Fig. 8.1
The maternal–fetal vascular system
Although it is one of the most intriguing of the human organs, the umbilical cord is also one of the least investigated [1], mainly because of the changes in cord structure occurring after birth and the difficulty to study such an organ by ultrasound (Fig. 8.2) during pregnancy, which has the burden of being highly operator dependent. This is a severe limit in obstetrics since several obstetrical syndromes, including intrauterine growth restriction (IUGR), stillbirth, and preeclampsia, have been associated to cord anomalies [2].


Fig. 8.2
A transabdominal ultrasonographic scan showing, with the white arrow, the umbilical cord emerging from the fetus at 14 weeks of pregnancy
8.1.1 Normal Anatomy of the Umbilical Cord
A normal umbilical cord is made of two arteries and one vein and is included within a homogeneous substance called Wharton’s jelly, which is a myxomatous connective tissue that varies in size and may be imaged with high-frequency ultrasound transducers [3] (Fig. 8.3).


Fig. 8.3
Transvaginal ultrasonographic scan showing an umbilical cord section, in a patient at 28 weeks of pregnancy with placenta previa
The cord is covered by the amniotic membranes (Fig. 8.4), and its diameter usually measures between 1 and 2 cm at term (variations in cord diameter are usually attributed to Wharton’s jelly volume, but might also be dependent on the caliber of the vessels), while it has an average length of 55–61 cm at term. Such a length is sufficient to allow a vaginal delivery to be accomplished in the presence of a fundal implantation of the placenta [4].


Fig. 8.4
The umbilical cord anatomy (modified from: Antonio Malvasi Gian Carlo Di Renzo, Semeiotica Ostetrica. C.I.C. International Publisher, Rome, Italy, 2012)
The umbilical cord develops throughout gestation, although its growth becomes slower after 28 weeks. At 6 weeks postconception, the cord has a mean length of about 0.5 cm (Fig. 8.5); by the fourth month, it averages between 16 and 18 cm (Fig. 8.6); and at the sixth month of gestation, it reaches a length of 33–35 cm [5] (Fig. 8.7).




Fig. 8.5
An embryo with the umbilical cord at 6 weeks of pregnancy

Fig. 8.6
A cross-scan representation of the fetus at 20 weeks of pregnancy, at inset source level of the cord in the fetus (modified from: Antonio Malvasi Gian Carlo Di Renzo, Semeiotica Ostetrica. C.I.C. International Publisher, Rome, Italy, 2012)

Fig. 8.7
A representation of a fetus at 24 weeks with the complete development of the umbilical cord
Standard curves for cord length have been provided from 34 to 43 weeks gestation [4].
The umbilical arteries arise from the fetal internal iliac arteries, course alongside the fetal bladder, and exit the umbilicus to form part of the umbilical cord. The paired umbilical arteries surround the umbilical vein with a helicoidal pattern for the entire length of the cord completing 10–11 coils between the fetal and placental insertion sites [3], and then they branch along the chorionic plate of the placenta.
The umbilical vein is the result of the confluence of the chorionic veins of the placenta. Its primary goal is the transport of oxygenated blood to the fetus. It enters the umbilicus and joins the left portal vein as it courses through the liver. The umbilical vein also presents a connection with the inferior vena cava that is provided through the ductus venosus, that shunts a third of the oxygenated blood directly toward the heart instead of entering the liver circulation [6]. The intra-abdominal portions of the umbilical vessels degenerate after birth; the umbilical arteries become the lateral ligaments of the bladder, while the umbilical vein regresses to be the round ligament of the liver [6].
The umbilical cord is usually twisted or coiled counterclockwise, to the left, with a left-to-right ratio of about 7:1 [5]. The average number of coils in the entire length of cord is about 40, with approximately 0.2 coils per 1 cm of cord. The latter is referred to as the coiling index [7]. Coiling is established early in gestation and can be seen by sonography as early as the ninth week. The origin of umbilical cord coiling has long been debated, but some data suggest that it is due, at least in part, to fetal movements.
