For over a decade, researchers have focused their attention on the development of non-invasive prenatal diagnosis tests based on cell-free fetal DNA circulating in maternal blood. With the possibility of earlier and safer testing, non-invasive prenatal diagnosis has the potential to bring many positive benefits to prenatal diagnosis. Non-invasive prenatal diagnosis for fetal sex determination for women who are carriers of sex-linked conditions is now firmly established in clinical practice. Other non-invasive prenatal diagnosis-based tests are set to follow, as future applications, such as the detection of single-gene disorders and chromosomal abnormalities, are now well within reach. Here, we review recent developments in non-invasive prenatal diagnosis for genetic conditions and chromosomal abnormalities, and provide an overview of research into ethical concerns, social issues and stakeholder view points.
Introduction
Traditionally, prenatal diagnosis of fetal genetic status or aneuploidy has been dependent on the use of invasive diagnostic tests (chorionic villus sampling [CVS] and amniocentesis) to obtain a sample of fetal genetic material. The invasive nature of these tests means that there is a small but significant risk of miscarriage of around 1%, and alternative sources of fetal material have long been sought to allow non-invasive prenatal diagnosis (NIPD).
The original focus for NIPD research was fetal cells in the maternal circulation. This line of investigation, however, has not been fruitful, as fetal cells have proven difficult to isolate and may not be pregnancy specific. In 1997, Lo et al. identified the presence of cell-free fetal DNA (cffDNA) in maternal plasma. Cell-free fetal DNA can be detected from 4–5 weeks gestation. Importantly, it is rapidly cleared from the maternal circulation after delivery, which means cffDNA is pregnancy specific. Free-fetal DNA emanates from trophoblast cells in the placenta, and is made up of relatively short fragments of DNA (143 bp in length on average). It is now also evident that the whole fetal genome is represented in the maternal plasma.
Most cell-free DNA (cfDNA) in the circulation is maternal in origin. Digital polymerase chain reaction (PCR) has allowed precise quantification of cfDNA levels, showing that the fetal component contributes around 9% in early pregnancy, increasing to 20% as the pregnancy progresses ; however, this is highly variable between pregnancies. The key limiting factor in the development of specific prenatal tests based on cffDNA has been the difficulty of differentiating the genetic material from the fetus from that of the mother. As a result, the first clinical applications of NIPD have been restricted to the identification of alleles present in the fetus but not in the mother (either inherited from the father or arising de novo ). These include fetal sex determination, paternally inherited single-gene disorders, or those arising de novo , such as achondroplasia. This perspective is rapidly changing with the development of new technologies such as digital PCR and massively parallel sequencing (MPS). These allow researchers to develop NIPD tests for single-gene disorders where parents have the same mutations and for aneuploidies, both of which need to take into account the presence of the mother’s allele or chromosomes.
In addition to testing for genetic and chromosomal disorders, NIPD can also be used to manage other complications of pregnancy; indeed, one of the early success stories for NIPD has been fetal rhesus genotyping in mothers who are rhesus-D (RhD) negative. Analysis of cffDNA in the plasma of RhD-negative pregnant women who have a past history of haemolytic disease of the newborn or have elevated levels of anti-D antibodies has been used clinically to determine the fetal RhD status for almost a decade, and fetal RhD typing of all RhD-negative pregnant women has the potential to become routine clinical practice in the next few years. In addition, complications such as pre-eclampsia, preterm labour and fetal restricted growth, have been shown to be associated with increased concentrations of cell-free fetal DNA and, in the future, at-risk pregnancies may be identified through NIPD.
In this review, we focus on the use of NIPD for genetic and chromosomal disorders. Current research, clinical applications and an overview of research into ethical concerns, social issues and stakeholder viewpoints are described.
Fetal sex determination
Fetal sex determination using cffDNA is a well-established prenatal test that can be carried out reliably from 7 weeks gestation using real-time quantitative PCR (RT-qPCR) to identify the presence or absence of Y chromosome-specific sequences in the maternal plasma. The most common clinical indication for early fetal sex determination is for carriers of X-linked genetic disorders, such as Duchenne muscular dystrophy (DMD) or adrenoleucodystrophy, where male-bearing pregnancies are primarily at risk. For women who are carriers of X-linked conditions, early fetal sex determination using cffDNA can inform the uptake of invasive testing. If a male fetus is identified, the woman can go on to definitive diagnosis of the condition after CVS from 11 weeks. If a female fetus is identified, an invasive test is not needed, and the associated miscarriage risk can be avoided.
