Objective
This study aimed to present a narrative review regarding androgen production, androgens’ role in folliculogenesis, and the available therapeutic approaches for androgen supplementation, and to perform a systematic review and meta-analysis regarding the impact of androgens (dehydroepiandrosterone/testosterone) compared with placebo or no treatment on ovarian response and pregnancy outcomes in patients with diminished ovarian reserve and/or poor ovarian responders.
Data Sources
An electronic search of MEDLINE, Embase, Cochrane Library, Cochrane Central Register of Controlled Trials, Scopus, ClinicalTrials.gov , the ISRCTN registry, and the World Health Organization International Clinical Trials Registry, was conducted for studies published until September 2021.
Study Eligibility Criteria
Randomized controlled trials that compared ovarian response and/or pregnancy outcomes between the different in vitro fertilization protocols using androgens (ie, dehydroepiandrosterone and testosterone) and conventional in vitro fertilization stimulation in patients with diminished ovarian reserve and/or poor ovarian responders were included.
Methods
The quality of each study was evaluated with the revised Cochrane risk-of-bias tool for randomized trials (RoB 2). The meta-analysis used random-effects models. All results were interpreted on the basis of intention-to-treat analysis (defined as the inclusion of all randomized patients in the denominator). Risk ratios and 95% confidence intervals were used and combined for meta-analysis.
Results
No significant differences were found regarding the number of oocytes retrieved (mean difference, 0.76; 95% confidence interval, −0.35 to 1.88), mature oocytes retrieved (mean difference, 0.25; 95% confidence interval, −0.27 to 0.76), clinical pregnancy rate (risk ratio, 1.17; 95% confidence interval, 0.87–1.57), live-birth rate (risk ratio, 0.97; 95% confidence interval, 0.47–2.01), or miscarriage rate (risk ratio, 0.80; 95% confidence interval, 0.29–2.22) when dehydroepiandrosterone priming was compared with placebo or no treatment. Testosterone pretreatment yielded a higher number of oocytes retrieved (mean difference, 0.94; 95% confidence interval, 0.46–1.42), a higher clinical pregnancy rate (risk ratio, 2.07; 95% confidence interval, 1.33–3.20), and higher live-birth rate (risk ratio, 2.09; 95% confidence interval, 1.11–3.95).
Conclusion
Although dehydroepiandrosterone did not present a clear effect on outcomes of assisted reproductive techniques, we found a potentially beneficial effect of testosterone priming on ovarian response and pregnancy outcomes. However, results should be interpreted with caution, taking into account the low to moderate quality of the available evidence.
Why was this study conducted?
To evaluate the effect of dehydroepiandrosterone and testosterone priming on ovarian response and on the reproductive outcomes of patients with diminished ovarian reserve or poor ovarian response.
Key findings
Although dehydroepiandrosterone did not present a clear effect on outcomes of assisted reproduction techniques, we found a potentially beneficial effect of testosterone priming on ovarian response and pregnancy outcomes. However, results should be interpreted with caution, taking into account the low to moderate quality of the available evidence.
What does this add to what is known?
This study presents the available evidence on the use of androgens in diminished ovarian reserve and poor ovarian response patients in a blended format. First, we describe the therapeutic rationale based on animal and human studies. Secondly, we perform a systematic review and meta-analysis to analyze the effect of androgen pretreatment on in vitro fertilization outcomes. Our results provide valuable information for clinical guidelines and future research.
Introduction
The primary aim of controlled ovarian stimulation (COS) is to maximize ovarian response and, therefore, the number of embryos obtained, to select the best-quality embryos for transfer and improve the chances of conception. Previous studies have shown that, the higher the number of oocytes retrieved, the higher the cumulative live-birth rate, which is the most reliable outcome measure in assisted reproductive techniques (ARTs). Patients with diminished ovarian reserve (DOR) and/or poor ovarian responders (PORs) present consistently low live-birth rates (LBRs), irrespective of the protocol of ovarian stimulation used. , In this regard, the management of DOR and/or POR patients remains 1 of the most challenging tasks in ART, and numerous adjuvant strategies have been proposed to improve ovarian response and increase pregnancy outcomes with variable levels of evidence.
Systemic administration of androgens has been proposed as 1 of these adjuvant approaches, aiming to improve follicular recruitment and, therefore, ovarian response to COS. However, despite the promising results behind its rationale, the clinical outcomes remain inconsistent.
Objectives
The focus of this review is two-fold. The first objective is to provide a narrative review regarding androgen metabolism, androgens’ role in folliculogenesis, and the available therapeutic approaches for androgen supplementation. Secondly, we aim to present a systematic review and meta-analysis regarding the impact of systemic androgen supplementation (dehydroepiandrosterone [DHEA] or testosterone [T]) on ovarian response (number of oocytes and mature oocytes retrieved) and pregnancy outcomes (clinical pregnancy rate, live-birth rate, and miscarriage rate [MR]) among infertile women characterized as poor responders (poor ovarian reserve or response).
