Objective
Obese pregnancy is associated with significantly higher rates of infection, which can harm both mother and fetus. The objective of this study was to determine the impact of obesity on maternal blood immune function.
Study Design
This was a cross-sectional, case control study of 15 obese (Ob) and 15 lean (Lc) subjects. Immune cell subsets, intracellular and serum cytokine production, and lymphocyte proliferation were measured in maternal blood during the second trimester of pregnancy.
Results
Obese women had a significantly lower proportion of CD8+ and NKT cells and a higher proportion of B cells, impaired cytokine production when stimulated ex vivo, and impaired ability of lymphocytes to proliferate compared with their lean counterparts.
Conclusion
Obese pregnancy is associated with impaired cell-mediated immunity. Because perinatal infections can have serious maternal and fetal consequences, it is imperative to better understand these mechanistic underpinnings to optimize prevention and devise targeted therapy.
Obesity affects 1 in 3 women of reproductive age in the United States. Women who are obese prior to becoming pregnant have increased prenatal, perinatal, and neonatal complications. A recent metaanalysis found obese pregnancy to be associated with increased rates of infectious complications such as endometritis, chorioamnionitis, preterm premature rupture of membranes, and delayed postpartum wound healing. These infectious morbidities have serious long-term consequences for both maternal and child health. The mechanism and extent of immune system dysregulation in obese pregnancy has yet to be delineated.
Obesity and pregnancy are each individually associated with immunomodulation. Studies examining the mechanisms linking obesity in nonpregnant adults with immunosuppression have had conflicting results, but all have shown obesity to be associated with some degree of immunosuppression. In a study of 157 nonpregnant female subjects, Nieman et al found obesity to be associated with decreased numbers of total lymphocytes and CD4+ T cells and impaired lymphocyte proliferation. O’Rourke et al compared T lymphocyte subsets in a cohort of 20 obese and lean nonpregnant subjects and found that CD4+ T cells were increased and CD8+ T cells were decreased in obese subjects. Tanaka et al reported a significant impairment in T cell proliferation as well as lower CD4+, CD8+, and CD4+/CD45+RO+ cells in 34 nonpregnant obese subjects compared with 50 controls. The largest study to examine the association between obesity and immune function was conducted longitudinally in 322 nonpregnant women with a median body mass index (BMI) of 29 kg/m 2 . The results of this study, in contrast to previous studies, showed a higher lymphocyte count and increased numbers of CD4+ T and white blood cells with increasing BMI.
Pregnancy also alters immune function. The fetal semiallograft presents paternally derived antigens to the maternal immune system. To allow for successful implantation and growth of the fetus and placenta, the maternal immune system, placenta, and developing fetal immune system must cooperatively facilitate a successful pregnancy. This involves directing natural killer cells, T regulatory cells, dendritic cells, and macrophages from the periphery to the placental decidua to protect the embryo, which alters the balance of the remaining peripheral immune cells.
In addition to changes in immune cell proportions, there is a change in immune cell function to facilitate fetal survival early in a healthy pregnancy. T cell production of interferon (IFN)-γ and tumor necrosis factor (TNF)-α, Th1 cytokines known to endanger fetal survival, is suppressed. As pregnancy progresses, the Th1/Th2 balance is progressively skewed in favor of Th2 cells, which produce interleukin (IL)-4, IL-5, IL-6, IL-9, IL-10, and transforming growth factor (TGF)-β. In addition, levels of progesterone and IL-10, which are known to functionally suppress T cells, are increased in pregnancy and lead to global immunosuppression.
There is early evidence that the immune system in obese pregnancy is further modulated beyond the individual contributions of obesity or pregnancy alone. Challier et al found that macrophage populations were higher in placentae and peripheral blood of obese compared with lean women. Multiple human and animal studies have confirmed that obese pregnancy, similar to the obese nonpregnant state, is associated with increased inflammatory cytokines, both systemically and in adipose tissue.
Given the strong association between maternal obesity, infections, and fetal injury, it is important to better understand the combined impact of these factors on maternal immune function, the key defense mechanism against infections. We hypothesized that obese pregnant women have impaired cell-mediated immune response compared with their lean counterparts.
