The urogenital system is divided functionally into two different embryologically component parts: the urinary system and the genital system . The urogenital system includes all the organs involved in reproduction and forming and voiding urine. Embryologically, the systems are closely associated, especially during their early stages of development. The urogenital system develops from the intermediate mesenchyme (primordial embryonic connective tissue consisting of mesenchymal cells) derived from the dorsal body wall of the embryo ( Fig. 12.1 A and B ). The mesenchyme is primarily responsible for the formation of the kidneys and internal genitalia and their ducts.

During folding of the embryo in the horizontal plane, the mesenchyme is carried ventrally and loses its connection with the somites ( Fig. 12.1 B to D ). A longitudinal elevation of mesoderm, the urogenital ridge , forms on each side of the dorsal aorta ( Fig. 12.1 D and F ). The part of the ridge giving rise to the urinary system is the nephrogenic cord (see Fig. 12.1 D to F ); the part of the ridge giving rise to the genital system is the gonadal ridge (see Fig. 12.29 C ).
Expression of the following genes is needed for the formation of the urogenital ridge: Wilms tumor suppressor 1 (WT1), steroidogenic factor 1, and DAX1 .
Development of Urinary System
11
The urinary system begins to develop before the genital system and consists of the
- •
Kidneys , which produce and excrete urine
- •
Ureters , which convey urine from the kidneys to the urinary bladder
- •
Urinary bladder , which stores urine temporarily
- •
Urethra , which discharges urine from the bladder externally
Development of Kidneys and Ureters
Three sets of successive kidneys develop in the embryos . The first set, the pronephroi , is rudimentary. The second set, the mesonephroi , functions briefly during the early fetal period. The third set, the metanephroi , forms the permanent kidneys.
Pronephroi
Pronephroi are bilateral transitory structures that appear early in the fourth week. They are represented by a few cell clusters and tubular structures in the developing neck region ( Fig. 12.2 A ). The pronephric ducts run caudally and open into the cloaca , the chamber into which the hindgut and allantois emptied ( Fig. 12.2 B ). The pronephroi soon degenerate; however, most parts of the ducts persist and are used by the second set of kidneys.

Mesonephroi
Mesonephroi , which are large, elongated excretory organs, appear late in the fourth week, caudal to the pronephroi (see Fig. 12.2 ). The mesonephroi function as interim kidneys for approximately 4 weeks, until the permanent kidneys develop and function ( Fig. 12.3 ). The mesonephric kidneys consist of glomeruli (10 to 50 per kidney) and mesonephric tubules ( Figs. 12.4 and 12.5 , and see also Fig. 12.3 ). The tubules open into bilateral mesonephric ducts , which were originally the pronephric ducts. The mesonephric ducts open into the cloaca (see Fig. 12.2 B and Chapter 11 , Fig. 11.25 A ). The mesonephroi degenerate toward the end of week 12; however, the metanephric tubules become the efferent ductules of the testes . The mesonephric ducts have several adult derivatives in males ( Table 12.1 ).



