KEY POINTS
- 1.
Nearly half of all very low birth weight infants may have had brain injury due to hypoxia-ischemia, arterial ischemic stroke(s), inflammation, infection, and intraventricular hemorrhages (IVHs).
- 2.
Neuroimaging is increasingly seen in a “biomarker-like role,” where it can add to the information to develop and tailor the clinical developmental screening programs. Cranial ultrasound has been the modality of first choice to screen for brain injury because it enables the assessment of the temporal evolution of brain injury. Magnetic resonance imaging, with its noninvasive neuroimaging protocols, has defined the morphometric alterations in brain injury.
- 3.
White matter injury (WMI) is the most common type of brain injury in preterm infants, seen with or without IVH. WMI can been seen in several neuropathological patterns, including cystic WMI with macroscopic focal necrosis evolving to cysts and noncystic WMI with multiple focal necrotic areas evolving to glial scars and diffuse astrogliosis without focal necrosis.
- 4.
IVH is an important determinant of neurodevelopmental outcome. High-grade IVH that is seen in more than half of the ventricular volume or extending into the surrounding brain parenchyma is a reasonable predictor of neuromotor impairment including cerebral palsy.
- 5.
Cerebellar hemorrhages are frequently seen in infants with IVH or periventricular hemorrhagic infarctions, and even isolated lesions have been associated with cognitive, learning, and behavioral deficits impacting quality of life and daily function.
- 6.
Imaging abnormalities have been noted in severely ill neonates with multisystem organ failure, who are at risk of neurodevelopmental abnormalities. These infants may benefit from repeat imaging studies and early clinical intervention during follow-up.
Introduction
Advances in obstetric and neonatal care during the past 3 decades have significantly reduced mortality in very preterm (VP) infants. , However, up to 50% of the surviving very low birth weight infants (born with birth weights <1500 g) may have had brain injury, secondary to hypoxia-ischemia, arterial ischemic stroke(s), inflammation, infection, and intraventricular hemorrhages (IVHs) followed by posthemorrhagic ventricular dilatation (PHVD) and periventricular venous hemorrhagic infarction (PVHI). , Intracranial hemorrhage and white matter (WM) abnormalities are frequently seen in infants with earlier brain injury. Such neurologic damage can cause life-long disabilities such as epilepsy, cerebral palsy (CP), motor dysfunction, neurosensory impairment, cognitive and language impairment, behavioral disorders such as attention deficit-hyperactivity disorder (ADHD), autism-spectrum disorders (ASDs), and even increased medium- and long-term mortality. ,
Neuroimaging is increasingly seen in a “biomarker-like role,” where it can add to the information to develop and tailor clinical developmental screening programs and improve our ability to define the exact type and extent of brain injury a neonate may have endured. In this context, the advances in precision medicine may be further helpful; applied machine learning and computational data analysis, neurocritical monitoring, neuroimaging, and the modern omics technologies may help in developing individualized programs. This chapter is focused on current and ongoing advances in neuroimaging to expedite early diagnosis, measure structural brain damage, and track long-term neurodevelopmental outcomes. With continuing concerns about the short- and long-term impacts of radiation on the developing brain, it has been cranial ultrasound (cUS) and magnetic resonance imaging (MRI), not computed tomography, that have been used most frequently to image the neonatal brain. In the following sections, we first briefly introduce these two modalities; the remaining part of chapter is then organized based on the anatomic localization of the lesions.
Imaging of the Neonatal Central Nervous System
Cranial Ultrasound
cUS has been the modality of first choice to screen for brain injury in neonates since the 1970s because it enables temporal assessment of the evolution of brain injury and the overall growth and maturation of the central nervous system. , Advances in the resolution and image processing speed of cUS during the past decades have improved detection of WM abnormalities and have facilitated the diagnosis of cystic WM injury (WMI), the detection of cerebellar lesions and supratentorial WM cystic lesions, and the evolution of germinal matrix hemorrhage-intraventicular hemorrhage (GMH-IVH). Unfortunately, cUS cannot detect subtle lesions or diffuse gray matter (GM) and WMI. Fig. 95.1 shows the normal anatomy through coronal images from full-term (see Fig. 95.1A ), premature (see Fig. 95.1A ), and term sagittal views (see Fig. 95.1C ). Fig. 95.2 shows the images through the occipital horn, and Fig. 95.3 shows the images seen through the mastoid fontanelle at the level of the fourth ventricle.