Materials and Methods
Subjects
We conducted a cross-sectional, case-control study to determine the impact of obesity on maternal immune response. The study protocols, procedures, and consent form were approved by the Tufts Medical Center/Tufts University Institutional Review Board. Subjects were recruited from the Tufts Medical Center Obstetrics clinic between 24 and 28 weeks of pregnancy.
Potential subjects were identified from the prepregnancy BMI noted in their prenatal records. BMI was calculated using the formula: (body weight in kilograms)/(height in meters) 2 . Lean control (Lc) subjects had a prepregnancy BMI of 20-25 kg/m 2 and obese (Ob) subjects had a prepregnancy BMI greater than 30 kg/m 2 . Thirty subjects were recruited for this study, of whom 15 women were obese before pregnancy and 15 control women were of a normal BMI before pregnancy.
Subjects with pregestational diabetes, preeclampsia, and autoimmune disease were excluded from the study. Those who developed complications such as preterm premature rupture of membranes and chorioamnionitis as well as other acute infections such as urinary tract infections or those who were diagnosed with gestational diabetes were excluded from the analysis, given that acute infection or gestational diabetes may alter subjects’ immune response.
Women with multiple gestation (twins or triplets) were not excluded. None of the participants had received antenatal corticosteroids at the time of study recruitment and blood draw. Women who agreed to participate signed informed consent and then completed a questionnaire with details about their medical and social history. Blood was drawn at the time of glucose challenge testing.
Lymphocyte subsets
Lymphocyte subsets were determined by flow cytometry as described previously. Briefly, whole blood cells were placed on ice and stained for 30 minutes with cyanine (Cy 5.5) fluorescein- or phycoerythrin-conjugated monoclonal antibodies (Becton Dickinson, San Jose, CA) to total T cells (anti-CD3), CD4+ cells (anti-CD4), CD8+ cells (anti-CD8), B cells (anti-CD19), natural killer (NK) cells (anti-CD314), naïve cells (anti-CD45RA), and memory cells (anti-CD45RO).
Naïve and memory cells were stained in separate tubes. Flow cytometry was performed on a FACS Calibur flow cytometer (Beckton Dickinson and Co, San Jose, CA). Cells of interest were quantified as a percentage of parent population, as follows: lymphocytes were gated on forward and side-scatter plots; CD3+ and B cells were gated upon total lymphocytes; CD4+, CD8+, and NKT cells were gated on CD3+ cells; naïve and memory cells were gated on the respective parent cells (CD4+ or CD8+).
Intracellular cytokine measurements
The ability of T cells to produce cytokines in response to stimulation with mitogens mimics the ability of immune cells to produce cytokines in response to pathogens. Lymphocyte subsets were stained for intracellular cytokine production of IFNγ and TNFα following stimulation. Briefly, whole blood was diluted 1 to 3.6 with media and stimulated with phorbol myristate acetate (PMA; 40 ng/mL) and ionomycin (1μg/mL) and treated with Brefeldin A (10 μg/mL) to prevent secretion.
After incubation, the cells were spun down and stained for surface antigens (as described in previous text) and then incubated with a conjugated anticytokine monoclonal antibody to IFNγ or TNFα; eBioscience, San Diego, CA). Samples were gated on lymphocytes by using forward- and side-scatter gates, and limits for fluorescence-positive cells were set using nonspecific IgG antibodies. Mean fluorescent intensity (MFI) was used to describe the shift of the intensity of the fluorochrome parameter of interest compared with an isotype control. Flow cytometric data were analyzed using Flow Jo software (Tree Star, Inc, Ashland, OR).
Ex vivo cytokine production
In addition to determining the production of cytokines from particular cells, total secreted production of cytokines, as measured from the cell-free supernatant, was measured as well. Whole blood was incubated in 24 well culture plates with one of the following: (1) lipopolysaccharide (LPS) at 1 μg/L or 10 ng/mL to stimulate IL-6, IL-10, and TNFα or (2) concavalin A (Con A) at 25 or 40 μg/mL to stimulate IL-2, IL-4, IL-17, IFNγ, and TGFβ production. The cell-free supernatant was analyzed for ex vivo production of IL-2, IL-4, IL-17, IFNγ, and TGFβ. Enzyme-linked immunosorbent assay (ELISA) analyses were performed using commercially available kits (eBioscience) according to the manufacturer’s instructions.