Embryonic Structure | Female | Male |
---|---|---|
Indifferent gonad | Ovary | Testis |
Cortex | Ovarian follicles | Seminiferous tubules |
Medulla | Rete ovarii | Rete testis |
Gubernaculum | Ovarian ligament Round ligament of uterus | Gubernaculum testis |
Mesonephric tubules | Epoophoron | Efferent ductules of testis |
Paroophoron | Paradidymis | |
Mesonephric duct | Appendix vesiculosa | Appendix of epididymis |
Duct of epoophoron | Duct of epididymis | |
Longitudinal duct (Gartner duct) | Ductus deferens | |
Ejaculatory duct and seminal gland | ||
Stalk of ureteric bud | Ureter, pelvis, calices, and collecting tubules | Ureter, pelvis, calices, and collecting tubules |
Paramesonephric duct | Hydatid (of Morgagni) | Appendix of testis |
Uterine tube | ||
Uterus, Cervix | ||
Urogenital sinus | Urinary bladder | Urinary bladder |
Urethra | Urethra (except navicular fossa) | |
Vagina | Prostatic utricle | |
Urethral and paraurethral glands | Prostate | |
Greater vestibular glands | Bulbourethral glands | |
Sinus tubercle | Hymen | Seminal colliculus |
Primordial phallus | Clitoris | Penis |
Glans clitoris | Glans penis | |
Corpora cavernosa of clitoris | Corpora cavernosa of penis | |
Bulb of vestibule | Corpus spongiosum of penis | |
Urogenital folds | Labia minora | Ventral aspect of penis |
Labioscrotal swellings | Labia majora | Scrotum |
Metanephroi
Metanephroi , or the primordia of the permanent kidneys , begin to develop in the fifth week ( Fig. 12.6 ) and become functional approximately 4 weeks later. Urine formation continues throughout fetal life; the urine is excreted into the amniotic cavity and forms a component of the amniotic fluid. The kidneys develop from two sources (see Fig. 12.6 ):
- •
The ureteric bud (metanephric diverticulum)
- •
The metanephrogenic blastema (metanephric mass of mesenchyme)

The ureteric bud is a diverticulum (outgrowth) from the mesonephric duct near its entrance into the cloaca (see Fig. 12.6 A and B ). The metanephrogenic blastema is derived from the caudal part of the nephrogenic cord. As the ureteric bud elongates, it penetrates the blastema.
The stalk of the ureteric bud becomes the ureter (see Fig. 12.6 B ). The cranial part of the bud undergoes repetitive branching, resulting in the bud differentiating into the collecting tubules ( Fig. 12.7 A and B , and see also Fig. 12.6 E ). The first four generations of tubules enlarge and become confluent to form the major calices (see Fig. 12.6 C and D ). The second four generations coalesce to form the minor calices . The end of each arched collecting tubule induces clusters of mesenchymal cells in the metanephrogenic blastema to form small metanephric vesicles (see Fig. 12.7 A and B ). These vesicles elongate and become metanephric tubules (see Fig. 12.7 B and C ).

As branching occurs, some of the metanephric mesenchyme cells condense and form cap mesenchyme cells ; these undergo mesenchymal-to-epithelial transition and further develop into the majority of the nephron’s epithelium. The proximal ends of the tubules are invaginated by glomeruli . The tubules differentiate into proximal and distal convoluted tubules; the nephron loop (Henle loop) , together with the glomerulus and the glomerular capsule , constitute a nephron (see Fig. 12.7 D ).
Proliferation of the nephron progenitor cells and formation of the nephrons are dependent on BMP7- and Wnt-mediated signals ([Notch]/β-catenin signaling) . Each distal convoluted tubule contacts an arched collecting tubule, and the tubules become confluent. A uriniferous tubule consists of two embryologically different parts (see Figs. 12.6 and 12.7 ):
- •
A nephron derived from the metanephrogenic blastema
- •
A collecting tubule derived from the ureteric bud
Between the 10th and 18th weeks, the number of glomeruli increases gradually and then increases rapidly until the 36th week, when an upper limit is reached. Nephron formation is complete at birth, with each kidney containing between 200,000 and 2 million nephrons. The nephrons must last forever because no new nephrons are formed after this time and limited numbers may result in significant consequences for health in the child and adult. In some particular population groups (e.g., Aboriginal Australians) with lower numbers of nephrons developed in utero, there is also a higher incidence of adult chronic renal failure.
The fetal kidneys are subdivided into lobes ( Fig. 12.8 ). The lobulation usually disappears at the end of the first year of infancy as the nephrons increase and grow. The increase in kidney size after birth results mainly from elongation of the proximal convoluted tubules as well as an increase of interstitial tissue (see Fig. 12.7 D ). Nephron formation is complete at birth except in premature infants. Although glomerular filtration begins at approximately the ninth fetal week, functional maturation of the kidneys and increasing rates of filtration occur after birth.

