Fig. 24.1
Left lung collapse due to mucus plugging before (a) and after (b) chest physiotherapy
It is important to note that the ‘indications or contraindications for or against chest physiotherapy should never be formulated on the basis of diagnostic entities but should rather stem from a detailed analysis of the prevailing individual pathophysiology’ (Oberwaldner 2000). Therefore, when deciding on whether or not CPT may be beneficial, consider the following: if there is an excessive amount and/or retention of secretions, and if so if this is impacting on lung mechanics and/or gaseous exchange, or if there is the potential for further complications, and if there is lung or lobar collapse and whether this is due to intrinsic mucus plugging or extrinsic compression.
Considering the known complications of CPT, relative contraindications and precautions to CPT include severely ill, unstable children; pulmonary haemorrhage (spontaneous or after surfactant treatment); pulmonary oedema; coagulation defects; raised or unstable intracranial pressure; pulmonary hypertension and/or a history of hypertensive crises; and very premature or small for gestational age infants. However, in certain cases CPT may be beneficial even in children presenting with one or more of the above conditions. For example, a child with raised intracranial pressure and acute lung collapse may benefit from CPT if the lung pathology is resulting in hypoxia and hypercapnia which could lead to further increases in intracranial pressure. The physiotherapist working in PICU must be aware of intersystem dynamics and take appropriate precautions if treatment is deemed necessary.
24.2.3 Modalities
…in the case of young children with respiratory disease, we have few effective therapies, and when [you think] your only tool is a hammer, everything starts to look like a nail. … patients have respiratory difficulties from a variety of causes, but we have one hammer, so we try it on everybody. (Schechter 2007)
A number of CPT modalities are used when treating the critically ill child, but few of these have been rigorously tested scientifically.
24.2.3.1 Positioning
Positioning uses gravity to move secretions from peripheral to proximal airways thereby enhancing mucociliary clearance (postural drainage), increasing lung volumes, reducing the work of breathing, minimising the work of the heart, and optimising ventilation/perfusion ratios (Stiller 2000; Clini and Ambrosino 2005). Historically a number of postural drainage (PD) positions were advocated, with no supporting objective evidence, including inverted or head-down positions. However, head-down positioning has been shown to increase systemic blood pressure with the potential for intraventricular haemorrhage in neonates (Crane et al. 1978), increase gastro-oesophageal reflux (Vandenplas et al. 1991; Button et al. 2003) and intracranial pressure (Emery and Peabody 1983), place the diaphragm at mechanical disadvantage (Vivian-Beresford et al. 1987), and may increase venous return thereby increasing the work of the heart.
Despite no proven effect on patient outcome (Curley et al. 2005), turning patients from the supine to prone position has been shown to dramatically improve oxygenation in mechanically ventilated adults and children with acute lung injury (Casado-Flores et al. 2002; Dupont et al. 2000; Jolliet et al. 1998; Kornecki et al. 2001; Pelosi et al. 1998). It has been suggested that prone positioning recruits atelectatic dorsal regions of the lung, limits anterior chest wall movement, and reduces the effects of abdominal pressure on the thoracic cavity, thereby promoting more uniform alveolar ventilation (Matthews and Noviski 2001); perfusion is redistributed away from the previously dependent lung region (Pelosi et al. 1998); and there may be improved ventilation/perfusion matching with a reduction in intrapulmonary shunt (Marraro 2003).
The PD positions advocated for clearing secretions from specific lobes or segments have never been objectively shown to be effective, and the upright position in comparison has been shown to increase end-expiratory lung volume, optimise oxygenation, and prevent ventilator-associated pneumonia (Drakulovic et al. 1999; Stark et al. 1984; Dellagrammaticas et al. 1991). Therefore, in PICU practice the inverted position should never be used. Other positions such as side lying, upright sitting, and prone should rather be used according to the indication, preferably with the head of the bed raised (Fig. 24.2).
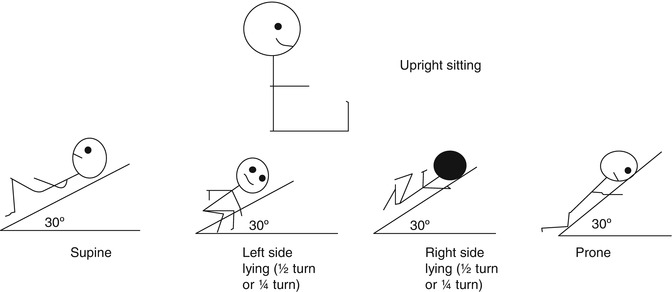
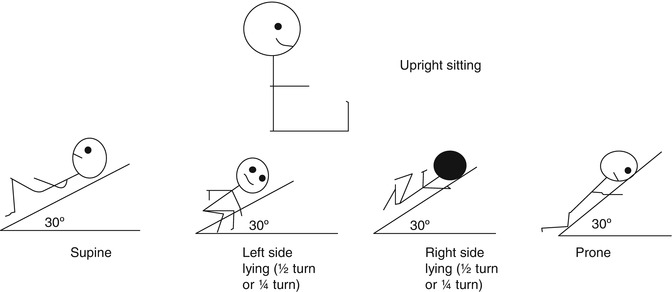
Fig. 24.2
Modified postural drainage positions for paediatric practice
24.2.3.2 Mobilisation
Teach us to live that we may dread
Unnecessary time in bed.