Lymphocyte proliferation
The ability of T cells to proliferate in response to mitogenic or anti-CD3/CD28 stimulation is another indicator of immune cell function. Diluted whole blood (1:5 dilution) was seeded into a 96 well plate. The blood was stimulated and incubated with the following mitogens: Con A at 3 different concentrations (10, 50, and 100 μg/mL), Phytohemagglutinin (PHA; at 4, 10, and 50 μg/mL), both polyclonal mitogens, and the T cell receptor–specific antibody, anti- CD3/CD28 (1, 5, and 10 μg/mL). After incubation for 68 hours, each well was pulsed with 20 μL 2.5% [ 3 H thymidine]. After incubation for 4 hours, cells were harvested and collected onto a fiberglass mat. Radioactivity was measured with a Betaplate scintillation counter (Perkin Elmer, Waltham, MA). Results are reported in counts per million.
Adipokines, progesterone, C-reactive protein (CRP), and glutathione measurement
To identify hormonal influences that could contribute to immune differences between Ob and Lc subjects, leptin, adiponectin, and progesterone were measured from the serum using commercially available kits according to the manufacturer’s instructions (eBioscience). In addition, CRP was measured as a marker of inflammation using a commercially available kit (eBioscience). Oxidized to reduced glutathione ratio was measured from serum to determine differences in oxidative stress between Ob and Lc using a commercially available kit (Biovision, Milpitas, CA.)
Statistical analysis
Data are reported as mean ± SD. The Student’s t test was used for normally distributed data, and the Mann-Whitney U test was used for data that were not normally distributed. A Pearson correlation coefficient analysis was used to determine the correlations between maternal BMI, cytokines, and immunologic parameters. A Fisher exact test was used to determine the significance of binomial data. All tests were 2 sided and judged statistically significant at P < .05. SAS 9.2 for Windows and SAS Enterprise Guide 4.2 (SAS Institute, Cary, NC) were used for all analyses.
Results
Subjects
The Ob group had a mean BMI of 38.6 ± 7 kg/m 2 and the Lc group had a mean BMI of 22.8 ± 1.5 kg/m 2 ( P < .01) ( Table ). One of the subjects in each group developed gestational diabetes and were excluded from the analysis. The mean 1 hour glucose (after ingestion of 50 g glucose drink) was not different between groups (Ob 116 ± 32 mg/dL vs Lc 109 ± 27 mg/dL; P = .5). The Lc group gained statistically significantly more weight at 28 weeks of pregnancy (when blood was drawn) compared with the Ob group (Ob 12.3 ± 9.7 pounds vs Lc 21.1 ± 5.1 pounds, P < .02). At 36 weeks, there was no statistically significant difference in weight gain between the groups. The Ob group was less likely to be college educated ( P < .01.) The Ob group had more African Americans than Lc ( P < .05). All subjects in both groups reported taking a prenatal vitamin with iron daily during the current pregnancy.
Characteristic | Obese (n = 15) | Lean (n = 15) | P value |
---|---|---|---|
BMI | 38.6 ± 7.0 | 22.8 ± 1.5 | < .01 |
Age, y (mean) | 29.7 ± 4.0 | 32.0 ± 5.8 | .20 |
Education (percentage with college degree) | 47% | 67% | .46 |
Race, n | |||
White | 6 | 9 | .46 |
African American | 6 | 0 | .02 |
Hispanic | 2 | 5 | .40 |
Asian | 1 | 1 | 1.0 |
Atopic history (asthma or eczema) | 1 | 2 | 1.0 |
Smokers, n | 2 | 1 | 1.0 |
ART, n | 1 | 0 | 1.0 |
Weight gain, lbs | |||
At 28 wks | 12.3 ± 9.7 | 21.1 ± 5.1 | < .02 |
At 36 wks | 28.9 ± 6.9 | 25.7 ± 19.7 | .60 |
GDM, n | 1 | 1 | 1.0 |
1 h glucose, mg/dL | 116 ± 32 | 109 ± 27 | |
Delivered via C/S | 7/15 | 4/15 | .45 |
Chorioamnionitis/PPROM/intrapartum fever | 0/15 | 0/15 | 1.0 |
Preterm birth | 3/15 | 1/15 | .60 |
Lymphocyte subsets