Branching of the ureteric bud is dependent on induction by the metanephric mesenchyme. Differentiation of the nephrons depends on induction by the collecting tubules. The ureteric bud and the metanephrogenic blastema interact and induce each other, a process known as reciprocal induction , to form the permanent kidneys.
Molecular studies, especially knockout and transgenic analyses in the mouse, show that this process involves two principal signaling systems that use conserved molecular pathways. Recent research has provided insight into the complex interrelated molecular events regulating the development of the kidneys ( Fig. 12.9 ) . Before induction, a transcription factor, WT1, is expressed in the metanephrogenic blastema supporting the survival of the as yet uninduced mesenchyme. Expression of Pax2, Eya1, and Sall1 is required for the expression of glial-derived neurotropic factor (GDNF) in the metanephric mesenchyme. The transcription factors vHNF1 (HNF1β), Wnt1b, and GDNF play an essential role in the induction and branching of the ureteric bud (branching morphogenesis) . The receptor for GDNF, c-ret, is first expressed in the mesonephric duct but later becomes localized on the tip of the ureteric bud. Subsequent branching is controlled by transcription factors, including Emx2 and Pax2, and growth factor signals of the Wnt, FGF, and BMP families. Transformation of the metanephric mesenchyme to the epithelial cells of the nephron, mesenchymal−epithelial transition , is regulated by mesenchyme factors, especially Wnt4. Recent studies reveal that mutation of the angiotensin−type 2 receptor gene might account for kidney and urinary tract abnormalities.

Positional Changes of Kidneys
Initially, the primordial permanent kidneys lie close to each other in the pelvis, ventral to the sacrum ( Fig. 12.10 A ). As the abdomen and pelvis grow, the kidneys gradually relocate to the abdomen and move farther apart (see Fig. 12.10 B and C ). The kidneys attain their adult position during the beginning of the fetal period (see Fig. 12.10 D ). This “ascent” results mainly from the growth of the embryo’s body caudal to the kidneys. In effect, the caudal part of the embryo grows away from the kidneys so that they progressively occupy their normal position on either side of the vertebral column.