Methods
Considering the complexity of the subject and the fact that the topics covered by the narrative review were not amenable to be addressed with the PICO strategy (Patients, Intervention, Comparison, and Outcomes), we adopted a combined format. Therefore, we firstly performed a narrative review and then a systematic review, with meta-analysis where appropriate.
Narrative review
For the narrative review, we searched PubMed for both original articles and review articles. The Medical Subject Headings (MeSH) terms “Testosterone”[MeSH], “Dehydroepiandrosterone”[MeSH], “Androgens/administration and dosage”[MeSH], “Androgens/therapeutic use”[MeSH], and “Fertilization in Vitro”[MeSH] were explored until September 2021.
Systematic review and meta-analysis
A full-length description of the systematic review and meta-analysis methods is available in the Supplemental Methods .
Eligibility Criteria
The systematic review and meta-analysis were registered in the International Prospective Register of Systematic Reviews database (CRD42021285328) and reported according to the Preferred Reporting Items for Systematic Reviews and Meta-Analyses (PRISMA) guidelines.
We used the PICO strategy to structure the research questions for the systematic review. We included randomized controlled trials that compared the selected reproductive outcomes between the different in vitro fertilization (IVF) protocols using androgens (ie, DHEA and T) (intervention) and those using conventional IVF stimulation (comparison) among subfertile women characterized as poor responders (poor ovarian reserve or response) undergoing ovarian stimulation in IVF/intracytoplasmic sperm injection (ICSI) treatment (patients). For the meta-analysis, the primary outcome was LBR, defined as the ratio between the number of deliveries resulting in at least 1 live birth per woman randomized (ie, intention-to-treat). Secondary outcomes included clinical pregnancy rates (CPRs), MR, number of oocytes retrieved, and number of mature metaphase II (MII) oocytes retrieved. All the PICO definitions (ie, outcomes, and patients) adhered to the International Committee Monitoring Assisted Reproductive Technologies/World Health Organization glossary.
Information Sources and Search Strategy
The following electronic databases were used: MEDLINE (OvidSP, 1956 to date), Embase (OvidSP, 1982 to date), the Cochrane Library (Cochrane Database of Systematic Reviews; OvidSP), the Cochrane Central Register of Controlled Trials (OvidSP), Scopus, ClinicalTrials.gov , the ISRCTN registry ( www.controlled-trials.com ), and the World Health Organization International Clinical Trials Registry Platform search portal ( http://apps.who.int/trialsearch/Default.aspx ) to identify all relevant studies published until September 2021.
- 1.
Combinations of the following keywords and MeSH search terms were used: “Testosterone” [MeSH] OR (testosteron∗) OR (DHEA) OR “Dehydroepiandrosterone” [MeSh], poor OR low OR slow OR inadequate OR suboptimal OR decreas∗ OR diminish∗ AND respon∗ OR ovarian reserve, poor ovarian reserve, poor responders, poor respon∗, GnRH analog∗, GnRH agonist, gonadotropins, live birth rate, pregnancy rate, number of oocytes “AND” “Fertilization in Vitro”[MeSH] OR “IVF” OR “in vitro fertilization” OR “Sperm Injections, Intracytoplasmic”[MeSH] OR “ICSI” OR “Reproductive Techniques, Assisted”[MeSH] OR “Assisted Reproductive Technology” “AND” random∗ controlled trial(s) ( Supplemental section on “Search strategy for identification of studies”). We did not adopt any language restrictions. We examined the reference list of all identified primary studies, published systematic reviews and meta-analyses, abstracts of major conference meetings (ie, European Society of Human Reproduction and Embryology and American Society for Reproductive Medicine), and the references from all included studies to identify further eligible studies.
Study Selection
First, all duplicates were removed. Secondly, all citations were screened by title and abstract by 2 authors (A.R.N. and P.M.B.). The discrepancies were solved by discussion and, if needed, in consensus with a third author (N.P.P.). Next, 2 authors (A.R.N. and P.M.B.) determined the randomized controlled trials’ (RCTs) study eligibility. We documented the study selection process in a PRISMA flow diagram ( Supplemental Figure 1 ).
Data Extraction
Using a standardized data extraction format, 2 authors (A.R.N. and P.M.B.) independently extracted relevant data from each selected study, including data referring to the study (country, journal, year of publication), participant characteristics, methodological characteristics (blinding, randomization, allocation concealment, etc.), sample characteristics, description of experimental and control interventions, results and data on quality assessment, and investigation of heterogeneity. The numeric information was cross-tabulated in spreadsheet software, and the results showed in forest plots.