There was no statistically significant difference in total lymphocytes or CD3+ T cell proportions between Ob and Lc ( Figures 1-4 ). The CD8+ T cell (CD3+/CD8+) proportion was significantly lower in Ob compared with Lc subjects (Ob, 16.4 ± 5.8% vs Lc, 23.5 ± 7.4%, P < .05; Figure 1 , A). A significant negative correlation was observed between BMI and CD8+ cells (r = −0.5, P < .01; Figure 1 , B). The CD8+ memory (CD3+/CD8+/CD 45 RO+) and CD8+ naïve (CD3+/CD8+/CD 45 RA+) proportions did not differ between groups. CD4+ T (CD3+/CD4+) cell proportions were not different between the groups; however, obese subjects had significantly lower CD4+RA+ cell proportion compared with lean subjects (Ob, 35.9 ± 7.7% vs Lc, 44.8 ± 10.8%, P < .05). There was no difference in proportions of CD4+RO+ cells between groups. Obese subjects had a lower percentage of NKT cells (CD3+/CD 314+) compared with the lean subjects, which negatively correlated with BMI (Ob, 22.9 ± 6.1% vs. Lc, 27.9 ± 4.1%, P < .05; Figure 1 , A; r = −0.4, P < .01, Figure 1 , B). Percentage of B cells (CD 19+) was higher in obese compared with lean subjects, and these percentages positively correlated with BMI (Ob, 21.9 ± 6.2% vs Lc, 13.3% ± 5.3, P < .05, Figure 1 , A; r = 0.6, P < .01, Figure 1 , B).




Intracellular cytokine measurements
There was no difference in the unstimulated intracellular level of IFNγ and TNFα between T cells of lean and obese subjects. However, when stimulated with PMA and ionomycin, obese subjects produced lower levels of intracellular TNFα and IFNγ compared with lean subjects, both in CD4+ and CD8+ T cells (TNFα: CD8+ T cells: Ob, 415 ± 242 vs Lc, 573 ± 262, P < .05; and CD4+ T cells: Ob, 406.8 ± 181.7 vs Lc, 686 ± 519, P < .05, Figure 2 , A; IFNγ: CD8+ T cells: Ob, 309 ± 226 vs Lc 502 ± 350, P < .05; and CD4+ T cells: Ob, 292 ± 281 vs Lc, 551 ± 396, P < .05, Figure 2 , B.)
Ex vivo cytokine production
There were no differences in the ex vivo production of TNFα and IFNγ as measured from the cell-free supernatant (data not shown). When stimulated with Con A, ex vivo production of IL-2, a Th1 cytokine, and IL-4, a Th2 cytokine, measured from the supernatant did not differ between Ob and Lc. Similarly, when stimulated with LPS, production of IL-6 and IL-10, Th2 cytokines measured from the supernatant did not differ between Ob and Lc.
Lymphocyte proliferation
Obese subjects had an impaired ability to proliferate in response to anti-CD3/CD28, at both 5μg/mL and 10 μg/mL (5μg/mL: Ob 22152 ± 10854 vs Lc 36576 ± 20676, P < .05; 10μg/mL: Ob 21665 ± 9975 vs Lc 38857 ± 18350, P < .05; Figure 3 ). A similar trend was observed in response to PHA, but the difference did not reach statistical significance ( Figure 3 ). There was no difference between groups in response to Con A ( Figure 3 ).
Adipokines, progesterone, CRP, and glutathione measurement
Markers that were significantly different between both groups are shown in Figure 4 , A-D. CRP was higher (Ob, 19.1 ± 14.6 vs Lc, 6.7 ± 3.0, P < .05; Figure 4 , A), and the ratio of oxidized to reduced glutathione was higher (Ob, 8.5 ± 0.01 vs Lc, 7.3 ± 0.01, P < .05; Figure 4 , B) in obese women ( Figure 4 , A and B). CRP was statistically significantly correlated with NKT cell count (r = −0.37, P < .05, data not shown). Leptin and adiponectin levels were different in Ob compared with Lc ( Figure 4 , C and D.) Leptin levels were higher (Ob, 87.2 ± 57.9 vs Lc, 32.5 ± 35.2, P < .05; Figure 4 , C) and adiponectin levels were lower (Ob, 5.1 ± 2.5 vs Lc, 9.1 ± 3.7, P < .05; Figure 4 , D) in obese compared with lean subjects. Leptin levels had a statistically significant correlation with CD8+ cell counts (r = −0.42, P < .02), B cell counts (r = 0.62, P < .01), and intracellular production of TNFα and IFNγ from CD8+ cells (TNFα: r = −0.39, P < .05; and IFNγ: r = −0.42, P < .02; data not shown). Adiponectin levels had a statistically significant correlation with CD8+ cell counts (r = 0.46, P < .02), B cell counts (r = −0.54, P < .01) and intracellular production of IFNγ from CD8+ cells (r = 0.47, P < .02; data not shown). Levels of serum IL-10 and progesterone were not significantly different between obese and lean subjects in our population (data not shown).