MRI
MRI, with its noninvasive neuroimaging protocols, has defined the morphometric alterations in brain injury. It has also helped identify predictors of neurodevelopmental outcome. Standard T1- and T2-weighted images are helpful in reviewing the anatomy of the developing brain. Common neurologic conditions such as hypoxic-ischemic brain injury, perinatal stroke, IVH-PVHI, central nervous system infections, and congenital cerebral malformations are easily detected on standard sequences. Advanced techniques such as brain morphometry, diffusion MRI, volumetric MRI, magnetic resonance spectroscopy (MRS), inversion recovery/fluid attenuated inversion recovery (FLAIR), and functional MRI at term-equivalent age (TEA) assist with prediction of developmental abnormalities. Ultrafast/haste MRI sequences are useful for monitoring the progression of PHVD and to confirm shunt placement. Qualitative MRI helps with numerical measurements and enables construction of quantitative maps and complex brain images. MRI outlines the appropriate burden of ventriculomegaly through a detailed presentation of the third and fourth ventricles, posterior fossa structures, and fourth ventricle outflow tract obstruction. Quantitative MRI supports volumetric assessments (myelinated and unmyelinated WM, cortical and deep GM, brainstem, cerebellum, and cerebrospinal fluid [CSF]) where volume is recorded in volumetric pixels or voxels. Higher ventricle volumes were associated with decreased cognitive performance in preterm children with IVH and other brain injury. , Bora et al. reported that VP infants (born at 28–32 weeks) with ADHD had 4% less total cerebral volume and 36% more CSF than preterm comparators and 8% less total brain tissue and 144% more CSF volume compared with full-term children without attentional issues, even after adjusting for intracranial cavity volumes. Table 95.1 highlights the important variables assessed at TEA by MRI (adapted from Inder [2003], Woodward [2006], Nguyen [2009], Kidokoro [2013], and Walsh [2014]). Table 95.2 provides an overview of cUS and MRI as neuroimaging modalities (adapted from Sewell et al. [2018]).
Cerebral WM | Cysts ± signal abnormality, myelination of PLIC and corona radiata, size and morphology of corpus callosum (CC) and lateral ventricles, volume of periventricular WM |
Cortical GM | Signal abnormality, cortical fold maturation, size of extracerebral space |
Subcortical GM | Signal abnormality, symmetry and size of basal ganglia and thalamus |
Cerebellum | Signal abnormality, symmetry, and size of hemispheres |
Modality | Anatomic Areas Visualized | Advantages | Disadvantages | Clinical Application | Recent Advances |
---|---|---|---|---|---|
Cranial ultrasound (cUS) | |||||
1.Anterior fontanelle views in coronal and sagittal sections | Ventricular systems, periventricular white matter, deep gray matter |
|
|
| Improved resolution and image processing speed has improved detection of WM abnormalities associated with preterm brain injury |
2.Posterior fossa views through mastoid and posterior fontanelles | Cerebellar hemispheres, cerebellar vermis, cisterna magna, fourth ventricle | ||||
3.Color Doppler | Visualization of venous flow in transverse and sigmoid sinuses | ||||
Magnetic resonance imaging (MRI) | |||||
1.Standard T1- and T2- weighted imaging for qualitative assessment of abnormal anatomy or myelination |
|
|
|
| Quantitative MRI techniques predict neurodevelopmental delay, language, executive function, behavioral issues |
2.Advanced MRI techniques focused on quantitative analysis |
Types of Brain Injury in the Neonatal Period
Preterm WMI
WMI is the most common type of brain injury in preterm infants and is seen with or without IVH ( Fig. 95.4 ). Romero-Guzman et al. showed that the visible prevalence of preterm WMI (cystic and noncystic) was 14.7% on cUS and 32.8% on MRI. Prevalence increased with decreasing gestation: 39.6% in extremely preterm (EP) infants (<28 weeks), 27.4% in VP infants (<32 weeks), and 7.3% in infants <37 weeks. Known clinical risk factors were perinatal hypoxia, hypotension (ischemia), intrauterine infections, late onset bacteremia, and necrotizing enterocolitis. The neuropathological patterns of WMI were cystic WMI with macroscopic focal necrosis evolving to cysts, noncystic WMI with multiple focal necrotic areas evolving to glial scars, and diffuse astrogliosis without focal necrosis. , Although classic cystic WMI is associated with spastic bilateral CP, diffuse cerebral WMI is associated with cognitive impairment or issues with behavior, attention, social interaction, hearing, and visual impairment. The constellation of WMI and secondary trophic GM damage leads to altered cortical and thalamic development and is labeled encephalopathy of prematurity . Reduced cerebral cortical and deep gray nuclei volumes, delayed cortical folding, and abnormal myelination are frequently reported at term-equivalent MRI (TE-MRI) in preterm infants with WMI. Table 95.3 summarizes the common neuropathology patterns in preterm infants with brain injury. Impaired neurodevelopment at 2 years (cognitive delay in 23% and motor delay in 26%) may be seen in many preterm infants with normal cUS.