Assessment of Risk of Bias
We employed the Grading of Recommendations Assessment, Development and Evaluation (GRADE) criteria (risk of bias, inconsistency of the effect, indirectness, imprecision, and publication bias) to assess evidence quality with GRADEpro GDT (Guideline Development Tool) ( Supplemental Table ). We evaluated overall evidence quality for each outcome included in the meta-analysis (LBR, CPR, MR, total number of oocytes and MII oocytes retrieved.) Two authors (P.M.B. and A.R.N.) independently made judgments about evidence quality (high, moderate, low, or very low). In the case of disagreement, a third review author (N.P.P.) was consulted to establish a consensus.
Data Synthesis
Quantitative analysis
All results were interpreted on the basis of intention-to-treat analysis (defined as the inclusion of all randomized patients in the denominator). Risk ratios (RRs) and 95% confidence intervals (CIs) were used and combined for meta-analysis. We pooled data related to the dichotomous outcomes to determine the RRs with corresponding 95% CIs. Regarding the number of oocytes and MII oocytes (continuous outcomes), the data were pooled using the inverse variance model and mean difference (MD) with 95% CI to determine effect size. A meta-analysis was conducted where appropriate, using the random-effects model. We assessed I 2 by detecting study-to-study variation, and a value >50% indicated significant heterogeneity. Statistical significance was set at P <.05. Statistical analysis was performed using the R language for statistical computing (R Core Team, Vienna, Austria), RStudio (Rstudio, Boston, MA), and the packages “metafor,” “meta,” “ggplot2,” “dmetar,” and “robvis.”
Multiple sensitivity analyses and an influence analysis were performed to assess the strength of obtained conclusions and detect any possible outliers. The analysis also showed whether the same results would be obtained if using the odds ratio (OR) instead of RR, the fixed-effects model instead of the random-effects model, or if we detected high risk of bias (in any domain). Finally, we used prediction intervals to better interpret heterogeneity and estimate true treatment effects.
Qualitative analysis
We evaluated the methodological quality and risk of bias of the studies included in this meta-analysis using the revised Cochrane risk-of-bias tool for randomized trials (RoB 2) recommended by the Cochrane Handbook for Systematic Reviews of Interventions ( Supplemental Figures 2 and 3 ). Two authors (P.M.B. and A.R.N.) assessed each study’s risk of bias in an independent and duplicate fashion, and a third author (N.P.P.) resolved conflicts. Finally, we assessed publication bias using funnel plots ( Supplemental Figure 4 ) and P -curves as the best ways for visualizing whether small studies with small effect sizes were missing from the systematic search. ,
Narrative Review
Androgen production in women
Androgen production in women originates mainly from the adrenal gland and the ovaries ( Figure 1 ). Peripheral tissues, such as the liver, the skin, or the adipose tissue, provide an accessory contribution by converting circulating dehydroepiandrosterone sulfate (DHEA-S).

Androgen production in the adrenal gland is under the influence of the hypothalamic secretion of corticotropin-releasing hormone and the anterior pituitary secretion of adrenocorticotropic hormone and, under normal circumstances, is lower than the gonadal production. Within the ovary, steroidogenesis is compartmentalized according to the 2-cell–2-gonadotropin theory, with DHEA, androstenedione (A4), and T being synthesized in the theca cells, under the control of luteinizing hormone (LH), before diffusing into the granulosa cells (GC) and being converted into dihydrotestosterone (DHT) or aromatized into estrone and estradiol, under the influence of follicle-stimulating hormone (FSH) ( Figure 1 ).
The major circulating androgens in normoandrogenic women, in descending order of serum concentrations and in ascending order of relative potency, are DHEA-S, DHEA, A4, T, and DHT. , Their bioavailability and metabolic clearance rate are mainly dependent on their degree of binding to the main circulating binding proteins: the low-capacity but high-affinity sex hormone-binding globulin and the high-capacity but low-affinity albumin. The Table summarizes androgen production and the bioavailability of the main circulating androgens.
