- •
Invasive mechanical ventilation of neonates increases their risk of developing bacterial infection of the lower airways and lung parenchyma, which is termed “ventilator-associated pneumonia” (VAP).
- •
VAP is diagnosed on the basis of defined clinical, radiographic, and laboratory criteria.
- •
Unlike other neonatal bacterial infections, culture isolation of a single, causative organism is unusual in VAP.
- •
Suspected VAP should initially be treated with broad-spectrum antibiotics with coverage against common drug-resistant bacteria.
- •
As treatment progresses, empiric antibiotic coverage should be narrowed—as possible—based on available data.
- •
VAP is associated with prolonged hospitalization and poor clinical outcomes, including death.
- •
VAP “bundles,” consisting of standard practices universally applied to prevent pneumonia among intubated patients, are accruing strong evidence and are now in widespread use.
Introduction
Infants in the neonatal intensive care unit (NICU) requiring mechanical ventilation (MV) can develop superimposed bacterial infection of the small airways and lung parenchyma, which is termed “ventilator-associated pneumonia” (VAP). VAP is classified as a type of health care–associated infection (also known as nosocomial infection). Health care–associated infections have come under increasing scrutiny as potentially preventable contributors to poor hospitalization outcomes and ballooning costs of inpatient care.
VAP is difficult to diagnose in the neonate and therefore requires a high index of suspicion. The concept of VAP first emerged in literature from adult ICUs, where a specific etiologic diagnosis can be aided by invasive airway sampling through bronchial brushings or lavage—techniques that are rarely used in the NICU. Adding further challenge to identifying VAP in the neonate is the fact that affected infants often have chronic pulmonary inflammation and dysfunction related to prematurity and respiratory support, which can complicate the diagnostic impression and make infection difficult to detect. Finally, as is the case with neonatal infection in general, infants with VAP show fewer localizing signs and symptoms than older children and adults, often presenting with general deterioration that may not immediately be attributed to VAP.
Nevertheless, with increasing awareness of VAP as a contributor to poor NICU outcomes, guidelines for diagnosis, management, and prevention have been developed and refined. This chapter reviews VAP epidemiology, pathogenesis, and the latest recommendations for limiting its impact on neonatal health.
Definition
The Centers for Disease Control (CDC)/National Nosocomial Infections Surveillance (NNIS) define VAP as pneumonia occurring in the setting of at least 2 days of MV through an endotracheal tube (ETT). Noninvasive forms of ventilation such as nasal continuous positive airway pressure or intermittent positive pressure through nasal prongs do not qualify. Formal criteria for diagnosing pneumonia are based on a combination of radiographic, laboratory, and clinical findings.
When there is underlying respiratory or cardiac disease (such as respiratory distress syndrome, chronic lung disease, or a patent ductus arteriosus), at least two serial chest radiographs demonstrating a new or progressive focal infiltrate, consolidation, cavitation, or pneumatocele are required to meet the radiographic diagnostic criteria for pneumonia. For an infant with no preexisting pulmonary or cardiac disease, a single chest radiograph demonstrating one or more of the above features is sufficient.
The clinical and laboratory findings required to diagnose pneumonia in patients younger than 1 year of age include worsening gas exchange (manifesting as desaturations, need for increasing ventilator settings, and/or a rising fraction of inspired oxygen requirement) and at least three of the following:
- •
Temperature instability
- •
Leukopenia (≤4000 white blood cells/mm 3 ) or leukocytosis (≥15,000 white blood cells/mm 3 ) and left shift (>10% band forms)
- •
New onset of purulent sputum, change in character of sputum, or increased respiratory secretions requiring increased suctioning frequency
- •
Apnea, tachypnea, or retractions of the chest wall
- •
Wheezing, rales, or rhonchi
- •
Bradycardia (<100 beats/min) or tachycardia (>170 beats/min)
In studies of VAP that have reported on the frequency of different clinical signs in newborns, the need for increased ventilator settings, increased airway secretions, and a new radiographic infiltrate have been described as the most common.