Neuroimaging Findings | Best Diagnostic Modality | Neuropathological Findings | Clinical Outcomes |
---|---|---|---|
Cystic WM abnormalities | cUS, MRI | Cystic PVL/cPVL (bilateral cysts) PVHI evolving to porencephalic cyst (usually unilateral) | Diplegic (RR, 5), hemiplegic (RR, 29), or quadriplegic (RR, 24) CP Location and size dependent (≥3 mm in parieto-occipital periventricular WM are highest risk) Hemiplegic CP with motor cortex involvement 10% with grade I PVL diagnosed with spastic diplegic CP by school age, ≈50% for those with cPVL Visual impairment with severe cPVL |
Gray matter abnormalities | MRI | Neuronal loss and GM gliosis Subcortical GM and cerebellar involvement | Cognitive impairment, behavioral issues |
Diffuse WM abnormalities | MRI | Diffuse cPVL (moderate-severe WM abnormality) Noncystic PVL (mild-moderate WM abnormality) Diffuse WM gliosis (normal-mild WM abnormality) | Cognitive impairment, behavioral issues Unclear |
GMH-IVH Posthemorrhagic ventricular dilatation (PHVD) | cUS, MRI | Rupture of germinal matrix vessels | Location and severity dependent, grade III/PVHI associated with quadriplegic (RR, 5.1), diplegic (RR, 2.3), or hemiplegic (RR, 5.8) CP, cognitive and behavioral issues, and