Another key clinical indication for early fetal sex determination is for pregnancies at risk of conditions associated with ambiguous development of the external genitalia, such as congenital adrenal hyperplasia (CAH). Female fetuses with CAH require early maternal treatment with dexamethasone to reduce the degree of virilisation. Male fetuses can have CAH, but they are not at risk of genital virilisation. Consequently, if gender can be determined early in pregnancy, women with female fetuses can continue prenatal steroid treatment and go on to definitive genetic diagnosis if requested, whereas those with male fetuses can discontinue the unnecessary steroid treatment and avoid invasive testing.
Fetal sex determination using cffDNA can also be useful in the clarification of some fetal ultrasound findings. These include confirmation of genetic sex if abnormal genitalia are identified and additional information for diagnosing genetic conditions where genital ambiguity or sex reversal is a feature of the condition.
Fetal sex determination can be carried out using ultrasonography, which is highly accurate in the second and third trimesters. It can only be carried out reliably earlier in specialist centres and not before 12 weeks, as the morphological appearance of the male and female external genitalia is similar before 12 weeks. As CVS can be offered at 11 weeks, sex determination at 12 weeks and beyond is too late for those women who would like to have early definitive genetic diagnosis. In addition, where there is a risk of genital ambiguity, ultrasound cannot be used reliably at any gestation. Fetal sex determination using cffDNA is, therefore, a useful alternative with clear clinical benefits.
Test performance
The cffDNA test is carried out by establishing whether the Y chromosome is present or absent in the maternal plasma by targeting Y chromosome-specific sequences using RT-qPCR. If the Y chromosome is detected, the fetus must be male. If the Y chromosome sequence is not detected, it is assumed that the fetus is female. A recent systematic review and meta-analysis has drawn together 57 studies published since the late 1990s describing the analysis of cffDNA for fetal sex determination in 3524 male- and 3017 female-bearing pregnancies. This meta-analysis showed that the test was reliable between 7 and 12 weeks (94.8% sensitivity; 98.9% specificity), increasing to a sensitivity of 99% and specificity of 99.6% after 20 weeks. Testing before 7 weeks was unreliable. This finding correlates with the increasing proportion of cffDNA in maternal plasma that occurs as gestational age increases. The most common Y chromosome targets that have been assessed are the single copy SRY gene and the multicopy DYS14 sequence located within the TSPY gene, and no significant differences were found between the performance of tests using either of these targets.
Current laboratory procedures raise two key concerns. The first is the potential for false-negative results, as female fetuses are identified through a negative result that could also mean there was insufficient cffDNA present in the assay. The sensitivity and specificity of the current tests is high, but the reporting of female fetuses would benefit from confidence that cffDNA is present in the sample assayed. This issue will be overcome in the near future by the concurrent use of universal-free fetal DNA markers that will reveal the presence or absence of free-fetal DNA. The second concern is the failure rate of individual tests of 5% seen in many studies, which means a repeat test is required. The amount of cffDNA in the assay is crucial for reducing the number of inconclusive assays, and optimised sample collection and cffDNA extraction techniques will undoubtedly assist in reducing the number of inconclusive results.
Use of fetal sex determination in clinical practice
Fetal sex determination using cffDNA is now available as a clinical service in some countries, making it possible to demonstrate the reliability and utility of the test in clinical practice. In the UK in particular, the cffDNA test is well established. In 2010, it was the second most requested prenatal test (excluding rapid trisomy testing). In the recent UK audit of fetal sex determination, the most common indications for testing were X-linked disorders (81.2%, of which 20.8% were for haemophilia) and CAH (11.3%). The clinical utility of the test was demonstrated, as only 43% women at risk of X-linked conditions (excluding haemophilia) and 38% at risk of CAH subsequently underwent invasive testing.