Initially the hilum of each kidney (depression on the medial border), where the blood vessels, ureter, and nerves enter and leave, faces ventrally; however, as the kidneys relocate, the hilum rotates medially almost 90 degrees. By the ninth week, the hila are directed anteromedially (see Fig. 12.10 C and D ). Eventually, the kidneys become retroperitoneal structures (external to the peritoneum) on the posterior abdominal wall. By this time, the kidneys are in contact with the suprarenal glands (see Fig. 12.10 D ).
Changes in Blood Supply of Kidneys
During the changes in the kidneys’ positions, the kidneys receive their blood supply from vessels that are close to them. Initially, the renal arteries are branches of the common iliac arteries (see Fig. 12.10 A and B ). Later, the kidneys receive their blood supply from the distal end of the abdominal aorta (see Fig. 12.10 B ). When the kidneys are located at a higher level, they receive new branches from the aorta (see Fig. 12.10 C and D ). Normally, the caudal branches of the renal vessels undergo involution and disappear.
The positions of the kidneys become fixed once the kidneys come into contact with the suprarenal glands in the ninth week. The kidneys receive their most cranial arterial branches from the abdominal aorta ; these branches become the permanent renal arteries . The right renal artery is longer and often in a more superior position than the left renal artery.
The common variations in the blood supply to the kidneys reflect the manner in which the blood supply continually changes during embryonic and early fetal life (see Fig. 12.10 ). Approximately 25% of adult kidneys have two to four renal arteries. Accessory (supernumerary) renal arteries usually arise from the aorta superior or inferior to the main renal artery and follow it to the hilum of the kidney ( Fig. 12.11 A , C , and D ). Accessory arteries may also enter the kidneys directly, usually via the superior or inferior pole (see Fig. 12.11 B ). An accessory artery to the inferior pole (polar renal artery) may cross anterior to the ureter and obstruct it, causing hydronephrosis , or distention of the renal pelvis and calices with urine. If the artery enters the inferior pole of the right kidney, it usually crosses anterior to the inferior vena cava and ureter.
Accessory renal arteries are end arteries; consequently, if an accessory artery is damaged or ligated, the part of the kidney supplied by it will become ischemic. Accessory arteries are approximately twice as common as accessory veins.
Some type of defect of the kidneys and ureters occurs in 3% to 4% of neonates. Defects in shape and position are most common. Many fetal urinary tract defects can be detected before birth by ultrasonography.
Renal Agenesis
Unilateral renal agenesis (absence) occurs approximately once in every 1000 neonates. Males are affected more often than females, and the left kidney is usually the one that is absent ( Figs. 12.12 A and B and 12.13A ). Unilateral renal agenesis often causes no symptoms and is usually not discovered during infancy because the other kidney usually undergoes compensatory hypertrophy and performs the function of the missing kidney. Unilateral renal agenesis should be suspected in infants with a single umbilical artery (see Chapter 7 , Fig. 7.18 ).
Bilateral renal agenesis (see Fig. 12.12 C ) is associated with oligohydramnios , a condition that develops because little or no urine is excreted into the amniotic cavity. This condition occurs approximately once in 3000 births and is incompatible with postnatal life. About 20% of cases of Potter syndrome are caused by bilateral renal agenesis. These infants have a characteristic facial appearance: the eyes are widely separated and have palpebronasal folds (epicanthic folds), the ears are low set, the nose is broad and flat, the chin is receding, and there are limb and respiratory anomalies. Infants with bilateral renal agenesis usually die shortly after birth from pulmonary hypoplasia leading to respiratory insufficiency.
Renal agenesis results when the ureteric buds do not develop or the primordia (stalks of buds) of the ureters degenerate. Failure of the buds to penetrate the metanephrogenic blastema results in failure of kidney development because no nephrons are induced by the collecting tubules to develop from the blastema. Renal agenesis probably has a multifactorial cause. There is clinical evidence that complete in utero involution of polycystic kidneys (many cysts) could lead to renal agenesis, with a ureter with a blind ending on the same side.
Malrotated Kidney
If a kidney fails to rotate, the hilum faces anteriorly; that is, the fetal kidney retains its embryonic position (see Figs. 12.10 A and 12.13 C ). If the hilum faces posteriorly, rotation of the kidney proceeded too far; if it faces laterally, lateral instead of medial rotation occurred. Abnormal rotation of the kidneys (malrotation) is often associated with ectopic kidneys.
Ectopic Kidneys