Androgen | Production | Serum concentrations | Menstrual cycle variation | Transport | |||
---|---|---|---|---|---|---|---|
Premenopausal | Postmenopausal | Free | Albumin | SHBG | |||
DHEA-S | 0% ovary 90% adrenal 10% peripheral | 0% ovary 90% adrenal 10% peripheral | 1000–4000 ng/mL | Relatively constant levels | 5% | 95% | — |
DHEA | 10% Ovary 50% Adrenal 40% Peripheral | 10% Ovary 50% Adrenal 40% Peripheral | 1–10 ng/mL | Relatively constant levels | 3.93% | 88.1% | 7.88% |
Androstenedione | 40% Ovary 50% Adrenal 10% Peripheral | 20% Ovary 70% Adrenal 10% Peripheral | 0.5–2 ng/mL | Peaks in midcycle | 7.54% | 84.5% | 6.6% |
Testosterone | 25% Ovary 25% Adrenal 50% Peripheral | 50% Ovary 10% Adrenal 40% Peripheral | 0.2–0.7 ng/mL | Peaks in midcycle | 1.4% | 30.4% | 66% |
DHT | Almost exclusively peripheral from T | Almost exclusively peripheral from T | 0.02 ng/mL | Relatively constant levels | 0.5% | 21.0% | 78% |
Common to all androgens in women is the decline in serum levels with age after a peak between 20 to 30 years, with a steeper decline in early reproductive years and, with the exception of DHEA-S, a tendency for a small increase in later decades.
Androgen action in folliculogenesis
Androgens exert their effects both through a direct and indirect pathway. The direct pathway is via the androgen receptor (AR), a member of the steroid/thyroid hormone nuclear receptor superfamily encoded by an X chromosomal gene. The indirect pathway results from the conversion into estrogens or 3β-androstenediol and activation of the estrogen receptor (ER) ( Figure 2 ). Importantly, only T and DHT are bioactive forms, binding directly to the AR, whereas the other androgens are precursors that require conversion into the bioactive hormones to exert their effect.

Animal studies
Hyperandrogenism, either from intraovarian origin, such as polycystic ovarian syndrome, or extraovarian origin, such as congenital adrenal hyperplasia or androgen-producing tumors, results in an increased number of developing follicles and an enlarged ovarian stroma. These observations were the starting point for the initial in vivo studies in primates showing that T and DHT treatment stimulated primordial follicle initiation and increased the number of growing small preantral and antral follicles and overall follicle survival. , Since then, several animal studies have followed, confirming the importance of T in the transition from the primordial to the primary follicle stage and from the preantral to antral stage. , Moreover, the importance of androgen support for overall follicle survival by decreasing follicle atresia and GC apoptosis and stimulating GC proliferation and differentiation has also been established.
The interaction between the FSH receptor (FSHR) and AR might explain some of these actions. In fact, studies in primates have shown that FSHR and AR messenger RNA (mRNA) levels in GC are positively correlated. Furthermore, T treatment has been shown to increase FSHR expression at all levels of follicular development, whereas FSH treatment increased AR expression in primary follicles. , Likewise, treatment with DHT in gilts has been shown to increase FSHR mRNA in preovulatory follicles, and studies in mice revealed that androgens increase FSHR protein levels, increasing follicular sensitivity to FSH and promoting preantral follicle growth and progression to antral follicles ( Figure 3 ).

Androgen receptor knockout (ARKO) studies
Despite the valuable findings provided by these experiments, they preclude the assessment of androgen action exerted directly through the AR because of the indirect effect exerted through ER activation ( Figure 2 ). Thus, genetic models with female mice homozygous for an inactivated AR (ARKO) have been developed to determine the direct action of AR on ovarian function. Based on these experiments, global ARKO female mice have demonstrated defective folliculogenesis, with a lower number of preantral follicles, higher rates of GC apoptosis, premature ovarian failure, lower response to COS, a defective luteal phase, and reduced litter size. Moreover, controls present normal cycles and fertility when ARKO and control ovaries are cross-transplanted, whereas ARKO hosts present abnormal cycles and reduced fertility, further suggesting an extraovarian neuroendocrine AR-mediated effect. Cell-specific ARKO models have also demonstrated that AR signaling in GCs is necessary for progression beyond the preantral stage, and AR importance in the pituitary signaling for a proper regulation of LH/FSH expression and secretion.
Human studies
In women, the AR is expressed at all levels of the female hypothalamic-pituitary-gonadal axis. Within the ovaries, the AR is expressed in the oocyte, GC, and theca cells, and in the ovarian stroma, and it is present in most stages of follicular development, although with different patterns of expression at different developmental stages. AR expression has not been detected in primordial follicles, but from the primary follicle stage onwards, and correlates linearly with follicle growth. Interestingly, the finding that early follicles acquire AR before FSHR and that AR expression in preantral follicles is higher than that of FSHR also points toward a role for androgens in early folliculogenesis. In line with these findings, data obtained from small antral follicles revealed a positive correlation between FSHR gene expression and AR expression on GC, and between the expression of FSHR and intrafollicular androgen (ie, A4 and T) concentrations, suggesting that androgens might have a role in the promotion of gonadotropin responsiveness of developing follicles.