In a Dutch study that considered attitudes to NIPD for fetal sex determination, both pregnant women and medical students supported the use of NIPD in the management of pregnancies at risk for early onset, sex-linked conditions. The use of NIPD for family balancing or late-onset conditions was not supported. In addition, a recent qualitative study that explored the views of 39 women (and partners) at risk of X-linked disorders and of CAH who had undergone fetal sex determination using cffDNA showed the positive attitudes of service users to cffDNA testing. Service users valued the opportunity to have a test that was early in pregnancy with no risk of miscarriage. The cffDNA test was clearly important to couples who had decided before embarking on any prenatal testing that they would persue invasive testing and termination. For other couples, the cffDNA test was a useful tool in the decision-making process, allowing them to make decisions one step at a time.
Fetal sex determination using cffDNA has been shown to be cost neutral for X-linked conditions such as DMD, for which diagnostic testing is commonly requested and also for CAH. Costs addressed included NIPD, CVS (sampling and molecular testing), scans, and pregnancy outcomes. Differences in mean costs per pregnancy for NIPD compared with invasive testing were small for both DMD and CAH. The costs of NIPD were offset by the smaller proportion of women who required CVS and, for CAH, the earlier cessation of unnecessary steroid treatment also contributed. Overall, NIPD was not associated with higher costs compared with invasive testing, which means women can have the clinical benefits of the test at no extra cost to health services.
Debate is ongoing about the clinical utility of using NIPD for fetal sex determination in pregnancies at risk of haemophilia, where it is important as active obstetric management is required to minimise birth-related trauma to confirmed and ‘at risk’ male neonates. As few women who are carriers of this X-linked condition go on to invasive testing with a view to terminating affected pregnancies, the usefulness of early determination of sex is debatable, as this could be carried out using ultrasound at the time of the routine fetal anomaly scan later in pregnancy. A recent qualitative study, involving interviews with 32 health professionals who offer prenatal care for women at risk of haemophilia, found that opinions are divided and practice is variable for how NIPD is offered for haemophilia around the UK. Further investigation into the value of this test for couples at risk of having a child with haemophilia and a formal cost analysis is required.
Offering cell-free fetal deoxyribonucleic acid testing for fetal sex determination
Overall, a number of key practice points must be considered when offering this test. Testing is only reliable after 7 weeks, so it is important that an accurate gestational age is established through ultrasound scanning before cffDNA testing. This scan should also be used to check for multiple pregnancies, as it is possible that false positives may result from the presence of a vanishing (male) twin with survival of a female. No test is 100% accurate, and discordant results do occur with NIPD. For this reason, it is recommended that ultrasound scanning after 12 weeks should be used to confirm the NIPD result. In addition, as inconclusive results do occur, parents need to be advised of the need for repeat testing.
Fetal sex determination
Fetal sex determination using cffDNA is a well-established prenatal test that can be carried out reliably from 7 weeks gestation using real-time quantitative PCR (RT-qPCR) to identify the presence or absence of Y chromosome-specific sequences in the maternal plasma. The most common clinical indication for early fetal sex determination is for carriers of X-linked genetic disorders, such as Duchenne muscular dystrophy (DMD) or adrenoleucodystrophy, where male-bearing pregnancies are primarily at risk. For women who are carriers of X-linked conditions, early fetal sex determination using cffDNA can inform the uptake of invasive testing. If a male fetus is identified, the woman can go on to definitive diagnosis of the condition after CVS from 11 weeks. If a female fetus is identified, an invasive test is not needed, and the associated miscarriage risk can be avoided.
Another key clinical indication for early fetal sex determination is for pregnancies at risk of conditions associated with ambiguous development of the external genitalia, such as congenital adrenal hyperplasia (CAH). Female fetuses with CAH require early maternal treatment with dexamethasone to reduce the degree of virilisation. Male fetuses can have CAH, but they are not at risk of genital virilisation. Consequently, if gender can be determined early in pregnancy, women with female fetuses can continue prenatal steroid treatment and go on to definitive genetic diagnosis if requested, whereas those with male fetuses can discontinue the unnecessary steroid treatment and avoid invasive testing.
Fetal sex determination using cffDNA can also be useful in the clarification of some fetal ultrasound findings. These include confirmation of genetic sex if abnormal genitalia are identified and additional information for diagnosing genetic conditions where genital ambiguity or sex reversal is a feature of the condition.