Some authors have argued for inclusion of microbiologic criteria in the definition of VAP, and positive culture results from suctioned sputum, bronchoscopy, blind bronchoalveolar lavage (BAL), or pleural fluid have sometimes been included as diagnostic criteria in clinical studies. However, there are several downsides to relying on microbiologic evidence of VAP in neonates. For example, suctioned secretion samples are frequently contaminated with bacteria colonizing the oropharynx and upper airway. The use of BAL may reduce this contamination. Comparisons between concurrent cultures of secretions suctioned from an endotracheal tube and BAL have shown that BAL samples are less likely to yield polymicrobial growth, suggesting less oropharyngeal and upper airway commensal contamination. However, BAL can be technically challenging in smaller patients and may not be feasible in an unstable infant. Bronchoscopy poses the same, if not greater, risks as BAL. Large infectious pleural effusions are unusual in neonatal VAP; therefore pleurocentesis should be reserved for cases when an effusion is hindering respiratory mechanics. Blood cultures are not reliable for diagnosing VAP.
Although not required to diagnose VAP, Gram stain and culture of a tracheal aspirate sample can provide valuable supplemental evidence. A Gram stain of tracheal secretions that shows a significant leukocytic infiltrate and a high bacterial load is consistent with VAP, and the bacterial morphology can potentially help inform antibiotic selection (see the “Treatment” section). Serial cultures and microscopic assessments of tracheal aspirate samples during treatment can be useful gauges of the patient’s response to antibiotics. Results from tracheal suction samples should not be considered definitive, however, and the diagnosis of VAP can be made solely based on radiographic, clinical, and laboratory criteria described previously and summarized in Box 8.1 .
a Infants receiving mechanical ventilation through an endotracheal tube for at least 48 hours must meet criteria in all three columns .
Radiographic | Worsening Gas Exchange | Clinical/Laboratory Evidence |
---|---|---|
If there is underlying pulmonary or cardiac disease, two serial x-rays demonstrating at least one of the following:
| Any of the following:
| Must have at least three of the following:
|
VAP, Ventilator-associated pneumonia; WBC, white blood cell.
Epidemiology
While the reported incidence of VAP varies depending on the source, neonatal VAP is common, accounting for 6.8% to 32.2% of health care–associated infections in level II and III NICUs in the United States. VAP rates appear to be decreasing. Sequential reports from the National Healthcare Safety Network, published in 2009 and 2013, show a drop from 1.9 to 1.2 cases of VAP per thousand ventilator days in level II and III NICUs.
Prematurity, low birth weight, and duration of MV have all been identified as major risk factors for VAP in multiple studies. Since smaller, sicker patients tend to require longer treatment with MV, it is difficult to conclusively establish which of these variables are independent risks. Several authors have shown statistically significant differences in VAP incidence per thousand ventilator days based on gestational age, but this analytical approach could be confounded by an uneven distribution of MV duration within preterm and term populations.
Cernada et al. published a prospective study of VAP in 198 neonates (gestational age range 27–37 weeks) intubated for more than 48 hours; VAP developed in 18 of the infants. In a multivariate regression model, only duration of MV emerged as an independent risk factor for VAP. In contrast, Apisarnthanarak et al. performed logistical regression on data from 19 extremely premature infants with VAP and found no significant independent risk from each additional week of MV. Instead, that group identified prior bloodstream infection as an independent risk factor for preterm VAP, although there was no significant relationship between the organism causing the prior bloodstream infection and the isolate (if any) responsible for pneumonia. One possibility is that treatment of an earlier infection with antibiotics may alter the microbiome of the neonate and allow colonization with pathogens more likely to cause VAP.
The infection control infrastructure of the NICU may also significantly affect local rates of VAP. An observational study by Goldmann et al. reported a 16-fold decrease in VAP after relocation of their nursery to a new facility with 50% more staffing, improved isolation and cohorting capacity, more sinks, and better air filtration. Other potential risk factors for VAP include administration of opiates for sedation during intubation, frequent suctioning (>8 times per day), and reintubation.
Pathogenesis
The most common organisms cultured from respiratory cultures in the setting of neonatal VAP are Pseudomonas aeruginosa , Enterobacter spp., Klebsiella spp., and Staphylococcus aureus ( Box 8.2 ). Tracheal aspirates from the ETT almost always yield polymicrobial growth owing to commensal contamination. BAL samples have a higher likelihood of growing a single isolate, but Cernada et al. still reported that 16% of BAL samples from neonates with VAP grew multiple organisms. Therefore it is likely that VAP often results from polymicrobial overgrowth rather than a single pathogen.
a Based on multiple studies; the exact order may vary depending on local factors.