8.1.2 Embryology of the Umbilical Cord
The umbilical cord forms during the first 5 weeks of gestation (7 menstrual weeks) as a fusion of the omphalomesenteric yolk stalk and allantoic ducts. An outpouching from the urinary bladder forms the urachus, which projects into the connecting stalk to form the allantois. The allantoic vessels become the definitive umbilical vessels. The umbilical cord acquires its epithelial lining after the enlargement of the amniotic cavity and envelopment of the cord by the amniotic membrane. The intestines grow at a faster rate than the abdomen; they herniate into the proximal umbilical cord at approximately 7 weeks and remain there until approximately 10 weeks. The insertion of the umbilical cord into the ventral abdominal wall is an important sonographic anatomic landmark because the evaluation of this area will reveal abdominal wall defects, such as omphalocele, gastroschisis, or limb–body wall complex [8].
8.2 Type of Cord Anomalies
There are several abnormalities that can be recognized in the umbilical cord and may affect pregnancy outcome and especially neonatal outcomes. They can be identified and classified as follows: (1) structural or morphologic anomalies, meaning that either the cord does not have its typical three-vessel structure or that it shows some alterations deviating from the normal anatomy described above; (2) anomalies leading to cord compression within a condition of normal structure, which is the largest group; and (3) conditions of histological cord pathology, with a normal structure and morphology, which includes obstetrical emergencies involving the umbilical cord during or before labor.
Table 8.1 summarizes the above mentioned classes of cord anomalies, but the reader might take into account that an overlapping of different classes of anomalies is always possible, since the division into groups is only an attempt to provide a clearer description of such a challenging subject. It is indeed absolutely possible that an umbilical cord with an abnormal structure or morphology becomes affected because of compression, or that it acquires histologic changes due to an additional pathologic event.
Table 8.1
Classification of cord anomalies
Class of anomaly | List of anomalies included in the class |
---|---|
Structural or morphologic anomalies | Single umbilical artery |
Persistent right umbilical vein | |
Marginal or velamentous insertion of the umbilical vessels | |
Vasa previa | |
Furcate cord insertion | |
Cord varix | |
Cysts of the umbilical cord | |
Aneurysms and hemangiomas of the umbilical cord | |
Abnormal coiling | |
Anomalies of cord diameter or length | |
Anomalies leading to cord compression | Cord entanglements |
Knots of the umbilical cord | |
Cord prolapse | |
Conditions of histological cord pathology | Funisitis (FIRS) |
Meconium-associated damage of the umbilical cord |
8.3 Structural or Morphologic Anomalies of the Umbilical Cord
8.3.1 Single Umbilical Artery
Single umbilical artery (SUA) refers to a variation of the umbilical cord that presents only one artery instead of the normal number of two (Fig. 8.8). This condition has a reported incidence of 0.2–2 % of pregnancies [9] and is shown to be associated with several fetal structural and chromosomal abnormalities (including cardiac, gastrointestinal, and renal anomalies) leading to fetal and neonatal complications [10].


Fig. 8.8
Section of a two-vessel cord
Thus, the American Institute of Ultrasound in Medicine recommends imaging of the umbilical cord including the number of vessels in the cord during routine prenatal ultrasound examinations and, if a SUA is identified, a targeted ultrasound is warranted to rule out known associated anomalies [11]. In the majority of cases, SUA is an isolated finding with no other apparent abnormality on ultrasound [12–14].
Much of the research conducted on isolated SUA focused on fetal growth. Many studies [9, 10, 15–22] have shown that isolated SUA is associated with fetal growth restriction and SGA infants although other studies [23–26] and a meta-analysis on this topic refuted this claim [27].
Several studies evaluating the significance of isolated SUA revealed associations with preterm delivery [9, 10, 15, 16] and suggested isolated SUA to be an independent risk factor for perinatal mortality [18], although others failed to establish such associations [23, 27, 28] and, therefore, the meaning of this finding is controversial. Recent studies found an increased rate of cesarean deliveries (mainly due to non-reassuring fetal heart rate patterns) and lower umbilical cord blood pH [17] for these fetuses.
With regard to perinatal mortality, data is again inconclusive. The same meta-analysis mentioned above [27] concluded that there was a trend toward higher rates of perinatal mortality in isolated SUA pregnancies, but this did not reach statistical significance.
The contradictory results regarding the relationship between isolated SUA and perinatal outcomes may be explained by the fact that studies in which increased perinatal mortality [10, 16, 18] was demonstrated included in their analysis fetuses with growth restriction or premature deliveries (before 37 weeks), while studies which found no increased risk of perinatal mortality [9, 17, 29] had smaller sample sizes or were conducted only for live-born infants [30].