blindness Bilateral IVH with PHVD needing a shunt is associated with the highest rates of CP (80% prevalence) 50%–80% with PHVI are diagnosed with intellectual disability and behavioral issues at school age Bilateral PVHI: worst cognitive outcomes, grade III IVH and PVHI; need for education support in reading, mathematics, and writing |
Punctate WM lesions | MRI | Ischemic lesion Hemorrhage/medullary venous congestion | Unclear, may have cognitive or behavioral issues |
Diffuse excessive high signal intensity (DEHSI) | MRI | Unknown | No association between DEHSI and abnormal neurodevelopment |
Encephalopathy of prematurity | MRI | PVL, neuronal/axonal loss | CP, autism, motor, cognitive, attention, behavioral, and social interaction issues |
Ventriculomegaly: moderate (1–1.5 cm), severe (>1.5 cm) | cUS, MRI | Gliosis, demyelination, axonal degeneration | Quadriplegic (RR, 17), hemiplegic (RR, 17), or diplegic (RR, 5.7) CP Moderate-severe ventriculomegaly: 2- to 3-fold increase in cognitive delay and low IQ |
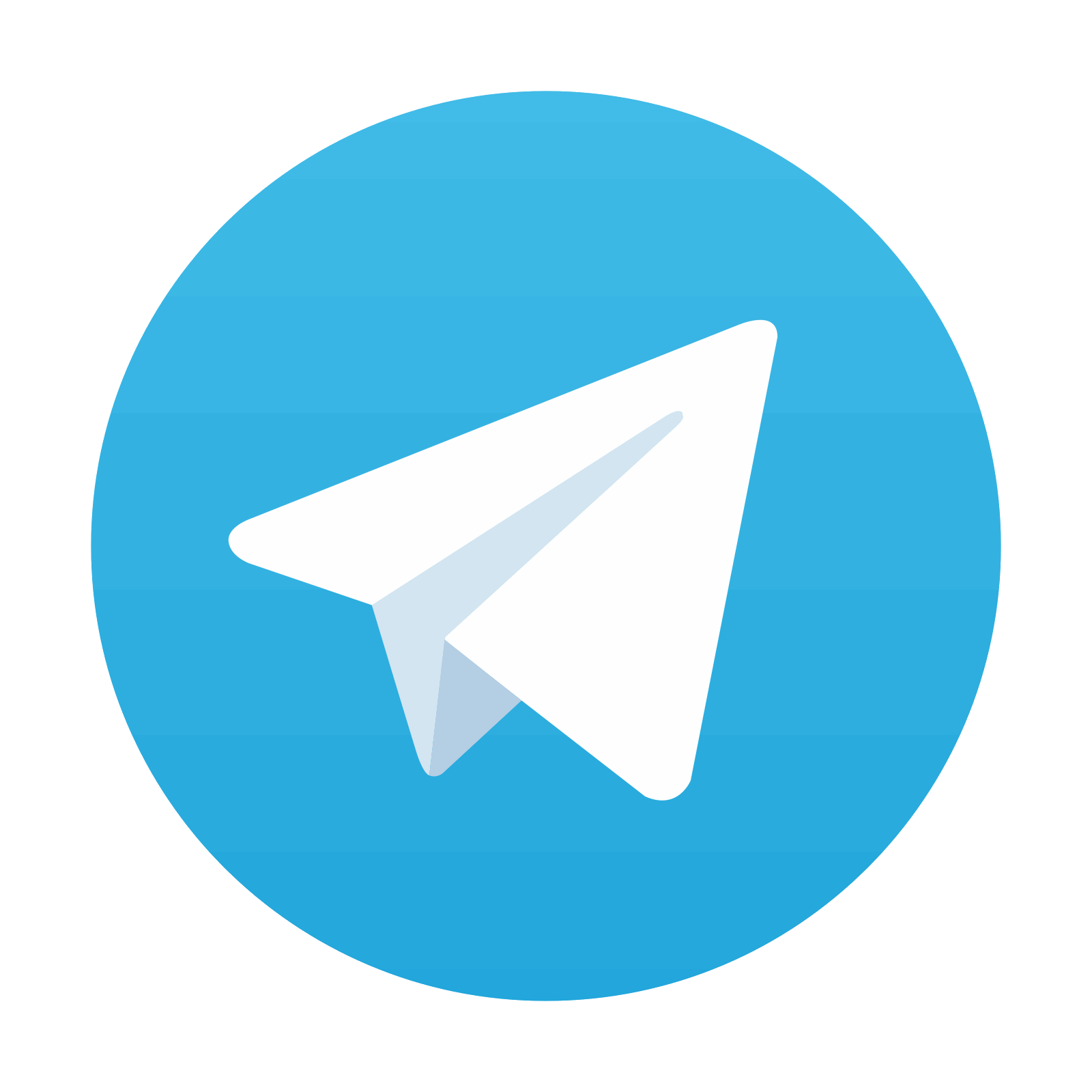
Stay updated, free articles. Join our Telegram channel

Full access? Get Clinical Tree
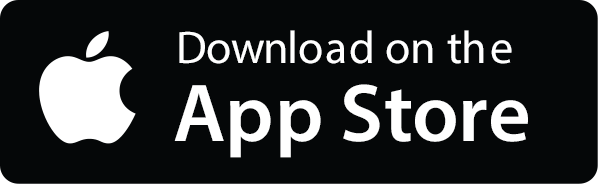
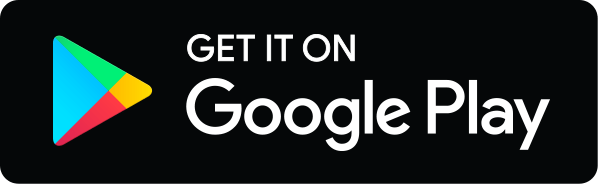