Immature innate immunity in the neonate increases the risk of VAP. Low immunoglobin levels (particularly in the premature population) and functionally impaired alveolar neutrophils and macrophages limit opsonization and phagocytosis of bacteria in the lower airways. Tissue damage from chronic inflammation, atelectasis, and pulmonary edema create potential niduses of infection and impede normal mucosal barrier functions and ciliary clearance of debris.
Although intubation can be lifesaving, the ETT itself contributes to VAP in multiple ways. It creates a physical barrier to ciliary action, prevents effective coughing, and provides a protected milieu for high-density bacterial colonization and biofilm formation. Zur et al. used electron microscopy to demonstrate progressive biofilm growth on the inner luminal and outer surfaces of ETTs from neonates intubated for at least 12 hours ( Fig. 8.1 ). Adair et al. performed within-patient comparisons of bacterial culture results from respiratory secretions and ETT biofilm swabs in 40 intubated patients and used genotyping to confirm clonal matches. Patients with VAP showed high correlation between ETT and sputum isolates, whereas controls without VAP showed no statistical correlation, suggesting that the ETT serves as a reservoir for pathogenic organisms once infection is established.

VAP develops from overgrowth of bacteria colonizing the oropharynx, which may have been present before intubation or may become introduced afterward from contaminated oral or gastric secretions. Through alterations in the tracheobronchial milieu described earlier, MV leads to positive selection for these organisms, followed by tracheal colonization and population expansion in the lower airways ( Fig. 8.2 ).

Patient positioning may influence pooling of orogastric secretions and the propensity of oropharyngeal commensals to be drawn into the trachea. Aly et al. performed a randomized controlled trial to test the hypothesis that gravity contributes to tracheal colonization, which may progress to VAP. They compared tracheal cultures among 60 intubated infants who were maintained in either supine or side-lying positions and showed that after 5 days of intubation, there was significantly more tracheal colonization among the supine group, which also had higher tracheal bacterial density and greater introduction of new species over the observation period.
The contribution of gastric bacteria to VAP pathogenesis is uncertain. Some experimental evidence supports the hypothesis that the stomach serves as a reservoir for potential VAP pathogens. Gastric pepsin has been shown to be present in the lungs of intubated neonates, indicating that gastric secretions become aspirated in this population. One study measured tracheal pepsin among intubated neonates and identified a reliable inverse relationship with the degree of head of bed elevation. In a study on 19 intubated adults in a medical ICU, technetium labeling of gastric contents was followed by scintigraphy of endotracheal suctioning samples to assess for migration of gastric bacteria to the lungs. Radioactivity counts were compared between patients maintained in supine and semi-recumbent positions. Migration occurred in both groups but happened faster among patients in the supine position, again indicating a role of patient positioning in the pathogenesis of VAP.
Other studies have cast doubt on the theory that gastric bacteria are a major cause of VAP. Cardeñosa Cendrero et al. performed daily, simultaneous sampling of the trachea, pharynx, and stomach in 123 adults receiving mechanical ventilation to study temporal patterns of colonization and invasion. Nineteen patients in whom VAP developed subsequently underwent bronchoscopy with BAL and protected brush sampling. There was no evidence of primary gastric colonization for any of the VAP isolates, which generally matched preceding tracheal colonizers. A smaller study by Feldman et al. with a similar design also did not reveal any evidence of primary gastric colonization with eventual VAP pathogens.
In summary, VAP pathogenesis data are limited and—in some regard—contradictory. The current model is also derived largely from adult research, which may limit its generalizability to the neonatal population. Based on the available evidence, the key steps of VAP pathogenesis seem to be initial tracheal colonization by one or more potentially virulent microorganisms, followed by progressive distal airway colonization and population expansion. This process is aided by the ETT itself, which offers a protected niche for potential pathogens; alterations in innate pulmonary immunity stemming from prematurity and chronic disease; and possibly from supine positioning that favors bacterial spread from the upper airways into the lungs.