To overcome this, in a recent report, Gutvirtz et al. [31] evaluated perinatal outcomes of fetuses with isolated SUA and no other risk factors for perinatal complications. They excluded other well-established risk factors for perinatal mortality (multiple gestations, structural and chromosomal abnormalities, prematurity, and growth-restricted fetuses) and controlled for possible confounders (placental abruption, birth weight, gestational age) in an effort to isolate any independent association of this finding with adverse perinatal outcomes.
The cohort studied by this group is the largest reported in the literature with 786 cases of isolated SUA, all reaching 37 weeks of gestation. These authors have demonstrated that isolated SUA at term carries a significant risk of adverse perinatal outcome and increased perinatal mortality [31].
The reason why isolated SUA may lead to adverse perinatal outcomes remains unclear. One possible explanation of the increased rates of perinatal mortality in an otherwise healthy term fetus relates to some structural deviations which may, hypothetically, increase the risk of a cord accident. Lacro et al. [32] found an increased incidence of absent umbilical cord twist in SUA cords, while Raio et al. [33] observed a reduction of Wharton’s jelly volume in these cords, and both groups showed an association with an increased incidence of stillbirth.
Currently, isolated SUA is not considered an indication for labor induction according to commonly used formal guidelines. However, due to the available literature, fetuses with isolated SUA should undergo closer monitoring of their well-being in order to prevent possible pregnancy complications and poor outcomes associated with this anomaly.
8.3.2 Persistent Right Umbilical Vein
Persistent right umbilical vein is the consequence of an altered development of the umbilical cord between fourth and seventh weeks of gestation when the left umbilical vein regresses but the right umbilical vein remains patent. This results in an abnormal blood flow in the fetal liver [34]. This anomaly occurs in 0.1–0.3 % of pregnancies and may be isolated or associated with other anomalies, especially in fetuses with situs inversus and heterotaxy, but also with genitourinary, gastrointestinal, cardiac, and skeletal developmental disorders [35].
Several sonographic findings on standard transverse images of the fetal abdominal circumference assist in the diagnosis of persistent right umbilical vein [36]. The fetal portal vein is curved toward the stomach instead of being approximately parallel to it, the fetal gallbladder is medial to the umbilical vein instead of appearing in its normal lateral position and is seen between the umbilical vein and the stomach, and the umbilical vein is connected to the right portal vein instead of the left [34]. Color Doppler is useful to determine the specific type of this anomaly according to the path of its drainage. In the more common intrahepatic persistent right umbilical vein, the isolated right umbilical vein joins the fetal portal system at the sinus venosus and gives rise to the ductus venosus. In extrahepatic persistent right umbilical vein, the right umbilical vein bypasses the liver completely and drains directly into the right atrium, inferior vena cava, or right iliac vein, with the absence of the ductus venosus [35, 37].
8.3.3 Abnormal Insertion of the Cord Vessels
Abnormal cord insertions include conditions in which the umbilical cord does not insert on the fetal surface of the placenta at or near its center (Fig. 8.9). These conditions may be associated with abnormal fetal heart rate tracings, fetal growth restriction, low birth weight, low Apgar scores [5, 38, 39], and adverse perinatal outcome associated to complications, such as disruption, thrombosis, or compression of the abnormally inserted cord vessels.


Fig. 8.9
Normal cord insertion
8.3.3.1 Marginal and Velamentous Insertion of the Umbilical Cord
In about 7 % of term placentas, the cord inserts marginally, and in about 1 %, it inserts within the membranes, and this is known as velamentous insertion [5, 40]. Both marginal and velamentous insertions are more common in twins [41]. Velamentous vessels run within the free membranes without the protection of Wharton’s jelly and are thus susceptible to thrombosis, compression, or disruption especially after membrane rupture when the added protection afforded by the amniotic fluid is lost. A velamentous cord may insert within a few centimeters of the placental margin or far away from it. Close insertion is much more common, representing a less severe condition.
Thrombosis of velamentous vessels has been associated with neonatal purpura and fetal death [41].
8.3.3.2 Vasa Previa
Velamentous vessels may cross the cervical os, preceding the presenting fetal part, determining a condition called vasa previa (Fig. 8.10). These vessels may be disrupted if a vaginal delivery is attempted. Hemorrhage from ruptured velamentous vessels is uncommon, occurring in about 1 in 50 velamentous cord insertions, but if rupture does occur, the mortality rate is estimated to be from 58 to 73 % [42]. Other outcomes associated with this condition include fetal distress and hypoxia from compression of the vessels during labor, neonatal thrombocytopenia, or severe neurologic impairment [43].