One or both kidneys may be in an abnormal position (see Fig. 12.13 B , E , and F ). Most ectopic kidneys are located in the pelvis ( Fig. 12.14 ), but some lie in the inferior part of the abdomen. Pelvic kidneys and other forms of ectopia result from failure of the kidneys to ascend. Pelvic kidneys are close to each other and usually fuse to form a discoid (pancake) kidney (see Fig. 12.13 E ). Ectopic kidneys receive their blood supply from blood vessels near them (internal or external iliac arteries and/or abdominal aorta). They are often supplied by several vessels. Sometimes a kidney crosses to the other side, resulting in crossed renal ectopia , and 90% of these kidneys are fused ( Fig. 12.15 ). An unusual type of abnormal kidney is unilateral fused kidney . In such cases, the developing kidneys fuse after they leave the pelvis, and one kidney attains its normal position, carrying the other kidney with it (see Fig. 12.13 D ).
Horseshoe Kidney
Horseshoe kidney is the most common renal fusion defect. In 0.2% of the population, the poles of the kidneys are fused; usually it is the inferior poles that fuse. The large U -shaped kidney usually lies in the pubic region, anterior to the inferior lumbar vertebrae ( Fig. 12.16 A ). In 60% of cases, the horseshoe kidney is found below the level of the inferior mesenteric artery or in the pelvis (see Fig. 12.16 B ).
A horseshoe kidney usually produces no symptoms because its collecting system develops normally and the ureters enter the bladder. If urinary flow is impeded, signs and symptoms of obstruction (urinary stones, hydronephrosis) and/or infection may appear. Approximately 7% of persons with Turner syndrome have horseshoe kidneys (see Figs. 20.3 and 20.4 ).
Duplications of Urinary Tract
Duplications of the abdominal part of the ureter and renal pelvis are common (see Fig. 12.13 F ). These defects result from abnormal division of the ureteric bud. Incomplete division results in a divided kidney with a bifid ureter (see Fig. 12.13 B ). Complete division results in a double kidney with a bifid ureter (see Fig. 12.13 C ) or separate ureters ( Fig. 12.17 ). A supernumerary kidney with its own ureter, which is rare, probably results from the formation of two ureteric buds (see Fig. 12.13 F ).
Ectopic Ureter
An ectopic ureter does not enter the urinary bladder. In males, the ectopic ureter will open into the neck of the bladder or the prostatic part of the urethra. The ureter may also enter the ductus deferens, prostatic utricle, or seminal gland. In females, the ectopic ureter may also open into the neck of the bladder or the urethra, vagina, or vestibule (cavity) of the vagina ( Fig. 12.18 ). Incontinence is the common complaint resulting from an ectopic ureter because urine flowing from the orifice of the ureter does not enter the bladder; instead it continually dribbles from the urethra in males and the urethra and/or vagina in females.
An ectopic ureter results when the ureter is not incorporated into the trigone between the openings of the ureters in the posterior part of the urinary bladder. Instead it is carried caudally with the mesonephric duct and is incorporated into the middle pelvic portion of the vesical part of the urogenital sinus. Because this part of the sinus becomes the prostatic urethra in males and urethra in females, the location of ectopic ureteric orifices is understandable. When two ureters form on one side (see Fig. 12.17 ), they usually open into the urinary bladder (see Fig. 12.13 F ).
Cystic Kidney Diseases
Autosomal dominant polycystic kidney disease (ADPKD) is the most common of all heritable cystic kidney diseases (1:500). Most commonly, PKD-1 and PKD-2 mutations are responsible; these encode for polycystin 1 and 2, respectively. These two molecules are mechanoreceptors localized to primary cilia of the kidney—they detect urine flow in the tubules. The main clinical findings in ADPKD are cysts involving < 5% of nephrons. These cysts can enlarge and reduce normal kidney function.
In autosomal recessive polycystic kidney disease (1 in 20,000 live births), diagnosed at birth or in utero by ultrasonography, both kidneys contain many small cysts ( Fig. 12.19 A ), which results in renal insufficiency . Death of the infant may occur shortly after birth, with 25% of cases associated with pulmonary hypoplasia; however, more than 80% of these infants are surviving beyond 1 year because of postnatal dialysis and kidney transplantation. Most cases have a mutation of the PKHD1 gene that results in polycystic kidney and congenital hepatic fibrosis.
Multicystic dysplastic kidney disease results from dysmorphology, abnormal development of the renal system (see Fig. 12.19 B ). The outcome for most children with this disease is generally good because the disease is unilateral in 75% of the cases. In this kidney disease, fewer cysts are seen than in autosomal recessive polycystic kidney disease, and they range in size from a few millimeters to many centimeters in the same kidney. It was thought that the cysts were the result of failure of the ureteric bud derivatives to join the tubules derived from the metanephrogenic blastema. It is now believed that the cystic structures are wide dilations of parts of the otherwise continuous nephrons, particularly the nephron loops (of Henle).