Fetal sex determination can be carried out using ultrasonography, which is highly accurate in the second and third trimesters. It can only be carried out reliably earlier in specialist centres and not before 12 weeks, as the morphological appearance of the male and female external genitalia is similar before 12 weeks. As CVS can be offered at 11 weeks, sex determination at 12 weeks and beyond is too late for those women who would like to have early definitive genetic diagnosis. In addition, where there is a risk of genital ambiguity, ultrasound cannot be used reliably at any gestation. Fetal sex determination using cffDNA is, therefore, a useful alternative with clear clinical benefits.
Test performance
The cffDNA test is carried out by establishing whether the Y chromosome is present or absent in the maternal plasma by targeting Y chromosome-specific sequences using RT-qPCR. If the Y chromosome is detected, the fetus must be male. If the Y chromosome sequence is not detected, it is assumed that the fetus is female. A recent systematic review and meta-analysis has drawn together 57 studies published since the late 1990s describing the analysis of cffDNA for fetal sex determination in 3524 male- and 3017 female-bearing pregnancies. This meta-analysis showed that the test was reliable between 7 and 12 weeks (94.8% sensitivity; 98.9% specificity), increasing to a sensitivity of 99% and specificity of 99.6% after 20 weeks. Testing before 7 weeks was unreliable. This finding correlates with the increasing proportion of cffDNA in maternal plasma that occurs as gestational age increases. The most common Y chromosome targets that have been assessed are the single copy SRY gene and the multicopy DYS14 sequence located within the TSPY gene, and no significant differences were found between the performance of tests using either of these targets.
Current laboratory procedures raise two key concerns. The first is the potential for false-negative results, as female fetuses are identified through a negative result that could also mean there was insufficient cffDNA present in the assay. The sensitivity and specificity of the current tests is high, but the reporting of female fetuses would benefit from confidence that cffDNA is present in the sample assayed. This issue will be overcome in the near future by the concurrent use of universal-free fetal DNA markers that will reveal the presence or absence of free-fetal DNA. The second concern is the failure rate of individual tests of 5% seen in many studies, which means a repeat test is required. The amount of cffDNA in the assay is crucial for reducing the number of inconclusive assays, and optimised sample collection and cffDNA extraction techniques will undoubtedly assist in reducing the number of inconclusive results.
Use of fetal sex determination in clinical practice
Fetal sex determination using cffDNA is now available as a clinical service in some countries, making it possible to demonstrate the reliability and utility of the test in clinical practice. In the UK in particular, the cffDNA test is well established. In 2010, it was the second most requested prenatal test (excluding rapid trisomy testing). In the recent UK audit of fetal sex determination, the most common indications for testing were X-linked disorders (81.2%, of which 20.8% were for haemophilia) and CAH (11.3%). The clinical utility of the test was demonstrated, as only 43% women at risk of X-linked conditions (excluding haemophilia) and 38% at risk of CAH subsequently underwent invasive testing.
In a Dutch study that considered attitudes to NIPD for fetal sex determination, both pregnant women and medical students supported the use of NIPD in the management of pregnancies at risk for early onset, sex-linked conditions. The use of NIPD for family balancing or late-onset conditions was not supported. In addition, a recent qualitative study that explored the views of 39 women (and partners) at risk of X-linked disorders and of CAH who had undergone fetal sex determination using cffDNA showed the positive attitudes of service users to cffDNA testing. Service users valued the opportunity to have a test that was early in pregnancy with no risk of miscarriage. The cffDNA test was clearly important to couples who had decided before embarking on any prenatal testing that they would persue invasive testing and termination. For other couples, the cffDNA test was a useful tool in the decision-making process, allowing them to make decisions one step at a time.
Fetal sex determination using cffDNA has been shown to be cost neutral for X-linked conditions such as DMD, for which diagnostic testing is commonly requested and also for CAH. Costs addressed included NIPD, CVS (sampling and molecular testing), scans, and pregnancy outcomes. Differences in mean costs per pregnancy for NIPD compared with invasive testing were small for both DMD and CAH. The costs of NIPD were offset by the smaller proportion of women who required CVS and, for CAH, the earlier cessation of unnecessary steroid treatment also contributed. Overall, NIPD was not associated with higher costs compared with invasive testing, which means women can have the clinical benefits of the test at no extra cost to health services.