Get people up and we may save
Our patients from an early grave. (Asher 1947)
Mobilisation techniques that can be used for intubated, ventilated patients, depending on the patients’ stability, age, developmental level, and general condition, include active limb exercises, rolling or turning in bed, sitting in or out of bed on a chair, standing, and walking (with or without assistance) (Stiller 2000) (Fig. 24.3a, b). Mobilising patients out of bed is commonly practised in an attempt to prevent atelectasis, stimulate a cough, and improve circulation, but there is little scientific evidence supporting the practice (Stiller 2000; Branson 2007). The aims of mobilisation are numerous, including improving thoracic mobility; increasing lung volumes (Zafiropoulos et al. 2004); assisting secretion clearance; improving exercise tolerance, muscle strength, and cardiovascular fitness (Stiller 2000); preventing postural deformities; improving bone ossification; benefiting bladder and bowel function; and psychological benefits (Bailey et al. 2007). In adults, mobilisation has been shown to be safe and feasible in the early stage of ICU admission (Bailey et al. 2007). This has not been studied in the paediatric population.
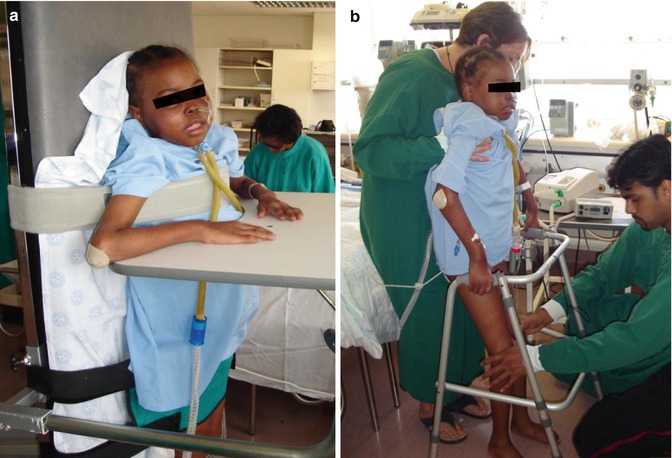
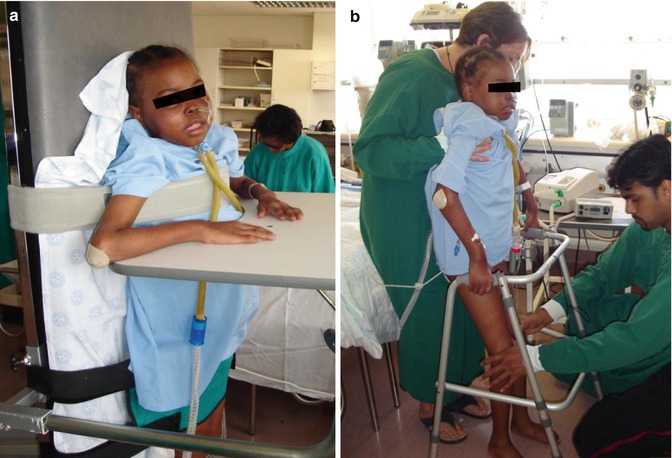
Fig. 24.3
A 10-year-old girl with following prolonged PICU stay after posttransplant complications (Photograph with parental and patient consent). (a) Mobilised to standing on tilt table (fully ventilated). (b) Assisted mobilisation using walking frame (on BiPAP ventilation)
24.2.3.3 Chest Manipulations
Percussion and vibration are techniques which are widely used to assist with removal of secretions from the lungs. They may be performed manually or with mechanical devices. It is thought that by applying chest manipulations to the chest wall, mechanical energy is transmitted into the airways where it liquefies thixotropic pulmonary secretions which can then be cleared by positioning, cough, or suctioning (Stiller 2000; McCarren et al. 2006b).
Manual vibration is a combination of compression and oscillation applied to the chest wall (McCarren et al. 2006a, b). Vibration has been shown to increase the expiratory flow rate via increased intrapleural pressure in a small randomised within-subject study of healthy adults (McCarren et al. 2006a).
Manual percussion is applied to the chest wall by means of a cupped hand or ‘tented’ fingers (Fig. 24.4), clapping the chest wall overlying the area of pathology (Stiller 2000). Percussion has been associated with cardiac arrhythmia and a drop in pulmonary compliance in critically ill adults (Stiller 2000).