To summarize, the available evidence seems to point toward a role for androgens in early folliculogenesis, maintenance of follicle health, and priming of later stages of follicle development.
The rationale for the use of androgens in DOR patients
Circulating levels of androgens have been reported to decline steeply with age. This phenomenon has been proposed to contribute to the reduced ovarian response to COS associated with ovarian aging and has been the rationale for androgen supplementation in patients with DOR and/or POR.
Taking into account the importance of androgens in the early steps of folliculogenesis, different clinical approaches have been proposed to increase intraovarian androgen availability: systemic administration of androgens (DHEA or T); administration of drugs with LH activity (recombinant LH, human chorionic gonadotropin) to increase thecal androgen synthesis , ; and administration of aromatase inhibitors, preventing the conversion of androgens to estrogens, and, therefore, increasing intraovarian androgen availability. , In this review, we will focus on the systemic administration of androgens.
Which androgen?
Vendola et al conducted the initial studies in primates that drove the scientific community’s attention to the potential role of androgen supplementation in increasing folliculogenesis. Although these initial studies were performed with the bioactive androgens T and DHT, the clinical role of androgens in ART was first proposed by Casson et al, who suggested that DHEA might augment ovarian response in women with previous POR.
DHEA is a preandrogen with a low affinity for the AR, requiring further conversion into T and DHT to exert its effect in the AR. A recent RCT has shown that DHEA supplementation significantly increased serum T and DHEA-S levels starting from week 4 of treatment. However, the fact that no difference was reported in follicular T levels questions the potential effect of DHEA on follicle development. Conversely, T, which is 1 of the most potent androgens in women, binds directly to the AR. Transdermal T supplementation has been shown to result in an increased expression of the AR gene in cumulus cells, supporting a role for T pretreatment at the follicular level.
Dose and duration of treatment
Multiple studies on DHEA supplementation have been published reporting the use of oral micronized DHEA 50 to 90 mg daily, with treatment durations ranging from 4 weeks to 12 months. However, no dose-finding studies have been performed to determine the optimal dose and duration for DHEA pretreatment. Although previous RCTs have reported a higher antral follicle count and ovarian volume after 16 weeks of DHEA supplementation in women with premature ovarian insufficiency, and a higher intrafollicular DHEA-S concentration after 12 weeks of DHEA pretreatment, these studies were not designed to determine the optimal duration of treatment. ,
Most studies regarding T treatment in DOR and POR patients have reported transdermal application, with dosages varying from a 2.5 mg per day patch to a 10 to 25 mg per day gel application, and a duration of treatment between 5 and 28 days before the beginning of COS. One exception was the study by Saharkhiz et al, in which transdermal T supplementation was started with COS and maintained until the day of trigger.
Most of the available studies used a hydroalcoholic gel containing 1% or 2% T. , After the first application, the serum T levels reach a steady state in 24 hours and remain so for the duration of gel application, with parallel increases in DHT and estradiol. T concentrations begin to decline 24 to 36 hours after gel application, justifying a once-daily dosage, and return to baseline levels within 4 days after stopping application. Other authors used a patch with a 2.5 mg per day nominal delivery rate of T, which was applied on the thigh at night and maintained for 12 hours. , This transdermal delivery system also maintains stable T levels with little intra- or interindividual variation.
Either with patch or gel application, doses of treatment have been based on Vendola’s studies on primates, reporting an effect on follicular development with 20 μg/Kg/day. , However, pharmacokinetic studies performed in postmenopausal women revealed that the administration of 4.4 to 5 mg T gel raised free T levels to the normal range for reproductive-aged women, whereas doses above 8.8 to 10 mg increased T levels above the physiological range, questioning the doses used in these previously mentioned studies. , In line with these findings, studies in female-to-male transgender patients have reported a significant suppression in anti-müllerian hormone serum levels after high-dose T pretreatment. Moreover, studies in mice have shown that supraphysiological T levels negatively affect meiosis resumption, further highlighting a potential detrimental effect of supraphysiological doses on ovarian reserve.
Regarding T treatment duration, some authors have adopted a 5-day pretreatment based on the previously mentioned primate studies. , , , However, Kim et al have shown that T effects at the follicular level occurred after at least 3 weeks of T pretreatment. Therefore, most studies have reported a 21- to 28-day pretreatment. Considering that the time that elapses in progressing from a primary follicle to ovulation is approximately 85 days, the beneficial effect of longer pretreatment durations cannot be disregarded.
In summary, there is limited evidence regarding the dose and duration of T or DHEA required and the intraovarian androgen availability after systemic administration at nonvirilizing concentrations.