Debate is ongoing about the clinical utility of using NIPD for fetal sex determination in pregnancies at risk of haemophilia, where it is important as active obstetric management is required to minimise birth-related trauma to confirmed and ‘at risk’ male neonates. As few women who are carriers of this X-linked condition go on to invasive testing with a view to terminating affected pregnancies, the usefulness of early determination of sex is debatable, as this could be carried out using ultrasound at the time of the routine fetal anomaly scan later in pregnancy. A recent qualitative study, involving interviews with 32 health professionals who offer prenatal care for women at risk of haemophilia, found that opinions are divided and practice is variable for how NIPD is offered for haemophilia around the UK. Further investigation into the value of this test for couples at risk of having a child with haemophilia and a formal cost analysis is required.
Offering cell-free fetal deoxyribonucleic acid testing for fetal sex determination
Overall, a number of key practice points must be considered when offering this test. Testing is only reliable after 7 weeks, so it is important that an accurate gestational age is established through ultrasound scanning before cffDNA testing. This scan should also be used to check for multiple pregnancies, as it is possible that false positives may result from the presence of a vanishing (male) twin with survival of a female. No test is 100% accurate, and discordant results do occur with NIPD. For this reason, it is recommended that ultrasound scanning after 12 weeks should be used to confirm the NIPD result. In addition, as inconclusive results do occur, parents need to be advised of the need for repeat testing.
Single-gene disorders
Prenatal diagnosis has seen a rapid increase in the number of disorders that can be tested for as a result of technological advances and research into the genetics underlying many conditions. In the 2009–2010 audit by the UK Clinical Molecular Genetics Society (CMGS), 1744 prenatal diagnoses were carried out in the UK for over 100 different monogenic diseases. Initial reports of NIPD for single-gene disorders were confined to the detection of autosomal dominant conditions that are paternally inherited or arise as a result of a de novo mutation; increasingly, however, new technologies and new approaches are also allowing us to apply NIPD to recessive conditions ( Table 1 ). The first use of cffDNA for detecting a single-gene disorder was for autosomal dominant disorder myotonic dystrophy. In this study, the paternally inherited CTG trinucleotide expansion in the DMPK gene was detected using PCR followed by gel electrophoresis. Non-invasive prenatal diagnosis has also been possible for other autosomal dominant disorders, with the successful diagnosis of cases of paternally inherited early onset primary dystonia and Huntington disease.
Study | Condition | Method | Total number of samples tested | Results |
---|---|---|---|---|
Autosomal dominant: paternally inherited mutation | ||||
Amicucci et al., 2000. | Myotonic dystrophy | PCR | 1 | One affected |
Meaney and Norbury, 2009 | Tortion dystonia | PCR | 2 | Two affected |
Gonzalez-Gonzalez et al., 2003 | Huntington disease | QF-PCR | 1 | One unaffected |
Bustamante-Aragones et al., 2008 | Huntington disease | QF-PCR | 4 | Two out of three affected One out of one unaffected |
Gonzalez-Gonzalez et al., 2008 | Huntington disease | QF-PCR | 1 | One unaffected |
Autosomal dominant: de novo mutation | ||||
Saito et al., 2000 | Achondroplasia | PCR-RFLP | 1 | One affected |
Chitty et al., 2011 | Achondroplasia | PCR-RFLP | 6 | Four affected Two unaffected |
Li et al., 2004 | Achondroplasia | PCR-RFLP plus QF-PCR | 1 | One affected |
Li et al., 2007 | Achondroplasia | Matrix-assisted laser desorption/ionisation-time of flight mass spectrometry plus single allele base extension reaction. | 2 | Two affected |
Au et al., 2011 | Apert Syndrome | Allele-specific real time PCR | 1 | One affected |
Raymond et al., 2010 | Apert syndrome | PCR-RFLP | 2 | One affected One unaffected |