Fig. 8.10
Vasa previa characterized by velamentous vessels may cross the cervical os, preceding the presenting fetal part (the clamp highlights the blood vessels)
8.3.3.3 Furcate Cord Insertion
Furcate cord insertion is a rare abnormality in which the umbilical vessels separate from the cord substance before reaching the surface of the placenta. Like a velamentous insertion, these vessels have lost the protection afforded by Wharton’s jelly and are prone to thrombosis and injury [41].
8.3.4 Cord Varix
The diameter of the normal fetal intra-abdominal umbilical vein is approximately 3 mm at 15 weeks of gestation and grows until up to 1 cm at term [44]. Cord varix is a rare condition characterized by focal dilatation of the umbilical vein that can occur in the intrahepatic portion of the vein or with an extrahepatic location [45]. It is associated with a 5.8 % risk for aneuploidy, especially trisomy 21, and a 28 % risk for other fetal anomalies such as hydrops, anemia, and IUGR [46]. The prognosis is worsened by early identification in pregnancy or association with fetal anomalies [46–48]. Although most cases have a favorable outcome, fetal death may occur in case of formation of a thrombus in the dilated segment of the vein obstructing fetal circulation or due to fetal cardiac insufficiency due to volume overload [47].
Although the definite size of cord varix is not well established, some authors consider a vein diameter abnormal if (a) >9 mm, (b) the transverse diameter of the extrahepatic umbilical vein is at least 1.5 times greater than the intrahepatic component, or (c) the vein diameter is more than two standard deviations above the mean diameter appropriate for gestational age [49]. Doppler ultrasound examination is critical in order to diagnose a cord varix. The sonographic appearance of an intra-abdominal varix is of an oblong cystic structure obliquely oriented in a craniocaudal direction between the abdominal wall and the inferior edge of the liver [46]. Weissmann-Brenner et al. [48] suggest that turbulent flow within a varix at color Doppler imaging is associated with a large varix size, premature delivery, and lower birth weights, while Cohen et al. [49] hypothesized that a linear bidirectional flow at color Doppler ultrasound imaging could be related to a tortuous intra-abdominal segment of the umbilical vein rather than to a varix.
A fetus with cord varix should be monitored with serial ultrasound examinations from diagnosis to delivery. If other fetal anomalies are detected, fetal karyotyping should be considered to diagnose or exclude aneuploidy. Fetal echocardiography is usually indicated because cord varix is associated with fetal cardiac abnormalities [50].
This condition should undergo differential diagnosis with other cystic structures that may be observed at sonographic examination of the fetal abdomen and cord, including normal fetal gallbladder and stomach; cystic masses such as a urachal, duplication, mesenteric, or omental cyst; umbilical artery aneurysm; and a cyst of the umbilical cord [47].
8.3.5 Cysts of the Umbilical Cord
Sonographic examination allows the identification of cystic lesions of the umbilical cord at various stages of gestation (Fig. 8.11). During the first trimester, the prevalence of umbilical cord cysts ranges from 0.4 to 3.4 % [51–55]. The prevalence of umbilical cord cysts in the second and third trimesters is unclear and based only on case reports or small series [56–70]. Umbilical cord cysts are usually classified as true cysts or pseudocysts. True cysts are derived from the embryological remnants of either the allantois or the omphalomesenteric duct, are typically located toward the fetal insertion of the cord, and range from 4 to 60 mm in size [71–73].


Fig. 8.11
A representation of a cyst of the umbilical cord
Pseudocysts are more common than true cysts and can be located anywhere along the cord; they have no epithelial lining and represent localized edema and liquefaction of Wharton’s jelly [74]. To differentiate between true cysts and pseudocysts on ultrasound imaging is a challenging task. Histological confirmation of the ultrasound diagnosis has been obtained in only a minority of reported cases, which makes it difficult to define the clinical significance of different types of cord cysts.
In addition, these lesions may be multiple or single and central or located near the fetal or the placental insertion of the cord [75].
8.3.5.1 Outcomes and Additional Anomalies Associated with Cystic Lesions of the Umbilical Cord
The finding of an umbilical cord cyst in a pregnant woman raises some main questions: what is the perinatal outcome of those fetuses with a diagnosis of cord cyst? Is there any association with chromosomal disorders and additional fetal structural defects?