Comment
Our results show that obesity during pregnancy is associated with a lower proportion of CD8+ and NKT cells, decreased intracellular cytokine production in response to mitogens, and impaired lymphocyte proliferation. These findings provide a potential mechanism for the increased rates of infection seen in obese pregnancy.
In addition to lower proportions of CD8+ and NKT cells, we found that the proportion of B cells increased linearly with BMI. This could increase antibody production against previously encountered pathogens (ie, memory B cell function). Although increased numbers of B cells may augment antibody production in response to novel pathogens, cross talk is necessary between B and T cells to optimize antibody production and destroy infected cells.
T and B cells arise from common hematopoetic stem cells, which migrate to the bone marrow as B cells or the thymus as T cells. Regulators of this process of cell determination have been identified that can shift the balance between B and T cells toward B cells. The leukemia related factor (LRF)/POKemon (POK) functions to shift hematopoetic stem cell differentiation toward a B cell lineage while suppressing T cell determination. Recently, in vitro evidence has emerged that antiinflammatory agents repress the transcription of the LRF/POK family, perhaps through the transcription factor, nuclear factor-κB.
A possible explanation of our finding is that increased systemic inflammation (eg, CRP) and oxidative stress (eg, ratio of oxidized to reduced glutathione) in the obese subjects increases POK/LRF transcription, thus shifting hematopoetic stem cell differentiation towards a B cell lineage. In addition, there is evidence that changes in steroid hormone production during pregnancy are associated with decreased B cell production from bone marrow precursors. Therefore, differences in tissue steroid hormone concentrations between lean and obese individuals could provide another possible explanation of this finding.
CD8+ and NKT cells have multiple functions in combating infection. One of these functions is targeted endocytosis and apoptosis of infected cells. CD8+ T cells are necessary to combat viral and intracellular infections. Our findings could potentially explain the increase in illness severity and mortality of obese pregnant women compared with their lean counterparts during the H1N1 influenza epidemic of 2009-2010. Based on these observations, we suggest that flu vaccination might be particularly beneficial to obese pregnant women. There is evidence that obese individuals have an attenuated immune response to vaccination compared with lean individuals. The ideal vaccine dosage for this population needs further investigation.
CD8+ cells are also needed for clearance of cytomegalovirus, herpes simplex virus, and toxoplasma gondii, a protozoa, all of which can adversely affect fetal health. Although it is not routine in pregnancy, our data suggest that perhaps in the obese pregnant population, screening of immune status for these pathogens might be considered. In addition, a nonimmune status for these pathogens might prompt heightened preventive measures during pregnancy. Decreased numbers of NKT cells increase susceptibility to mycobacteria, plasmodium, and listeria. All of these pathogens have been implicated in congenital infection, with listeria being the most common of the 3 in the United States. When evaluating and treating an obese mother and her infant for a perinatally acquired infection, special attention to these organisms might be warranted.
Although Ob subjects had impaired intracellular production of TNFα and IFNγ from both CD4+ and CD8+ T cells, the levels of these cytokines in the supernatant as measured by ELISA was not different between groups. Although intracellular cytokines are measured on a per-cell basis in the same cell type, ex vivo cytokines are measured after all cell types are pan stimulated with mitogens and secreted cytokines from all cell types are combined. Therefore, it is not unexpected to see a difference between intracellular and ex vivo cytokine production. Infectious vulnerability results from the impaired ability of a specific cell type to produce a critical cytokine. Our data show that although there is heightened baseline inflammation in obese pregnant women, their ability to mount a cytokine response to pathogens is diminished compared with lean pregnant women.