Raymond et al., 2010 | Crouzon | PCR-RFLP | 1 | One unaffected |
Raymond et al., 2010 | Thanatophoric dysplasia type 1 (TD1) and type 2 (TD2) | PCR-RFLP | 2 | One out of one affected TD1 One affected TD2 |
Autosomal recessive: parents carrying different mutations | ||||
Chiu et al., 2002 | Congenital adrenal hyperplasia | Amplification refractory mutation system PCR | 1 | One unaffected |
Gonzalez-Gonzalez et al., 2002 | Cystic Fibrosis | PCR-RFLP | 1 | One affected |
Bustamante-Aragones et al., 2008 | Cysic Fibrosis | Single base primer extension (SnaPshot) | 3 | Two affected One unaffected |
Bustamante-Aragones et al., 2008 | Propionic acidaemia | Single base primer extension (SnaPshot); melt curve analysis. | 1 | One affected (positive using both methods) |
Bustamante-Aragones et al., 2008 | Leber Congenital Amaurosis | Denaturing high-performance liquid chromatography | 1 | One affected |
Galbiati et al., 2011 | β-thalassaemia | Co-amplification at lower denaturation temperature PCR | 35 | 10/21 affected Cd39 |
11/21 unaffected | ||||
12/14 affected IVSI-110 | ||||
2/14 unaffected. | ||||
Papasavva et al., 2008 | β-thalassaemia | Arrayed primer extension | 7 | Three inherited paternal mutation |
Three normal | ||||
One incorrect | ||||
Lo et al., 2010 | β-thalassaemia | Genome wide MPS and SPRT analysis | 1 | One carrier |
Autosomal recessive: parents carrying the same mutations | ||||
Lun et al., 2008 | β-thalassaemia | Relative mutation dosage using digital PCR | 10 | Five correctly classified |
One incorrect | ||||
Four unclassified | ||||
Barrett et al., 2012 | Sickle cell disorder | Relative mutation dosage using digital PCR | 65 | 52 correctly classified |
Seven incorrectly classified | ||||
Five unclassified | ||||
Sirichotiyakul et al., 2011 | α-thalassaemia | Real-time quantitative PCR | 158 | 61/62 affected |
X-linked | ||||
Tsui et al., 2011 | Haemophilia | Relative mutation dosage using digital PCR | 7 | Three out of three affected haemophilia A |
Four out of four affected haemophilia B |
Several studies have been published on the use of NIPD for achondroplasia. This condition is inherited in an autosomal dominant fashion, with most cases arising as a de novo mutation in the fibroblast growth factor receptor 3 gene. The mutation creates a restriction site, which can be detected using PCR followed by restriction fragment length polymorphism. Size fractionation followed by matrix-assisted laser desorption and ionisation time-of-flight mass spectrometry (MALDI-TOF MS) and single allele base extension reaction (SABER) was also successfully used for detecting fetuses carrying achondroplasia mutations. Several other conditions that arise as a result of de novo mutations have also proven amenable to non-invasive testing using PCR and restriction fragment length polymorphism, including Apert syndrome, thanatophoric dysplasia and Crouzon syndrome ( Table 1 ).
Non-invasive prenatal diagnosis for autosomal recessive or maternally transmitted autosomal dominant disorders is more complicated, owing to the difficulty of distinguishing between the maternal- and fetal-free DNA in maternal plasma. First attempts involved the exclusion of the paternal mutation in autosomal recessive conditions where the parents were carriers of different mutations ( Table 1 ). If a paternally identified allele can be detected, the fetus has a 50% risk of inheriting the disorder, and therefore invasive testing would be recommended. Alternatively, in the absence of a paternal allele, the fetus could at most be a carrier, and invasive testing would not be indicated. The first exclusion study was carried out for a couple who were carriers of CAH. Using polymorphic markers to show that the paternal allele had not been inherited, Chiu et al. were able to show that the fetus was not affected by CAH. Following this, several studies using a range of different technologies have been successful in detecting or excluding the paternal allele in recessive conditions where parents carry different mutations, including cystic fibrosis, propionic academia, Leber congenital amaurosis and beta-thalassaemia ( Table 1 ).