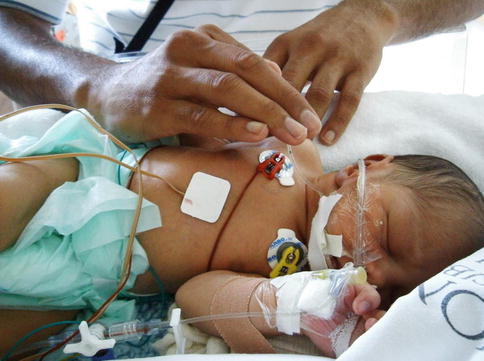
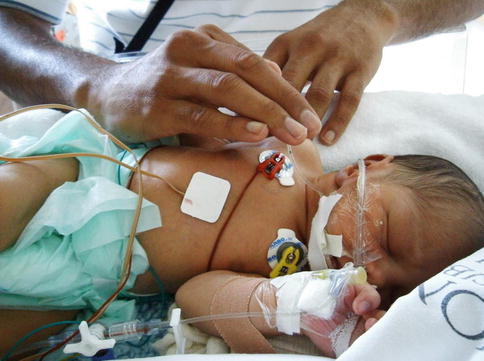
Fig. 24.4
Percussion using the ‘tenting’ technique in a 3-week-old infant with aspiration pneumonia and right upper lobe collapse (Photograph with parental consent)
Both percussion and vibration have been shown to cause or exacerbate bronchospasm (Kirilloff et al. 1985), and in an animal study the application of manual techniques was associated with the development of atelectasis (Zidulka et al. 1989). At present, the use of percussion or any external vibration method is unfounded and unsupported by scientific evidence (Stiller 2000; Branson 2007; Kirilloff et al. 1985).
24.2.3.4 Manual Hyperinflation
Physiotherapists working in adult intensive care units often use manual hyperinflation techniques in conjunction with other manipulations in order to expand the lung and loosen secretions (Patman et al. 2000; McCarren and Chow 1996). These manoeuvres are usually repeated deep manual inflations reaching a predetermined set pressure or volume with a brief inspiratory hold, followed by a quick release of the bag to enhance expiratory flow (Stiller 2000). Manual hyperinflation aims to prevent lung collapse, re-expand atelectasis, improve oxygenation and compliance, and enhance secretion clearance (Stiller 2000). It is of great concern that manual hyperinflation, and manual ventilation generally, is usually performed by delivering 100 % cold, dry oxygen, by means of devices which provide variable (often unmeasured) pressures and unknown tidal volumes, often without PEEP (O’Donnell et al. 2003).
Studies reporting the efficacy and safety of manual hyperinflation in adults have been conflicting, with some reporting improvements in atelectasis, lung compliance, and gas exchange (Stiller 2000; Patman et al. 2000; Choi and Jones 2005; Stiller et al. 1990), whilst others have found no change (Stiller 2000; Barker and Adams 2002). Other adult studies have reported increased intracranial pressure and significant cardiovascular complications (Stiller 2000). There is always the risk of overdistension of normal alveoli and barotrauma during manual hyperinflation (Stiller 2000; Branson 2007), and therefore great care should be taken when applying adult hyperinflation studies to paediatric practice.
In infants and children, performing hyperinflation manoeuvres may be especially dangerous due to the increased risk of baro- or volutrauma. The only study investigating manual hyperinflation in ventilated paediatric patients incorporated this technique into a saline-lavage-simulated cough technique, which was effective in improving lung volume in 48 of 57 infants with atelectasis not responsive to conventional CPT (Galvis et al. 1994).
It must be remembered that peak inspiratory pressure (PIP) is a proxy for inspired tidal volume. Even if the PIP is measured and controlled, one cannot directly extrapolate the tidal volume as this depends on a number of variables, particularly respiratory compliance (which changes even as the lungs expand) (O’Donnell et al. 2003). The role of ‘volutrauma’ in lung injury is well described, and limiting inspired tidal volume is an important component of lung protective ventilation (Dreyfuss and Saumon 1998; Brochard et al. 1998; Carpenter 2004). If the tidal volume is too large, it will contribute to lung damage regardless of the pressure applied, particularly with low lung compliance and immature, fragile lungs (O’Donnell et al. 2003). Considering the lack of evidence supporting efficacy of manual hyperventilation in infants and children, and the likely associated hazards, this practice cannot be considered an acceptable component of standard CPT in PICU practice.
24.3 Endotracheal Suctioning
Recommendations and clinical guidelines for ET suctioning have been made (Young 1995; Boothroyd et al. 1996; Branson et al. 1993; Runton 1992; Morrow and Argent 2008; Hodge 1991; Young 1984), but few of these have been objectively shown to be appropriate or safe. Surveys conducted in clinical settings suggest that suction practice guidelines and protocols vary widely and are not, in general, based on sound evidence (Tolles and Stone 1990; Copnell and Fergusson 1995). Consequently, ET suctioning practices vary widely among critical care practitioners (Kelleher and Andrews 2008).