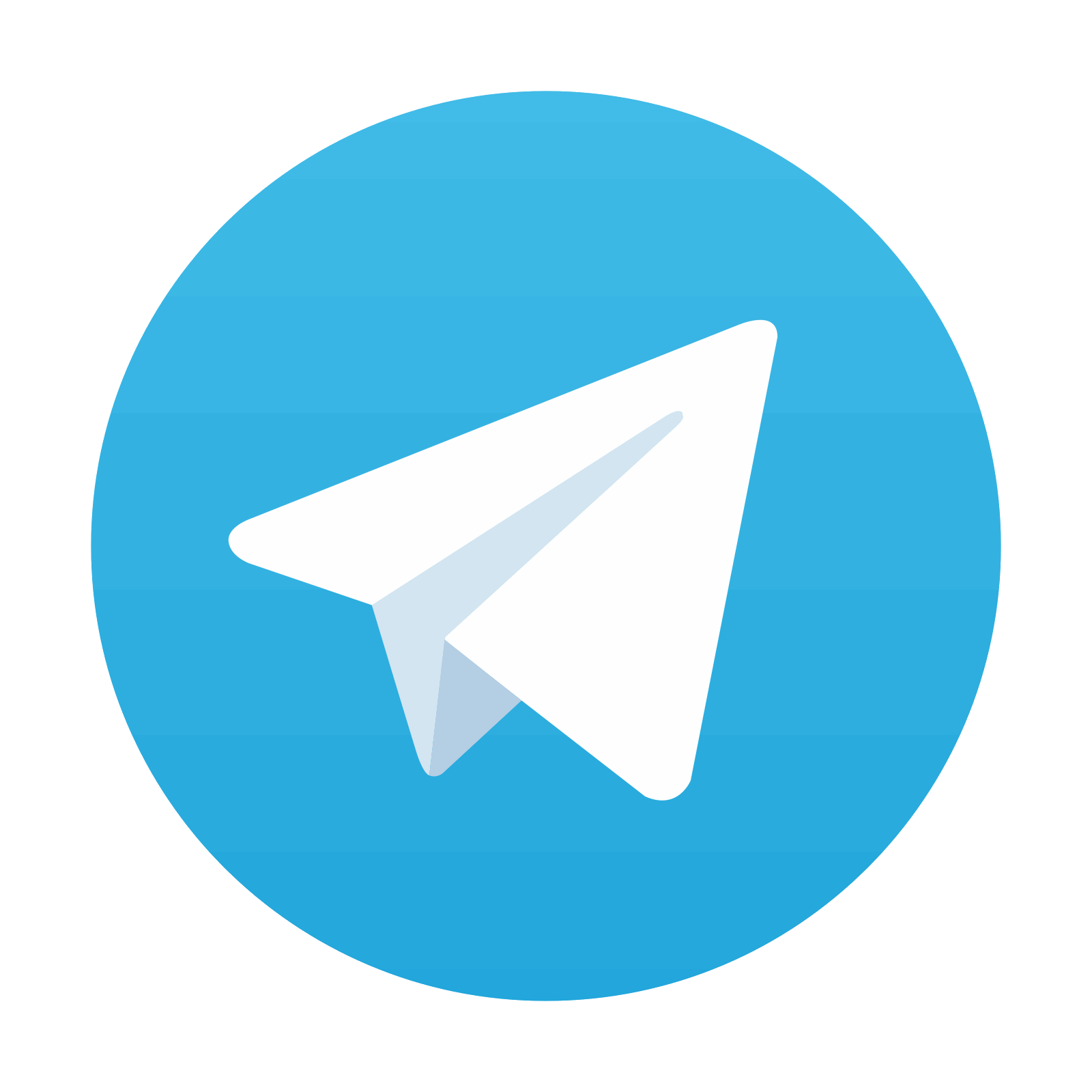
Stay updated, free articles. Join our Telegram channel

Full access? Get Clinical Tree
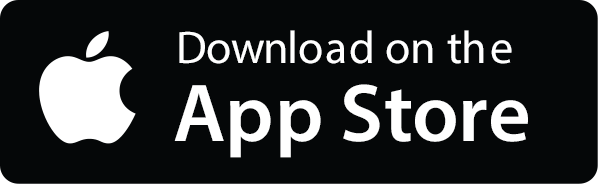
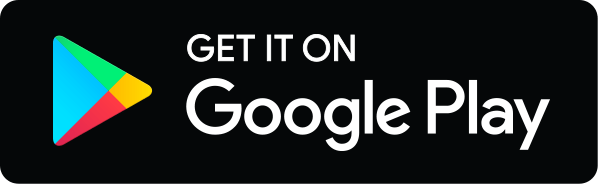