococcus agalactiae, as well as E faecium (including vancomycin- resistant strains) and E faecalis (vancomycin-sensitive strains). Daptomycin is given as a once-daily intravenous infusion of 4 mg/kg and is approved for therapy of complicated SSTIs in adults, but has not been sufficiently studied in children younger than 18 years to make recommendations for dosing and use. Daptomycin therapy of pneumonia was unsuccessful in a large percentage of cases, and should not be used. Daptomycin is excreted renally, so a modification of dosing is needed in patients with impaired renal function. Nausea, constipation, and headache are the most common side effects of therapy. In patients with muscle pain, monitoring creatinine phosphokinase levels should be done.
REFERENCES
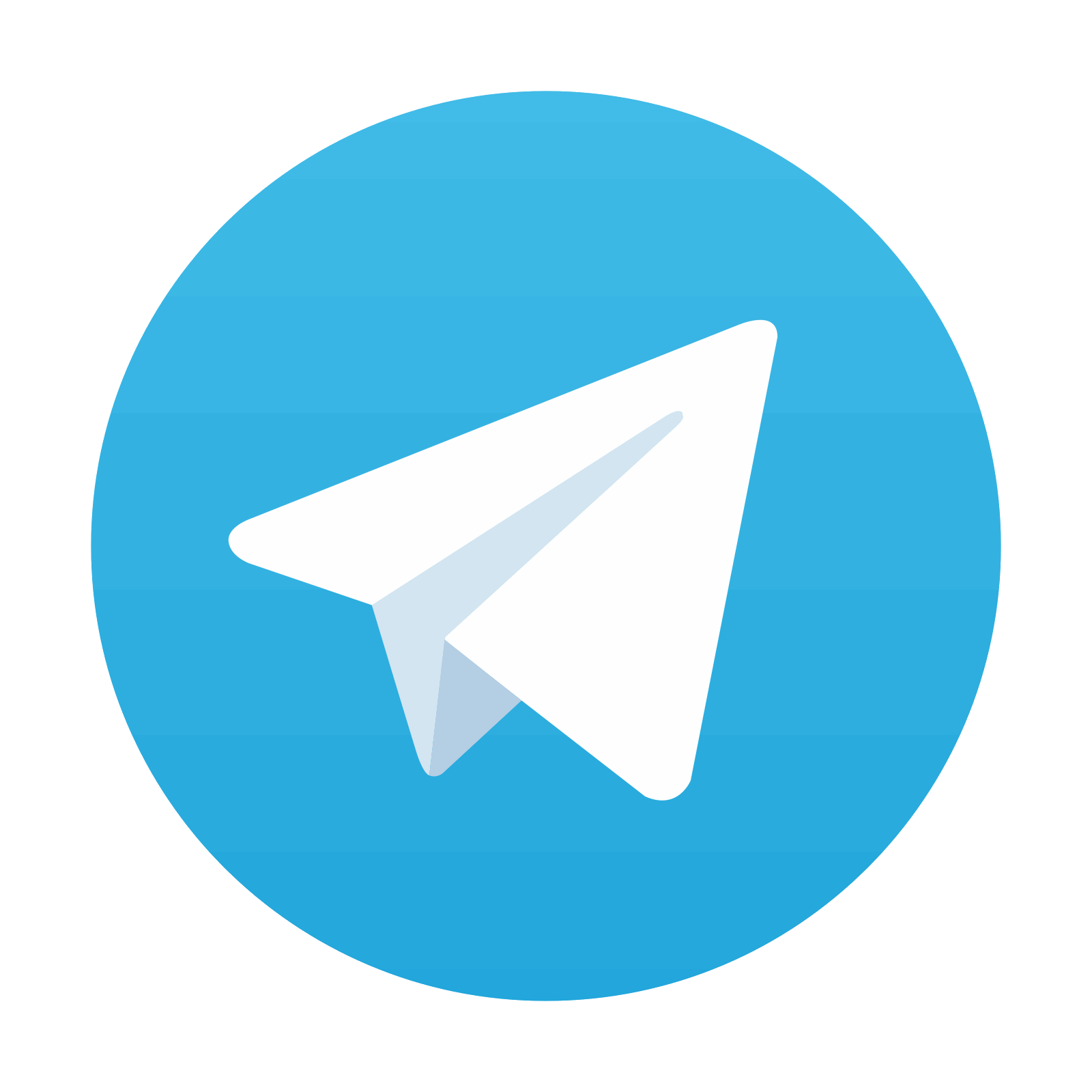
Stay updated, free articles. Join our Telegram channel

Full access? Get Clinical Tree
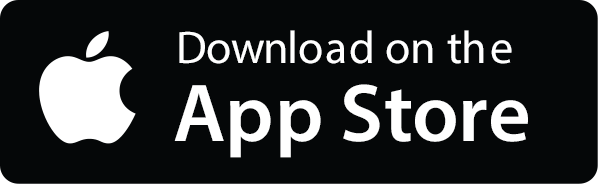
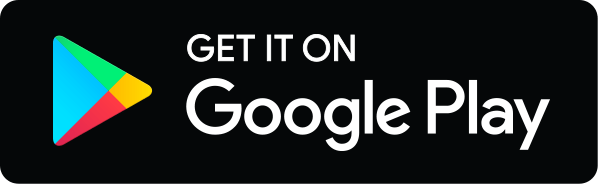