All of the above exclusion studies were based on determining the absence or presence of a paternal mutation. Notably, Papasavva et al. were able to extend this approach to allow the detection of paternal inheritance of beta-thalassaemia mutations, in which the parents carry either the same or different mutations. An arrayed primer extension microchip assay was used to detect single-nucleotide polymorphisms (SNPs) that could differentiate between the maternal and paternal alleles. Using this assay, the inherited paternal allele was correctly identified in six of seven families. A key limitation of this approach is the number of SNPs that are required for informativity, as only 68% of the 34 families studied had two or more informative SNPs. Also problematic is the necessity to genotype grandparents in order to link the parental alleles.
For recessive conditions where parents carry the same mutation, it is difficult to distinguish the maternally inherited fetal allele from the maternal alleles, and new approaches to NIPD are required. A quantitative approach known as relative mutation dosage (RMD) has been developed on the basis of digital PCR to allow non-invasive diagnosis where parents carry the same mutation. Digital PCR requires the dilution of template DNA to an average concentration of less than one molecule per well, and hundreds to thousands of replicates of a PCR reaction are analysed to allow high copy number counting and quantification of the initial molecules in the template DNA. Because most positive wells contain just one molecule, counting allows quantification of the initial molecules in the template DNA. If a woman is heterozygous and the fetus is also a heterozygote, it is expected that there will be an allelic balance between the wild type and normal alleles; if the fetus is homozygous for either the mutant or the wild type allele there will be an over-representation of one or the other. Sequential probability ratio testing (SPRT) is the statistical method used to determine whether the counts from the digital PCR are in allelic balance or whether there is an imbalance for the over-represented allele. This technique is dependent upon the knowledge of fractional fetal DNA concentration, which can be easily determined in male fetuses using sequences on the Y chromosome, but, in cases where there is a female fetus, are reliant on detection of paternally inherited SNPs.
RMD was first developed for beta-thalassaemia and haemoglobin E mutations. Five out of 10 cases tested were correctly classified (three beta-thalassaemia and two haemoglobin E cases); those that were not classified or were incorrectly classified contained less than 10% cffDNA. More recently, this approach has been used for sickle-cell anaemia. In this study, fractional fetal DNA concentrations for female fetuses were determined using informative insertion and deletion polymorphisms (indels). The sickle-cell genotype was correctly determined in 82% of male fetuses (37 out of 45) and 75% of female fetuses (15 out of 20) where the fractional fetal DNA concentration was greater than 7%. RMD has also been used to determine whether a fetus has inherited mutations for the X-linked disorder haemophilia. The principle of RMD in the case of X-linked disorders differs from that used in the case of the HBB gene mutations in that there are only two possible genotypes: mutant or wild-type. This test was successful for three carriers of haemophilia A and four carriers of haemophilia B. A similar principle to RMD was followed by Sirichotiyakul et al., who successfully used RT-qPCR for detecting homozygous alpha-thalassaemia-1 (Hb Bart’s) in 155 out of 158 pregnancies.
Massively parallel sequencing can also be used to identify single-gene disorders non-invasively. Lo et al. used genome-wide scanning to determine the inheritance of two different beta-thalassaemia alleles in a 12-week old fetus. Over 3.9 billion sequencing reads, providing 65-fold coverage of the genome, were aligned to the non-repeat-masked human genome. The father’s 4bp–CTTT mutation in codons 41/42 of HBB was easily detected. The mother carried the A>G mutation at position −28 of the HBB gene. With the use of SNP homozygous in the father and heterozygous in the mother located near to the mutation, a haplotype could be created, and relative haplotype dosage was used to determine whether there was an increased representation of the haplotype containing the mutation or the haplotype on the unaffected allele. It was found that the fetus was a carrier, having inherited the mutant allele from the father and the normal allele from the mother.