24.3.1 Adverse Clinical Effects
The not-infrequent occurrence of cyanosis during endotracheal suctioning and an occasional death attributable to the procedure have prompted studies on the subject. (Boutros 1970)
Although considered necessary to prevent airway obstruction from accumulation of secretions, it is recognised that severe adverse events may result from suctioning: respiratory complications include hypoxia (Kerem et al. 1990; Singh et al. 1991), pneumothorax (Loubser et al. 1989; Anderson and Chandra 1976), mucosal trauma (Bailey et al. 1988; Loubser et al. 1989; Kuzenski 1978; Nagaraj et al. 1980), atelectasis (Boothroyd et al. 1996; Choong et al. 2003; Morrow et al. 2006), and loss of ciliary function (Bailey et al. 1988). Atelectasis has been attributed to the aspiration of intrapulmonic gas (Ehrhart et al. 1981), mucosal oedema (Boothroyd et al. 1996), or bronchial obstruction as a result of mucosal trauma (Nagaraj et al. 1980).
Cardiovascular complications of ET suctioning include bradycardia (Hoellering et al. 2008; Kohlhauser et al. 2000; Simbruner et al. 1981; Zmora and Merritt 1980; Cabal et al. 1979) and other arrhythmias (Simbruner et al. 1981) and increases in systemic blood pressure (Simbruner et al. 1981; Fanconi and Duc 1987). Raised intracranial pressure has been reported to occur with endotracheal suctioning (Tume and Jinks 2008; Kerr et al. 1997; Singh et al. 1991). Opiates and neuromuscular blockade have been shown to suppress ET-suction-induced coughing in adults, thereby minimising associated increases in intracranial pressure (Kerr et al. 1998).
ET suctioning has been implicated in nosocomial bacteraemia, attributed to the introduction of pathogens by the suction catheter (Bailey et al. 1988). Endotracheal suctioning has also been shown to be painful in neonates (Evans et al. 1997; Cignacco et al. 2008) and in adults (Puntillo 1994; Payen et al. 2001). The discomfort caused by suctioning is frequently recalled upon discharge from the adult ICU (Van de Leur et al. 2003).
Neonatal studies investigating the use of intermittent, pre-suction analgesia have had conflicting results (Pokela 1994; Cignacco et al. 2008). Considering that ET suctioning cannot and should not be scheduled, it is not appropriate to recommend intermittent analgesia for ET suction-induced pain control. Therefore, regular or continuous analgesia is recommended (Morrow and Argent 2008). Non-pharmacological pain reduction strategies such as facilitated tucking and sucrose and nonnutritive sucking are promising but require further investigation (Leslie and Marlow 2006; Cignacco et al. 2007; Helder and Latour 2008).
Some of the complications of suctioning may be due to vagal nerve stimulation (Zmora and Merritt 1980), coughing, or catheter trauma (Bailey et al. 1988; Hodge 1991; Anderson and Chandra 1976), and others may be directly related to the physical effects of suctioning on the lungs (Morrow et al. 2006; Ehrhart et al. 1981; Maggiore et al. 2003; Rosen and Hillard 1962; Hipenbecker and Guthrie 1981; Brandstater and Muallem 1969; Morrow et al. 2004; Lu et al. 2000).
The effect of different ET suctioning techniques on patient outcome, length of PICU and hospital stay, and patient mortality and morbidity is currently not known, and this requires further investigation.
24.3.2 Precautions and Contraindications
Considering that all intubated and ventilated patients may require ET suctioning in order to maintain a patent airway (Fig. 24.5), there can be no absolute contraindications to the procedure (Branson et al. 1993).
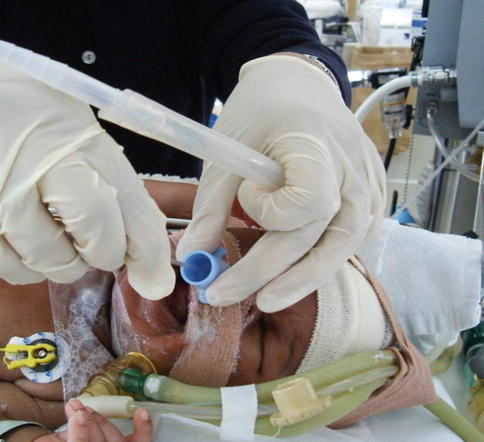
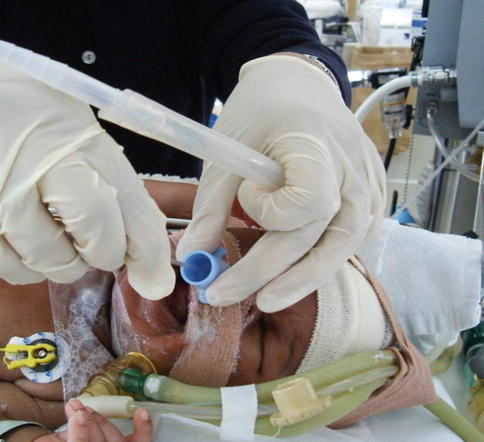
Fig. 24.5
Open-endotracheal suctioning of an infant with tracheo-oesophageal fistula and pneumonia (Photograph with parental consent)
Special care should be taken with patients who have raised intracranial pressure, as this can be exacerbated by ET suctioning and coughing (Fanconi and Duc 1987; Kerr et al. 1997; Durand et al. 1989) as can pulmonary hypertension. Patients with pulmonary oedema and pulmonary haemorrhage should only be suctioned when absolutely necessary (Pang et al. 1998; Demers 1982).