Side effects
A few side effects including hair loss, hirsutism, and voice deepening have been reported in women undergoing DHEA treatment, but they are negligible in most studies with 75 mg; acne and oily skin are the most frequently reported. , Wong et al reported 2 cases of asymptomatic transient polycythemia. Karp et al described a case report of a first generalized seizure episode in a 30-year-old patient with DOR and a previous brain lesion after a car accident, undergoing pretreatment with 75 mg of oral DHEA daily. The authors underlined that special attention should be given to women with risk factors for convulsive activity, taking into account that DHEA crosses the blood–brain barrier and affects central nervous system excitability.
Regarding transdermal T, Singh et al reported few cases of acne, oily skin, increased hair growth, breast tenderness, vaginal spotting, and mild leg swelling. However, no local or systemic side effects related to the use of transdermal T patches or gel have been reported in most studies. , , , ,
Systematic Review
Effect of androgens on ovarian response to controlled ovarian stimulation
Oocyte yield
The impact of androgen pretreatment on the number of oocytes retrieved was compared among 12 RCTs ( Figure 4 , A). No significant difference was found after DHEA pretreatment (MD, 0.76; 95% CI, −0.35 to 1.88; 5 RCTs; 538 patients; I 2 =77%; random-effects model; moderate certainty). Conversely, a significantly higher number of oocytes was obtained after T pretreatment (MD, 0.94; 95% CI, 0.46–1.42; 7 RCTs; 603 patients; I 2 =42%, random-effects model; moderate certainty).

No significant effect on the number of MII oocytes retrieved was observed after DHEA pretreatment (MD, 0.25; 95% CI, −0.27 to 0.76; 3 RCTs; 372 patients; I 2 =19%; random-effects model; moderate certainty) nor after T priming (MD, 0.62; 95% CI, 0.00–1.25; 6 RCTs; 553 patients; I 2 =71%; random-effects model; moderate certainty) ( Figure 4 , B).
Embryo yield and embryo quality
Limited evidence exists regarding the effect of androgen priming on the number of embryos obtained. Only 2 RCTs evaluated the impact of DHEA supplementation on the total number of embryos. The authors found no statistically significant difference when comparing DHEA with the control group (respectively, median 2.5 [range 0–7] and median 2 [range 0–7], P =.297). Four RCTs evaluated the effect of T pretreatment on embryo yield. Although 3 did not report a significant effect, , , Saharkhiz et al reported a significantly higher number of embryos after T supplementation. Taking into account the heterogeneity regarding the embryo stage (cleavage stage vs blastocyst), no meta-analysis was performed.
Similarly, although no meta-analysis could be performed regarding embryo quality because of the different grading systems, the impact of androgen pretreatment on embryo quality has also been analyzed in several RCTs.
The effect of DHEA priming on embryo quality has been evaluated in 5 RCTs. Although some reported improved embryo quality, others reported no difference , , or even a lower embryo score after DHEA pretreatment. Notably, despite finding no statistically significant difference in the number of top-quality embryos after 12 weeks of DHEA pretreatment, Yeung et al reported significantly improved embryo quality in the subgroup of women who had higher intrafollicular DHEA-S levels. The authors hypothesized that higher intraovarian DHEA-S concentration, either natural or achieved through DHEA supplementation, might improve follicular maturation, leading to reduced aneuploidy and better-quality embryos.
The effect of T pretreatment on embryo quality has also been a matter of debate. Although some RCTs found no difference in the rate of top-quality embryos transferred, , others found a higher embryo score , , after T priming.
Effect of androgens on clinical outcomes
Pregnancy rate and live-birth rate
The impact of androgen priming on reproductive outcomes is displayed in Figure 5 . Overall, pooled data showed a similar CPR after DHEA priming in DOR/POR patients undergoing IVF (RR, 1.17; 95% CI, 0.87–1.57; 8 RCTs; n=727; I 2 = 0%; low certainty), and a similar LBR (RR, 0.97; 95% CI, 0.29–3.19; 3 RCTs; n=117; I 2 = 0%; low certainty) compared with placebo or no treatment. On the contrary, T pretreatment showed a higher CPR (RR, 2.07; 95% CI, 1.33–3.93; 8 RCTs; n=653; I 2 =0%; low certainty) and higher LBR (RR, 2.09; 95% CI, 1.11–3.95; 4 RCT; n=333; I 2 =0%; low certainty).


Miscarriage rate
The impact of androgen priming on the MR has been evaluated in 10 RCTs. No significant differences were found when DHEA was compared with controls (RR, 0.80; 95% CI, 0.29–2.22; 4 RCTs; n=322; I 2 =0; moderate certainty). Likewise, similar MRs were yielded when T priming was compared with controls (RR, 1.83; 95% CI, 0.58–5.81; 6 RCTs; n=444; I 2 =0; moderate certainty) ( Figure 5 , C).