As stated above, there is a paucity of data regarding affected pregnancies, so the answer to these questions remains unclear. However, the literature shows that the majority of first trimester cysts are transient findings that have no adverse effect on pregnancy outcome [51, 52, 54], while a diagnosis of second or third trimester cysts of the umbilical cord may be associated with fetal anomalies. This is described in the reports by Smith et al. and Sepulveda et al. who found that fetal anomalies were associated with cystic lesions of the cord in 80–85 % of the cases. In the study of Ross et al. [53], 100 % correlation was reported between persistent second trimester cysts and anomalies, while Shipp et al. [68] reported fetal anomalies in 38 % of the cases.
Among the structural defects, a remarkable association exists between umbilical cord cysts and abdominal wall anomalies, including omphalocele [56, 58, 76–78] and patent urachus [57, 63, 66, 79–85]. The correct diagnosis is crucial in these cases because the outcome of neonates with patent urachus is usually favorable after surgical closure of the defect, while omphalocele requires complicated serial abdominal wall reconstruction and is highly associated with aneuploidy.
The body of literature regarding the diagnosis of these lesions during the second and third trimester is reported herein:
- 1.
Shipp et al. [68] were the first to present a series of 13 cases of umbilical cord cystic lesions detected during the second and third trimesters. They reported four cases of clear cysts on the umbilical cord, eight cases with complex masses, and one case with complete cystic morphology of the cord throughout its length. The postnatal outcome included eight normal neonates (of them one with omphalitis and chorioamnionitis), one case of trisomy 13, two cases of patent urachus, one case with multiple vascular anomalies (ventricular septal defect, superior vena cava and innominate vein varicosity, and asymmetric venous dilatation of the left side of the body), and one case with a small umbilical hernia and IUGR, in which the cord pathology revealed multiple syncytial knots adjacent to a complex cyst of the cord. Overall, 12 of the 13 newborns survived and the vast majority had a favorable outcome.
- 2.
Different results are reported by Sepulveda et al. [58] on 13 fetuses with umbilical cord cysts detected during the second and third trimesters of pregnancy but with additional sonographic findings diagnosed in 11 cases. A prenatal karyotype testing was performed in ten of these fetuses, detecting aneuploidy in seven of them. In the three cases with normal karyotype, multiple anomalies were found in two fetuses and isolated omphalocele in one fetus. In a further case with isolated omphalocele, karyotyping was not performed. All chromosomally abnormal fetuses and two chromosomally normal fetuses with associated multiple structural defects died in utero or after birth. There were no perinatal complications in the fetuses with isolated cysts and normal karyotype. Fetuses with omphalocele were born without other defects, and the omphalocele was repaired. In all cases of chromosomal abnormalities, there were additional anomalies detected by ultrasound scans.
- 3.
Smith et al. [56] reported the outcome of three cases with umbilical cord cysts. One, in which a transient cyst was detected at the end of the first trimester, had a normal outcome, and two, in which the cyst was detected at 23 and 39 weeks of gestation, were diagnosed as having trisomy 18. They reviewed the literature and summarized the outcome of their two, and another 21 cases of persistent second and third trimester umbilical cord cystic masses reported in the literature between 1982 and 1996. Nineteen of these cases were associated with either aneuploidy or congenital anomalies. Karyotype testing was performed in 15 cases and found to be abnormal in 13, including 11 with trisomy 18 and two with trisomy 13. A VATER syndrome was diagnosed in one of the cases with a normal karyotype. Among the 19 cases with anomalies, omphalocele was diagnosed in eight, and in two cases it was the only anomaly (and successfully repaired). Only four of the 23 reported cases had a normal fetal outcome. In two cases umbilical cord cyst was the only finding besides IUGR.
- 4.
Ten cases of umbilical cord cysts of the second or third trimester are presented by Zangen et al. [75] In seven of them, the umbilical cord cyst was the only abnormal finding, and in one case the additional findings were polyhydramnios and suspected IUGR without structural anomalies, despite very careful anatomical screening. In all of these cases, normal neonates were born.
Sepulveda et al. [58] showed a correlation between small multiple cysts and aneuploidy, and Ross et al. [53] showed that the risk of fetal anomalies is increased in the presence of cysts located at the fetal or placental insertions. Yet, from this small cohort of cases, it is difficult, if not impossible, to establish a correlation between the appearance of the cyst and the prognosis.
In conclusion, given the apparent association with lethal chromosomal aneuploidy and/or congenital anomalies, the finding of an isolated umbilical cord cystic mass should lead to further detailed sonographic evaluation in a tertiary center. When either IUGR or other anomalies are found, karyotype testing should be recommended.