All patients should be continually monitored to assess clinical and physiological changes in response to ET suctioning.
24.3.3 Effects of ET Suctioning on Lung Mechanics
Many reported complications of ET suctioning are due to the exposure of negative pressure to the tracheobronchial tree (Kiraly et al. 2008). Lung model studies have shown that the negative tracheal or thoracic pressure induced during ET suctioning is directly proportional to the suction pressure applied and is further determined by the relationship between catheter and ETT size (Morrow et al. 2004; Kiraly et al. 2008; Copnell et al. 2009). This has also been shown to apply in clinical practice with studies of lung mechanics during ET suctioning in paediatric patients (Morrow et al. 2006, 2007; Copnell et al. 2007).
Main et al. (2004) found that, overall, there were no significant changes in tidal volume or respiratory system compliance after ET suctioning in 100 paediatric patients with variable lung disease. It was noted, however, that individual responses varied with some patients showing a marked improvement, whilst others deteriorated (Main et al. 2004).
In an observational study prospectively investigating the effects of a standardised suctioning procedure in 78 ventilated paediatric patients, Morrow et al. (2006) showed that ET suctioning reproducibly resulted in a decrease in dynamic compliance and tidal volume, attributable to a loss of lung volume, which increased to pre-suction levels again within 10 min of being reconnected to the ventilator. This recurrent derecruitment and subsequent rerecruitment on reconnection to the ventilator may exacerbate lung injury (Maggiore et al. 2003; Taskar et al. 1997; Suh et al. 2002). Choong et al. (2003) also showed that ET suctioning resulted in loss of lung volume in 14 paediatric patients receiving conventional ventilation.
Theoretically, removal of secretions from the airways should reduce airway resistance (Fox et al. 1978), but this has not been clinically demonstrated. The reduction in resistance caused by clearing the large airways could be negated if suctioning-induced volume loss occurred, with an associated increase in airway resistance. An increase in airway resistance as a result of transient bronchoconstriction following ET suctioning has also been described (Guglielminotti et al. 1998). ‘Routine’ suctioning, performed in the absence of secretions, would not be expected to drop airway resistance, as has been demonstrated clinically (Morrow et al. 2006; Main et al. 2004).
There is still no clear evidence that ET suctioning improves respiratory mechanics (Guglielminotti et al. 1998). Conversely, most evidence points to the detrimental effects of ET suctioning on lung mechanics. However, many available studies are limited by small sample sizes, patient heterogeneity, lack of intervention standardisation, and the absence of a suitable control group. Although in most studies the overall effect was found to be negative or of no benefit, individual patients did appear to improve their lung mechanics.
24.3.4 Frequency of ET Suctioning
It is generally accepted that suctioning should not be performed routinely but rather as indicated following a thorough clinical assessment (Branson 2007; Day et al. 2002). Observational studies of clinical practice have suggested that the identification of the need for ET suctioning is complex, involving changes in both clinical signs and patient behaviour (Thomas and Fothergill-Bourbonnais 2005).
Guidelines based on expert consensus have suggested that clinical indications for suctioning should include audible or visible secretions in the ETT or coarse breath sounds on auscultation, coughing, increased work of breathing, arterial desaturation and/or bradycardia as a result of secretions, decreased tidal volume during pressure-controlled ventilation, the need for a tracheal aspirate culture (Guglielminotti et al. 2000; Branson et al. 1993; Branson 2007), and following CPT in order to clear mobilised secretions. If ventilators are equipped with flow-volume loop displays, changes in graphics (Branson et al. 1993) or a saw-toothed pattern may indicate the presence of secretions in the ETT. Patients receiving high-frequency oscillatory ventilation should be observed with regard to the amount of chest wall oscillation – if this changes it may indicate the presence of secretions.
Many of these indications are very subjective, and closer monitoring of, for example, transcutaneous pCO2 levels may provide a more objective indication for suctioning. This requires investigation.
24.3.5 Open- Versus Closed-System ET Suctioning
As with electric hand-dryers public acceptance does not always mean demonstrable efficacy. (Lindgren 2007)
Commonly used suctioning systems are open-ET suctioning (OES) and closed-system suctioning (CSS). OES involves first disconnecting the patient from the ventilator and then suctioning the ETT before reconnecting the patient to the ventilator circuit. CSS is performed through an adaptor inserted at the ETT-ventilator circuitry interface. The catheter is encased in a plastic sleeve on insertion, providing a seal that maintains a closed system (Taggart et al. 1988), allowing ventilation to continue during the suctioning procedure.
Initial studies suggested that use of CSS may prevent ET suctioning-induced hypoxia and decreases in lung volume in paediatric (Choong et al. 2003) and adult (Taggart et al. 1988) patients. However, animal and clinical studies have shown no advantage of CSS in terms of lung volume protection (Copnell et al. 2009; Tingay et al. 2010; Hoellering et al. 2008; Heinze et al. 2008) (Table 24.1).