Interferons are the first line of defense against viral infections and interfere with viral replication. TNF is released by activated macrophages, T lymphocytes, and other immune cells in response to a variety of infectious stimuli. The biological effects of TNF include antitumor and antiviral activity and the mediation of systemic inflammatory responses to infection as well as a crucial role in the host response to a variety of infections, particularly those involving Mycobacterium tuberculosis and other intracellular pathogens. Thus, when faced with a pathogen, particularly a viral or intracellular agent, this impaired ability to mount an appropriate cytokine response may further predispose Ob women to infectious morbidity; this has serious consequences for both maternal and fetal health.
Immune cells are active throughout pregnancy at the maternal-fetal interface. During healthy pregnancy, the decidua is rich in macrophages, NK cells, and T regulatory cells. Early in the first trimester, it has been found that the absence of any of these critical cell types prevents successful endometrial vascularization and can lead to miscarriage. Although we did not directly study the effect of obesity on the maternal-fetal interface, our findings suggest that obesity may be associated with alterations in immune cell subtypes necessary for early placentation. Recent studies have also shown that as pregnancy progresses, the placental trophoblast cells recognize and respond to pathogens, suggesting that the placenta has an active immune function. We have shown that the function of immune cells in obese pregnant women is impaired, with less cytokine production in response to stimulation with pathogens. This may also apply to the maternal-fetal interface, in which trophoblast cells may have an impaired ability to mount a cytokine response to pathogens, rendering the fetus at higher risk for infection.
Adipose tissue is an active endocrine organ that secretes numerous chemokines, adipokines, and cytokines. It is speculated that increased levels of IL-10 and progesterone, which allow for survival of the fetus, also impair the ability of the T cell receptor to respond to stimulation. Although there was no difference in levels of IL-10, tissue progesterone is known to be concentrated in adipose tissue and tissue levels could be increased in obese subjects. It is possible that higher tissue levels of this immunosuppressive hormone in obese pregnancy leads to impaired T cell function. As has been previously reported in the literature, leptin levels were higher and adiponectin levels lower in Ob vs Lc. The expression of these adipokines has been shown to correlate with BMI and circulating levels are closely related to adiposity. Both of these adipokines are known to have immunomodulatory effects, and this is another potential etiology for the differences seen in immune function in obese pregnancy.
In our subjects, obesity was associated with less maternal education, lower income, and African American race. Upon analysis, we did not find education, race, or income to be specifically associated with markers of immune function. Although glucose levels were similar in the Ob and Lc groups, there is still a possibility that the Ob group had insulin resistance with higher insulin levels. Future studies could investigate the association between insulin resistance and immune function. Additionally, the Lc group gained more weight than the Ob group at 28 weeks of pregnancy, when immune markers were measured. If both prepregnancy weight and weight gained during pregnancy modulate immune response, this would further strengthen our findings. Finally, our sample size was small and future studies should aim to replicate results in a larger, more racially diverse population.
Our results provide a potential mechanistic underpinning and show the specific components of the immune system that are dysregulated in obese pregnancy, predisposing mothers and infants to infectious morbidity. This is of particular importance because more than half of the reproductive-age women in the United States today are overweight or obese. Perinatal infections can have serious, lifelong consequences for mother and infant. Our results suggest that heightened surveillance, prevention, and treatment of specific infectious morbidities of pregnancy in obese women could be warranted.
Acknowledgments
Author contributions include the following: S.S. contributed to the research design and methodology, laboratory analysis, data analysis, and manuscript preparation; C.I. contributed to the subject recruitment, laboratory analysis, and data analysis; D.K. contributed to the laboratory analysis; A.H. contributed to the data analysis; J.A.A. contributed to the laboratory analysis and data analysis; and S.N.M. contributed to the research design and methodology and manuscript preparation.
This study was supported by a Tufts Medical Center Research Grant and US Department of Agriculture grant number 58-1950-7-707 .
The authors report no conflict of interest.
Cite this article as: Sen Sarbattama, Iyer C, Klebenov D, et al. Obesity impairs cell-mediated immunity during the second trimester of pregnancy. Am J Obstet Gynecol 2013;208:139.e1-8.