8.3.6 Aneurysms and Hemangiomas of the Umbilical Cord
Aneurysms of the umbilical vessels, usually the vein, are described. Elastic stains have shown that the elastic fibers of such veins are focally deficient. Aneurysmal dilatations of umbilical vessels may compress the other vessels or cause a cord rupture or hematoma. Fetal death, neurologic impairment [86–88], and fetal growth restriction have been described as associated with these complications. In addition, aneurysms have been associated with abnormal cord insertion, single umbilical artery, and other miscellaneous placental anomalies [5].
Hemangiomas appear histologically similar to those described elsewhere in the body, and those developing within the cord may coexist with similar lesions in the fetus [89]. They are more common at the placental insertion of the cord, arising from one or more umbilical vessels [90]. Prenatal diagnosis may sometimes be performed by sonographic evaluation. These tumors are never malignant, but fetal death is frequent if the tumor is large [41].
The adverse outcomes are mostly dependent on rupture and secondary hemorrhage or formation of a hematoma. Hemangiomas up to 18 cm in length, 14 cm in diameter, and weighing up to 900 g have been described [5]. In some of them, myxoma-like Wharton’s jelly is present within the tumor [86], and in these cases, the tumor is designated as angiomyxoma. Unlike chorioangiomas within the placental parenchyma, angiomas of the cord are usually not associated with hydramnios or hydrops. Of interest, elevation in maternal serum alpha-fetoprotein may be observed.
8.3.7 Abnormal Cord Coiling
Since the origin of umbilical cord coiling (Fig. 8.12) is suggested to be, at least in part, the result of fetal rotation and activity, the lack of coiling may partially reflect fetal inactivity or possible neurologic abnormalities [91], whereas marked coiling may reflect fetal hyperactivity [5]. Absent or minimal coiling is uncommon, but, when present, it may be associated with fetal distress, fetal anomalies, chromosomal errors, and increased fetal and perinatal mortality [7, 91–93]. Similarly, excessive coiling has been associated with preterm labor, fetal demise, low umbilical arterial pH, fetal asphyxia, and chronic fetal hypoxia [7, 91–93]. Excessive coiling is also seen more frequently in cords with constrictions and those of excessive length, both of which are also associated with adverse outcome [7, 94, 95]. Regardless of the etiology, excessive coiling has the potential for obstruction of blood flow through the umbilical vessels. As with other types of mechanical obstruction, the presence of associated thrombosis in the umbilical vessels and the fetal circulation may reflect lesions affecting neonatal outcome.


Fig. 8.12
Umbilical cord coiling, as the result of excessive fetal rotation and activity
8.3.8 Anomalies of Cord Diameter and Length
8.3.8.1 Lean Umbilical Cord and IUGR
It has been shown that a lean umbilical cord on prenatal sonography poses a risk for small for gestational age at delivery and distress during labor [96]. Moreover, it is reported that umbilical cords of women with early onset preeclampsia and otherwise appropriate fetal growth are more likely to be lean and to have a smaller size in umbilical vein than those of women with an uneventful pregnancy course [97]. In addition, lean umbilical cords with reduced vein caliber are also described in IUGR fetuses with normal umbilical artery Doppler [98, 99].
These findings are confirmed by pathology [100], since computerized microscopic morphometric analysis showed that umbilical cords of IUGR fetuses were significantly smaller and characterized by reduced umbilical vein cross-sectional areas compared with those of healthy fetuses. Inan et al. [101] reported similar findings in umbilical cords of pregnant women with hypertensive disorders. Of interest, biochemical studies showed that, in women with preeclampsia, Wharton’s jelly has a high content of sulfated glycosaminoglycans and type III collagen, whereas hyaluronic acid is reduced when compared with that of healthy women [102]. Hyaluronic acid is highly hydrophilic, and its concentration influences the amount of Wharton’s jelly, and this is particularly important for the mechanical properties and macroscopic appearance of the cord and may explain, at least in part, the distress developed in some of these fetuses.