Table 24.1
Main findings of studies comparing open- and closed-system suctioning in adults, paediatric patients, and neonates
Reference | Study type | Patient group | Sample size (n) | Main finding |
---|---|---|---|---|
Hoellering et al. (2008) | Randomised crossover | Neonates | 30 | Both OES and CSS resulted in variable and transient desaturation, reductions in heart rate, and lung volume changes which were not significantly different between the two methods |
Heinze et al. (2008) | Randomised crossover | Adult | 20 | No difference in functional residual capacity loss following three suctioning methods (CSS during pressure-controlled and volume-controlled ventilation and OES) |
Peter et al. (2007) | Meta-analysis | Adults | 9 RCTs. n = 1,292 | No difference between OES and CSS on incidence of VAP or mortality |
Jongerden et al. (2007) | Meta-analysis | Adults | 15 RCTs. n = 1,436 | No difference between OES and CSS on incidence of VAP or mortality |
Vonberg et al. (2006) | Meta-analysis | Adult | 9 RCTs. n = 1,292 | No difference between OES and CSS on incidence of VAP |
Freytag et al. (2003) | Prospective randomised controlled | Adults | 23 | Increase in lower respiratory tract colonisation if CSS catheter unchanged for 72 h |
Choong et al. (2003) | Randomised crossover | Paediatric | 14 | Total lung volume loss and desaturation greater with OES |
Kalyn et al. (2003) | Randomised crossover | Neonates | 200 | CSS maintained better physiological stability, and recovery time was reduced |
Woodgate and Flenady (2003) | Systematic review | Neonates | 2 RCTs. n = 22 | Insufficient evidence available |
Cordero et al. (2000)) | Prospective randomised controlled | Preterm neonates | 175 | No benefit of CSS for a number of outcome measures |
Morrow et al. (2012) | Nonrandomised controlled trial | Paediatric | 250 | No benefit of CSS on incidence of VAP, PICU stay, duration of ventilation, or mortality |
The drawbacks of CSS include the risk of producing high negative pressures (Stenqvist et al. 2001) if the amount of air suctioned exceeds the gas flow delivered to the patient by the ventilator, reduced efficiency in clearing secretions from the airways (Copnell et al. 2007; Lindgren et al. 2004), and the high financial cost of the system which has to be replaced regularly in order to avoid microbial lower respiratory tract colonisation (Freytag et al. 2003; Meyer et al. 2009). Practically, there is also a risk of not withdrawing the catheter completely after the suctioning event, thus partially occluding the ETT and increasing airway resistance.
24.3.6 Preoxygenation
A meta-analysis of 15 adult studies showed that the occurrence rate of hypoxia was 32 % lower when preoxygenation was applied before suctioning than if it was not applied (Oh and Seo 2003).
Kerem et al. (1990) examined ways of preventing hypoxia during ET suctioning in a prospective crossover trial of 25 haemodynamically stable paediatric patients. Patients underwent one of four suctioning approaches: preoxygenation, hyperinflation pre-suction, hyperinflation post-suction, and a control with no treatment. The significant fall in SaO2 and PaO2 occurring as a result of suctioning was completely prevented by delivering 100 % inspired O2 for 1 min before the procedure.
The optimal degree and duration of preoxygenation is currently not known (Oh and Seo 2003). Branson et al. (1993) suggested that patients should receive 100 % inspired O2 for >30 s prior to suctioning. Hodge (1991) suggested increasing the FiO2 by 10–20 % higher than the baseline fraction of inspired oxygen (FiO2) for about 1 min before suctioning. Neither of these recommendations is supported by high-level evidence.
Due to the known risks of hyperoxia, it is essential that FiO2 be returned to pre-suctioning levels as soon as the SaO2 has stabilised.
24.3.7 Suction Catheter Size
If a catheter largely or completely occludes an artificial airway or bronchus, the full suction pressure may be transmitted to that airway leading to atelectasis (Morrow et al. 2004, 2006; Rosen and Hillard 1962). Therefore, the most severe lung volume changes are likely to occur during ET suctioning of young infants intubated with small internal diameter ETT, as in these patients, the catheters used will always be relatively large compared to the ETT size. The suction catheter chosen should therefore be large enough to effectively suction thick secretions but not so large that it traumatises the mucosa or occludes the ETT (Singh et al. 1991).
In a prospective study of 17 ventilated paediatric patients, Singh et al. (1991) found that significant changes in SaO2, heart rate, and intracranial pressure occurred during ET suctioning regardless of the catheter diameter. A catheter with outer diameter: ETT inner diameter of 0.7 was found to be the easiest to introduce into the ETT and was most effective in clearing secretions (Singh et al. 1991).
The recommendation for catheter size selection presented in Table 24.2 (Morrow and Argent 2008) has not been subjected to rigorous testing by means of a prospective controlled clinical trial. It is recommended that this be used as a guideline until stronger evidence is available.