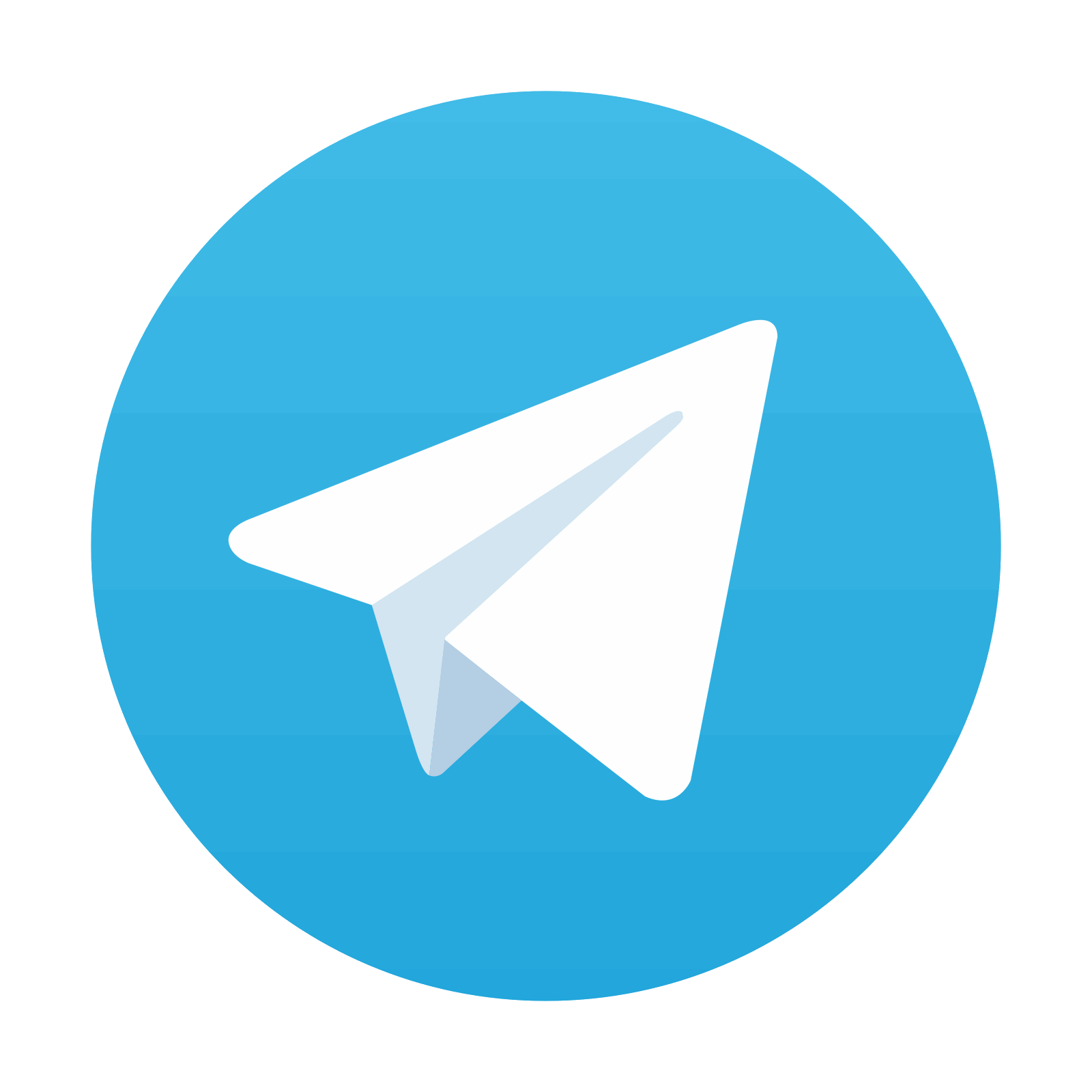
Stay updated, free articles. Join our Telegram channel

Full access? Get Clinical Tree
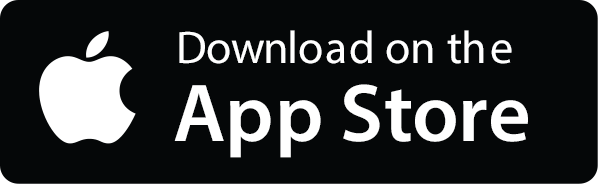
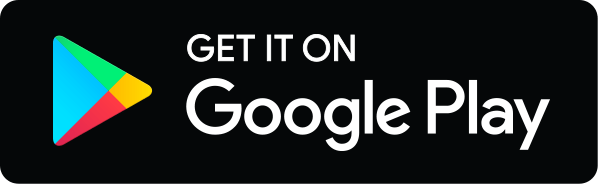