Raio et al. [103] assessed the relationship between sonographic morphometric changes of different umbilical cord components (umbilical cord cross-sectional area, vein area, artery area, and Wharton’s jelly area) and umbilical artery Doppler abnormalities in IUGR fetuses. They studied 84 intrauterine growth-restricted fetuses and 168 appropriate for gestational age fetuses. All umbilical cord components were reduced in the growth-restricted fetuses. Specifically, the rate of lean umbilical cords, defined as a cross-sectional area <10th percentile for gestational age, was significantly higher in growth-restricted fetuses compared with those appropriate for gestational age (73.8 % versus 11.3 %; P < 0.0001). A significant and progressive reduction of the umbilical vein area corresponding to the worsening of umbilical artery Doppler abnormality was found. The umbilical artery area was not related to the hemodynamic changes of the blood flow in the umbilical arteries.
In addition, several anatomic studies investigated the umbilical cord structure in the presence of fetal IUGR [100] and hypertensive disorders [100, 101]. Bruch et al. [100] reported that umbilical cords of IUGR fetuses with normal umbilical artery Doppler parameters were characterized by a reduction of both the total vessel area and the Wharton’s jelly area in comparison with healthy fetuses. When IUGR fetuses with normal umbilical artery Doppler parameters were compared to those with abnormal Doppler parameters, a further decrease of the total vessel area was observed, which was mainly due to a reduction of the vessel wall thickness. These findings are in agreement with those reported by Inan et al. [101]. However, a limitation of pathologic studies is that the umbilical cords are analyzed after fixation. Significant variations of its structure may be consequences of physiologic changes after delivery or may be due to the fixation itself [104, 105]. A possible explanation for the reduced umbilical vessel area seen in IUGR fetuses might be vasoconstriction of the umbilical vessels, mediated by an altered function of locally acting factors. Because human umbilical cord vessels are unique in lacking innervation, the action of vasoactive substances might be crucial in the control of their tone [103].
Of interest, structural changes observed in trophoblasts and blood vessels in placentas complicated by fetal growth restriction have been explained as the result of an altered balance of vasoactive substances, which, in turn, downregulate the production of nitric oxide, a potent vasodilative agent [106–108]. Indeed, nitric oxide has been found not only within the placenta but also in the human umbilical cord vessels and, in particular, in the umbilical vein endothelial cells [109]. This may explain the histologic findings of vasoconstricted umbilical cord vessels observed in IUGR fetuses with normal umbilical artery Doppler parameters [100, 101].
8.3.8.2 Large Cross-Sectional Study of the Umbilical Cord: An Indirect Sign of Fetal Pathology?
Ultrasound examinations are commonly requested when a large fetus is suspected, and these findings are likely to influence obstetric management [110] despite recommendations against changes to medical treatment based on estimated fetal weight. Moreover, several protocols, including the ACOG guidelines [111], use estimated fetal weight as a basis for clinical decision-making, and such recommendations have become standards of care that are difficult to ignore in the current medicolegal scenario.
Unlike ultrasound measurement of conventional biometric parameters that can be technically difficult late in gestation due to the low position of the fetal head, abdominal circumference distortion, and posterior position of the femora, a successful assessment of umbilical cord area seems not to be influenced by gestational age or amniotic fluid volume [112].
Cromi et al. [112] studied consecutive patients >34 weeks’ gestation, who presented for sonographic examination and delivered within 4 weeks. These authors analyzed the cross-sectional areas of the umbilical cord, umbilical vessels, and Wharton’s jelly, measured in a free loop of the umbilical cord. In addition, they performed a logistic regression analysis to determine significant predictors of macrosomia (actual birth weight >4,000 and >4,500 g). Fetal biometric parameters (biparietal diameter, abdominal circumference, and femur length), sonographic estimated fetal weight, and umbilical cord area >95th percentile for gestational age were used as covariates. They found that, if a cross-sectional area of the umbilical cord >95th centile was detected at ultrasound, only 25 % of newborns actually weighed more than 4,000 g, concluding that a large cross-sectional area of the umbilical cord by itself poorly performs as a predictor of fetal macrosomia.
However, by combining this parameter with an abdominal circumference >95th percentile, 100 % of neonates predicted to be macrosomic were confirmed at birth. The new formula incorporating the umbilical cord area to predict fetal weight appeared to perform marginally better than did the Hadlock formula, although without reaching statistical significance. The authors suggested the possibility that this equation may result in a significant improvement in the prediction of fetal weight in more selected groups or with a larger number of patients [112].