Comments
Principal findings
Evidence from basic research has indicated a strong potential link between androgens and folliculogenesis. The AR has been shown to have a prominent role in primordial follicle initiation and overall follicle development and survival, and these actions seem to be mediated, to a great extent, by an intricate interaction with the FSHR.
However, despite strong evidence from basic research, available clinical data regarding androgen supplementation in ART are fragmented and heterogeneous. This is mainly because of different study designs, inclusion criteria, doses and durations of treatment, and IVF protocols used. Despite the low quality of the available evidence, a survey published by Andersen et al has shown that more than 40% of physicians in Europe and Australia are prescribing off-label androgens in this subgroup of patients.
Moreover, no studies have been conducted so far to evaluate long-term safety data following off-label androgen supplementation in POR patients undergoing fertility treatments. Consequently, before introducing a routine clinical practice, not only do we need efficacy, but also more solid safety data.
Our meta-analysis evaluated the efficacy of 2 types of androgen pretreatment in improving ovarian response and clinical outcomes in poor responders and yielded different results. Although DHEA did not present a clear effect on improving ART outcomes, we found a potentially beneficial effect of T priming on ovarian response and pregnancy outcomes. However, the results should be interpreted with caution taking into account: (1) the low to moderate quality of the available evidence and (2) the significant heterogeneity in the definition of POR and/or DOR populations and in pretreatment and COS protocols.
Strengths and limitations
A significant strength of the current review is its comprehensive approach, meticulously addressing the pathophysiological role of androgens in female reproduction, and the rigorous systematic review in terms of study selection. Moreover, we reported the prediction intervals for every outcome to better interpret study heterogeneity and estimate the real treatment effects, aiming to facilitate medical advice. The limitations of this review concern the clinical and statistical heterogeneity of the available studies, lacking rigorous criteria for patient selection and treatment protocols, and precluding a clear interpretation of the results. Furthermore, the lack of safety data should be taken into account in future studies.
Comparison with existing literature
A Cochrane meta-analysis analyzed the effect of T pretreatment in POR patients and concluded that pretreatment with T was associated with higher LBRs (OR, 2.60; 95% CI, 1.30–5.20; 4 RCTs; N=345; I 2 = 0%) compared with placebo or no treatment. However, sensitivity analysis removing all studies at high risk of performance bias showed no evidence of a difference between the groups (OR, 2.00; 95% CI, 0.17–23.49; 1 RCT; N=53). The same meta-analysis evaluated the effect of DHEA priming in POR patients and reported an increase in CPR (10 RCTs; OR, 1.44; 95% CI, 1.06–1.94; 1122 women) following DHEA priming compared with placebo or no treatment. Since the publication of this meta-analysis, 4 RCTs have been published regarding the use of T pretreatment in POR patients yielding conflicting results. Although 2 studies have shown a beneficial effect in terms of CPR, , the other 2 reported no difference in terms of ovarian response. , Likewise, 2 RCTs have been published regarding the use of DHEA in POR patients. Although Kotb et al have shown a beneficial effect in terms of reproductive outcomes, Narckwichean et al observed no difference when DHEA priming was compared with placebo. All these RCTs have been incorporated in our meta-analysis.
Conclusions and implications
This review summarizes the available evidence regarding the use of androgens in DOR and/or POR patients and highlights the main weaknesses in the literature, and the need for future evidence-based research. Although our results point toward a potentially beneficial effect of T priming on ovarian response and pregnancy outcomes, these results should be interpreted with caution, taking into account the low to moderate quality of the available evidence. Further properly designed, adequately powered RCTs, including safety data, are needed to clarify the role of androgens in female reproduction.
The T-TRANSPORT trial ( ClinicalTrials.gov Identifier: NCT02418572, available at http://clinicaltrials.gov ) is a currently ongoing multicenter, multinational double-blinded placebo-controlled RCT, including POR women that fulfill the “Bologna criteria.” The trial’s main objective is to assess the effect of transdermal T administration for 2 months before ovarian stimulation on the pregnancy rates of POR patients. Moreover, the study protocol includes additional experiments with cumulus cells and the follicular fluid, aiming to elucidate the mechanism behind the effect of T on folliculogenesis. Hopefully, these highly-awaited results will shed some light on the impact of this approach on POR’s reproductive outcomes.