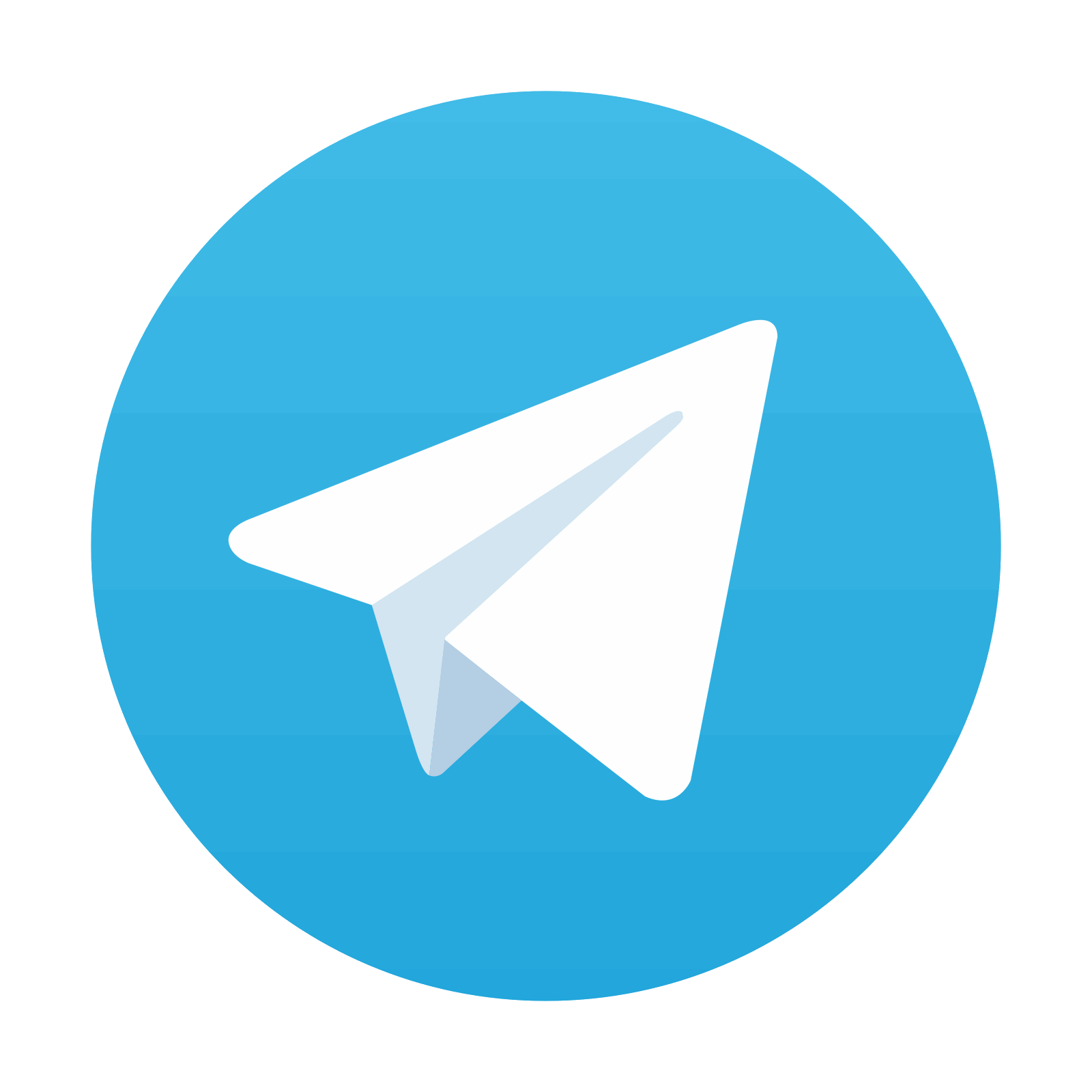
Stay updated, free articles. Join our Telegram channel

Full access? Get Clinical Tree
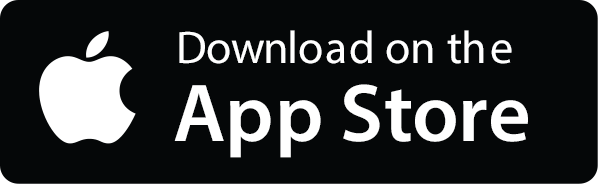
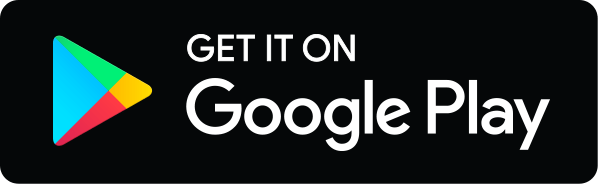