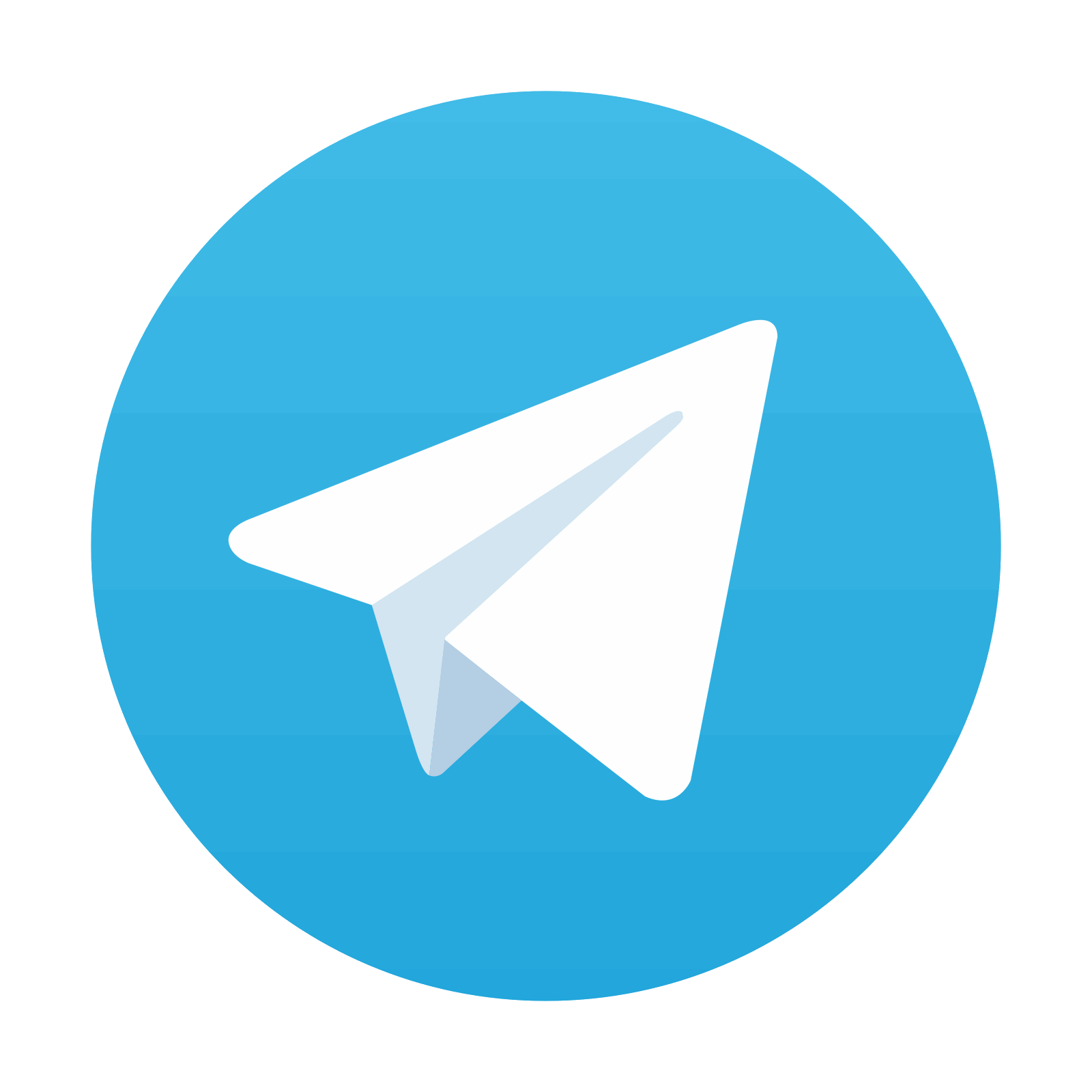
Stay updated, free articles. Join our Telegram channel

Full access? Get Clinical Tree
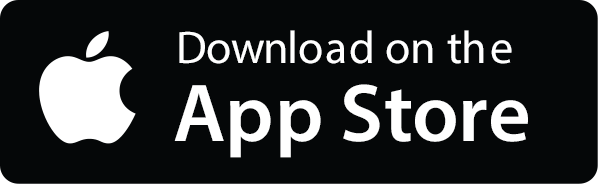
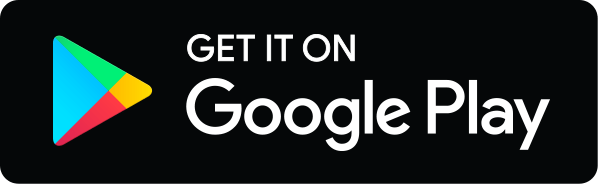