Supplementary Data
Search strategy for identification of studies
The following electronic databases were searched:
Electronic Database | Results | Status |
---|---|---|
|
489 results, 16 references | Reviewed |
|
289 results, | Reviewed |
|
3 references | Reviewed |
|
91 references | Reviewed |
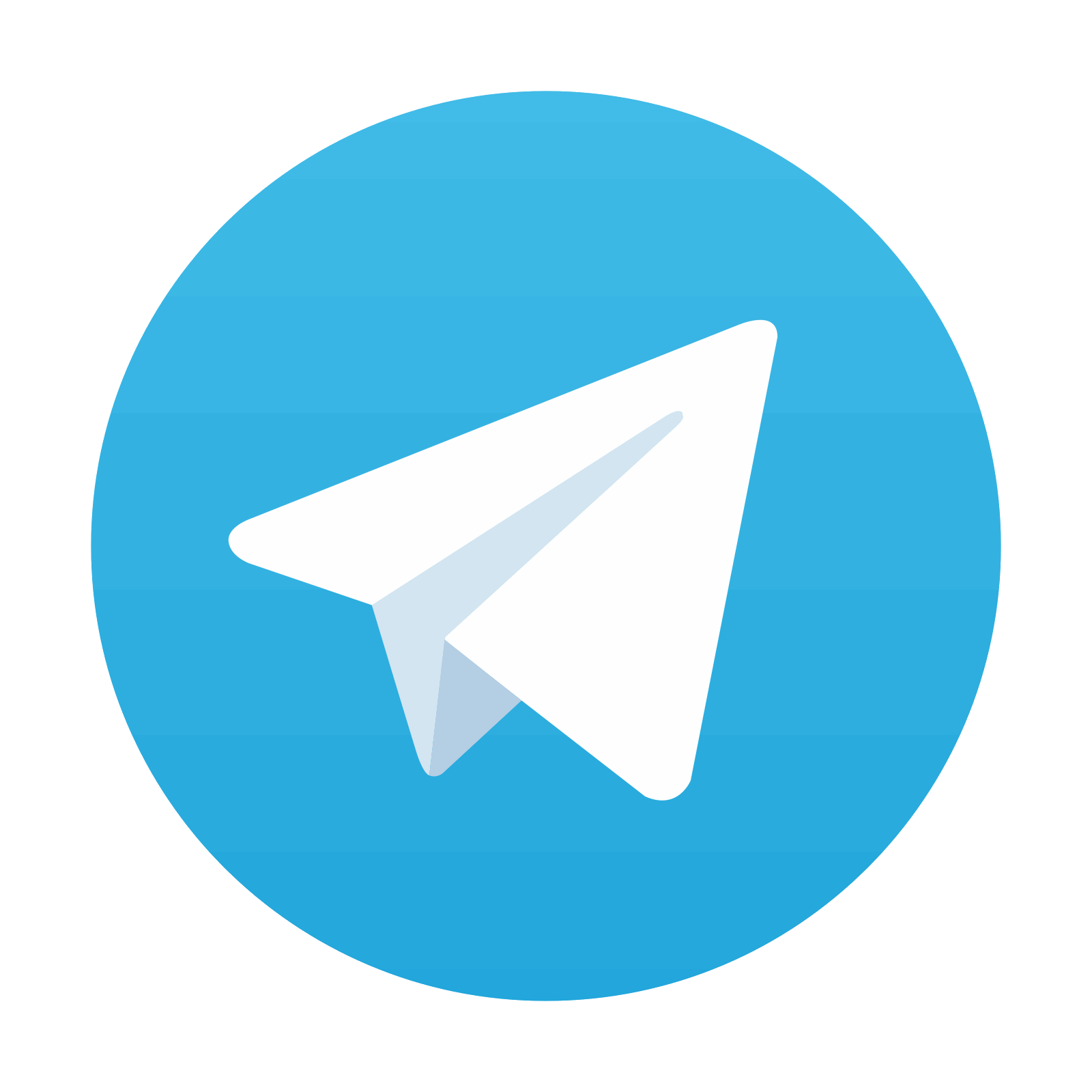
Stay updated, free articles. Join our Telegram channel

Full access? Get Clinical Tree
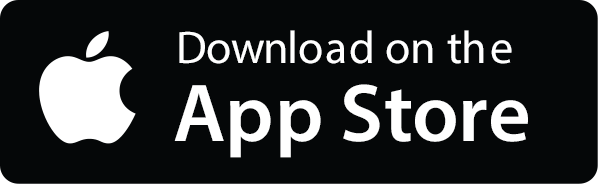
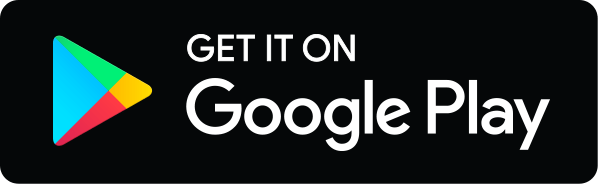