Table 24.2
A proposed guideline for suction catheter selection
Age | Weight (kg) | ETT (mm ID) | Catheter size (FG) | ||
---|---|---|---|---|---|
Mucus viscosity | Liquid | Medium | Thick | ||
0–3 months | 3.5 | 3.5 | 5 | 6 | 7 |
3 months | 6 | 3.5 | 5 | 6 | 7 |
1 year | 10 | 4.0 | 6 | 7 | 7 |
2 years | 12 | 4.5 | 6 | 7 | 8 |
3 years | 14 | 4.5 | 6 | 7 | 8 |
4 years | 16 | 5.0 | 7 | 8 | 8 |
6 years | 20 | 5.5 | 7 | 8 | 8 |
8 years | 24 | 6.0 | 8 | 10 | 10 |
10 years | 30 | 6.5 | 8 | 10 | 12 |
12 years | >30 | 7.0 | 8 | 10 | 12 |
24.3.8 Vacuum Pressure
When selecting suction pressures the balance between effective suctioning of secretions and potential risk to the patient must be considered. The suction pressure should be high enough to be effective in removing secretions but not so high that it causes mucosal damage or lung volume loss. There is still no high-level evidence supporting a maximum safe and effective suction level.
The findings of an observational paediatric study suggest that suctioning in the presence of ETT secretions may not result in loss of lung volume (Morrow et al. 2006) because negative pressure in the lungs produced during suctioning would only occur whilst air was flowing through the suction catheter. As soon as secretions are drawn into the catheter, the pressure in the lungs would return to that of the atmosphere (Rosen and Hillard 1962). However, routine suctioning, which often occurs in the absence of secretions, is likely to cause significant atelectasis. Therefore, although suction pressures should be limited, the issue may not be as critical when suctioning only when indicated to do so in the presence of secretions.
Results of an animal study showed that mucosal trauma occurred when using suction pressures of both 100 and 200 mmHg; however, damage was greater at the higher suction level. This study also suggested that efficiency of aspiration was not affected by the suction pressure used (Kuzenski 1978). Conversely, in an in vitro study, it was shown that suction pressures up to −360 mmHg measured at the vacuum source were more effective in removing secretions than using vacuum pressures of ~ −200 mmHg (Morrow et al. 2004). These suction pressures were the lowest two options on the commercially available suction units in use at the time of these investigations.
Most authors advocate a range of suction pressures from 70 to 150 mmHg (Hodge 1991; Kacmarek and Stoller 1995). Young (1984) suggested that these pressures may be increased to 200 mmHg (27 kPa) to aspirate thick secretions. Singh et al. (1991) did not show any difference in the change of physiological parameters when suctioning children using vacuum pressures of 80, 100, or 120 mmHg. Clinical studies have not comparatively investigated the effects of higher suction pressures on physiological changes, efficacy of secretion removal, or patient outcome.
The potential impact of high suction pressures (potential mucosal damage and lung volume loss) needs to be weighed against the potential damage that may occur with repeated suction passes when using an inadequate vacuum level.
24.3.9 Sterility
There is a risk of introducing pathogens into the respiratory tract during ET suctioning, largely as a result of environmental exposure of the suction catheter (Cobley et al. 1991). Therefore, it has been suggested that a strictly aseptic technique be used during ET suctioning (Branson et al. 1993; Wood 1998). During ET suctioning, however, the catheter is passed through an unsterile port, which may be colonised with potentially pathogenic organisms, into the ETT. This will occur regardless of sterility. A randomised controlled trial of 486 intubated children and infants found that reusing a disposable suction catheter in the same patient over a 24-h period did not affect the incidence of nosocomial pneumonia (Scoble et al. 2001).
The emergence of multidrug-resistant organisms in the PICU setting and the spread among patients pose the threat of outbreaks of untreatable infectious diseases with significant associated mortality and morbidity. Use of infection control precautions to prevent transmission among patients is, therefore, a top priority (Siegel 2002). Considering that transmission of infectious organisms from patient to patient frequently occurs on the hands of healthcare workers (Siegel 2002), hand washing before and after patient contact is essential despite the wearing of gloves and regardless of suctioning method (open or closed). There is also a risk of infection to the person performing ET suctioning (Curtis et al. 1999; Rabalais et al. 1991). Standard and transmission-based precautions are the only preventive measures for minimising this risk (Siegel 2002).
The recommended contact and standard precautions for patients with presumed infectious diseases include the use of gloves (either ‘clean’ or sterile); face protection (face masks and goggles) for open-ET suctioning, which is likely to cause splashes or sprays of secretions; washing hands before and after donning gloves; and wearing a gown to protect the skin and prevent contamination of the clothes (Siegel 2002). Specific pathologies may require more complex barrier protection.
Although the same suction catheter may be used for several suction passes (Scoble et al. 2001), external environmental contamination should be limited. The suction catheter should be immediately discarded if it comes into contact with any surfaces and should not be used to suction the nose or mouth before introduction into the ETT.
24.3.10 Duration of Suctioning
Increasing the duration of suction application significantly increases the amount of negative pressure within a lung model (Morrow et al. 2004) and has been implicated in the degree of hypoxia induced (Rosen and Hillard 1962; Brandstater and Muallem 1969). Although there is currently no strong evidence supporting an appropriate duration of suctioning, most authors recommend between 10 and 15 s (Branson et al. 1993; Young 1984). Runton (1992) suggests that the actual time of negative pressure application during each suctioning event be limited to ≤5 s.
24.3.11 Depth of Catheter Insertion
The depth of insertion of the suction catheter during ET suctioning varies according to institutional practice (Spence et al. 2003). In shallow ET suctioning, the catheter is passed to the tip of the ETT, whereas in deep ET suctioning, the catheter is passed beyond the ETT into the trachea or bronchi, usually until resistance is felt.
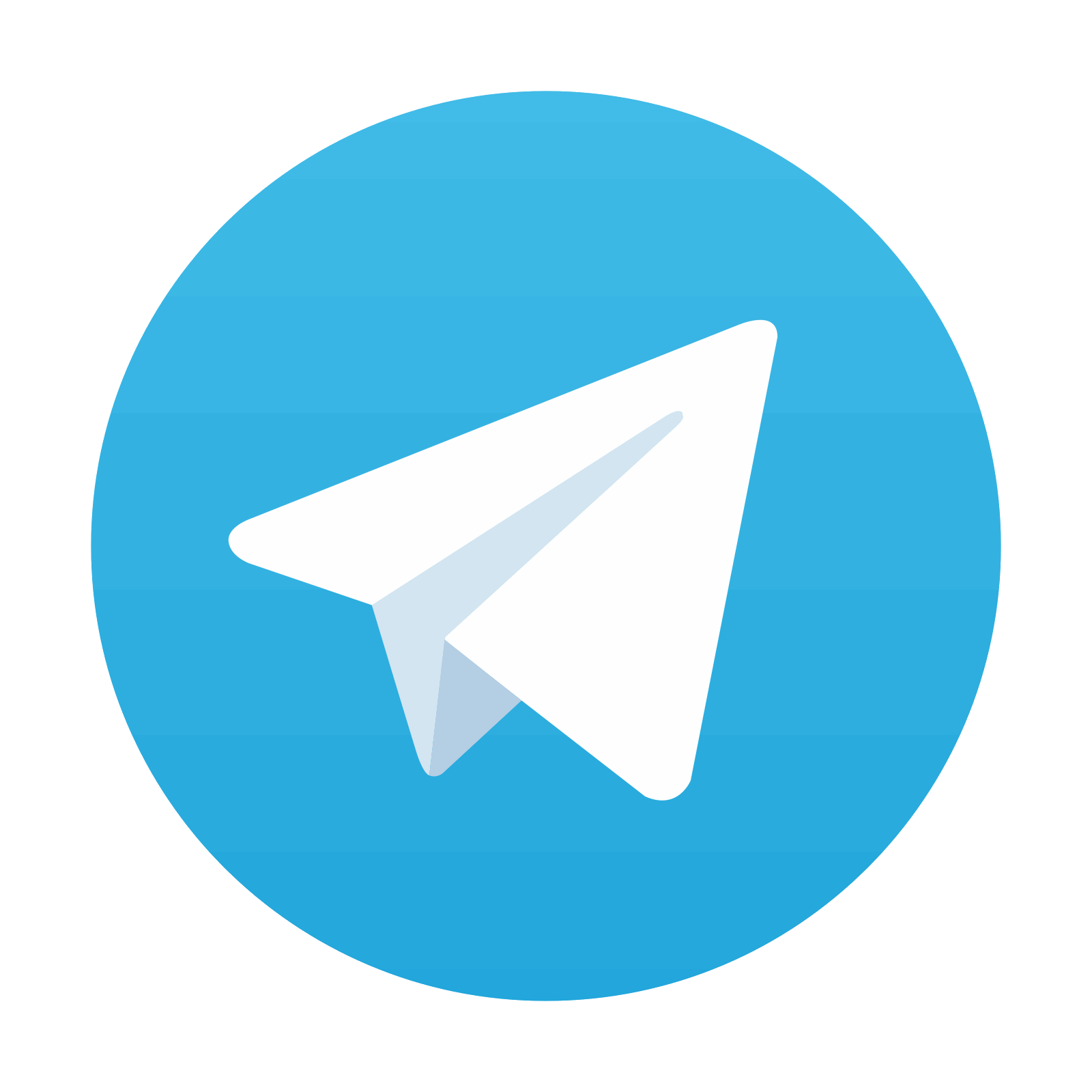
Stay updated, free articles. Join our Telegram channel

Full access? Get Clinical Tree
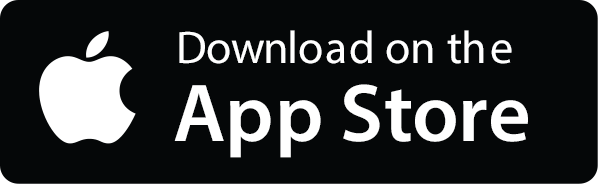
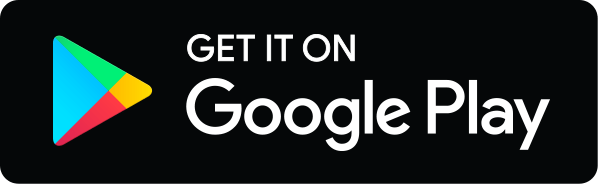