Development of Urinary Bladder
For descriptive purposes, the urogenital sinus is divided into three parts ( Fig. 12.20 C ):
- •
A vesical part that forms most of the urinary bladder and is continuous with the allantois
- •
A pelvic part that becomes the urethra in the neck of the bladder; the prostatic part of the urethra in males and the entire urethra in females
- •
A phallic part that grows toward the genital tubercle (primordium of the penis or clitoris; see Figs. 12.20 C and 12.37 )

The bladder develops mainly from the vesical part of the urogenital sinus (see Fig. 12.20 C ). The entire epithelium of the bladder is derived from the endoderm of the vesical part of the urogenital sinus , or ventral part of the cloaca (see Fig. 12.20 C ). The other layers of its wall develop from the adjacent splanchnic mesenchyme.
Initially, the bladder is continuous with the allantois , a fetal membrane developed from the hindgut (see Fig. 12.20 C ). The allantois soon constricts and becomes a thick fibrous cord, the urachus . It extends from the apex of the bladder to the umbilicus ( Fig. 12.21 , and see also Fig. 12.20 G and H ). In adults, the urachus is represented by the median umbilical ligament .

As the bladder enlarges, distal parts of the mesonephric ducts are incorporated into its dorsal wall (see Fig. 12.20 B to H ). These ducts contribute to the formation of the connective tissue in the trigone of the bladder . As these ducts are absorbed, the ureters open separately into the urinary bladder (see Fig. 12.20 C to H ). Partly because of traction exerted by the kidneys as they ascend, the orifices of the ureters move superolaterally and enter obliquely through the base of the bladder (see Fig. 12.20 F ). In males, the orifices of the ducts move close together and enter the prostatic part of the urethra as the caudal ends of the ducts develop into the ejaculatory ducts (see Fig. 12.33 A ). In females, the distal ends of the mesonephric ducts degenerate (see Fig. 12.33 B ).
In infants and children, the urinary bladder, even when empty, is in the abdomen. It begins to enter the greater pelvis at approximately 6 years of age; however, the bladder does not enter the lesser pelvis and become a pelvic organ until after puberty. The apex of the bladder in adults is continuous with the median umbilical ligament , which extends posteriorly along the posterior surface of the anterior abdominal wall.
In infants, a remnant of the urachal lumen may persist in the inferior part of the urachus. In approximately 50% of cases, the lumen is continuous with the cavity of the bladder. Remnants of the epithelial lining of the urachus may give rise to urachal cysts ( Fig. 12.22 A ), which are not usually detected unless the cysts become infected and enlarged. The patent inferior end of the urachus may dilate to form a urachal sinus that opens into the bladder. The lumen in the superior part of the urachus may also remain patent and form a urachal sinus that opens at the umbilicus (see Fig. 12.22 B ). Very rarely, the entire urachus remains patent and forms a urachal fistula that allows urine to escape from its umbilical orifice (see Fig. 12.22 C ).
A pathologically large urinary bladder, megacystis (megalocystis) , may result from a congenital disorder of the ureteric bud, which in turn can dilate the renal pelvis. The large bladder may also result from posterior urethral valves ( Fig. 12.23 ). Many infants with megacystitis suffer from renal failure in early childhood.
Exstrophy of the bladder, a severe birth defect, occurs approximately once in every 30,000 to 50,000 births. Exstrophy (eversion) of the bladder usually occurs in males ( Fig. 12.24 ). Exposure and protrusion of the mucosal surface of the posterior wall of the bladder characterize this defect. The trigone of the bladder and ureteric orifices are exposed, and urine dribbles intermittently from the everted bladder.
Exstrophy of the bladder, a deficiency of the anterior abdominal wall, is caused by incomplete median closure of the inferior part of the wall ( Fig. 12.25 ). The defect involves both the abdominal wall and the anterior wall of the urinary bladder. The defect results from failure of mesoderm to migrate between the ectoderm and endoderm of the abdominal wall (see Fig. 12.25 B and C ). As a result, the inferior parts of the rectus muscles are absent, and the external and internal oblique and transversus abdominis muscles are deficient.
No muscle or connective tissue forms in the anterior abdominal wall over the urinary bladder. Rupture of the cloacal membrane results in wide communication between the exterior and the mucous membrane of the bladder. Rupture of the cloacal membrane before connection of the urogenital septum results in exstrophy of the cloaca , resulting in exposure of the posterior wall of the bladder ( Fig. 12.25 F ) and hindgut.
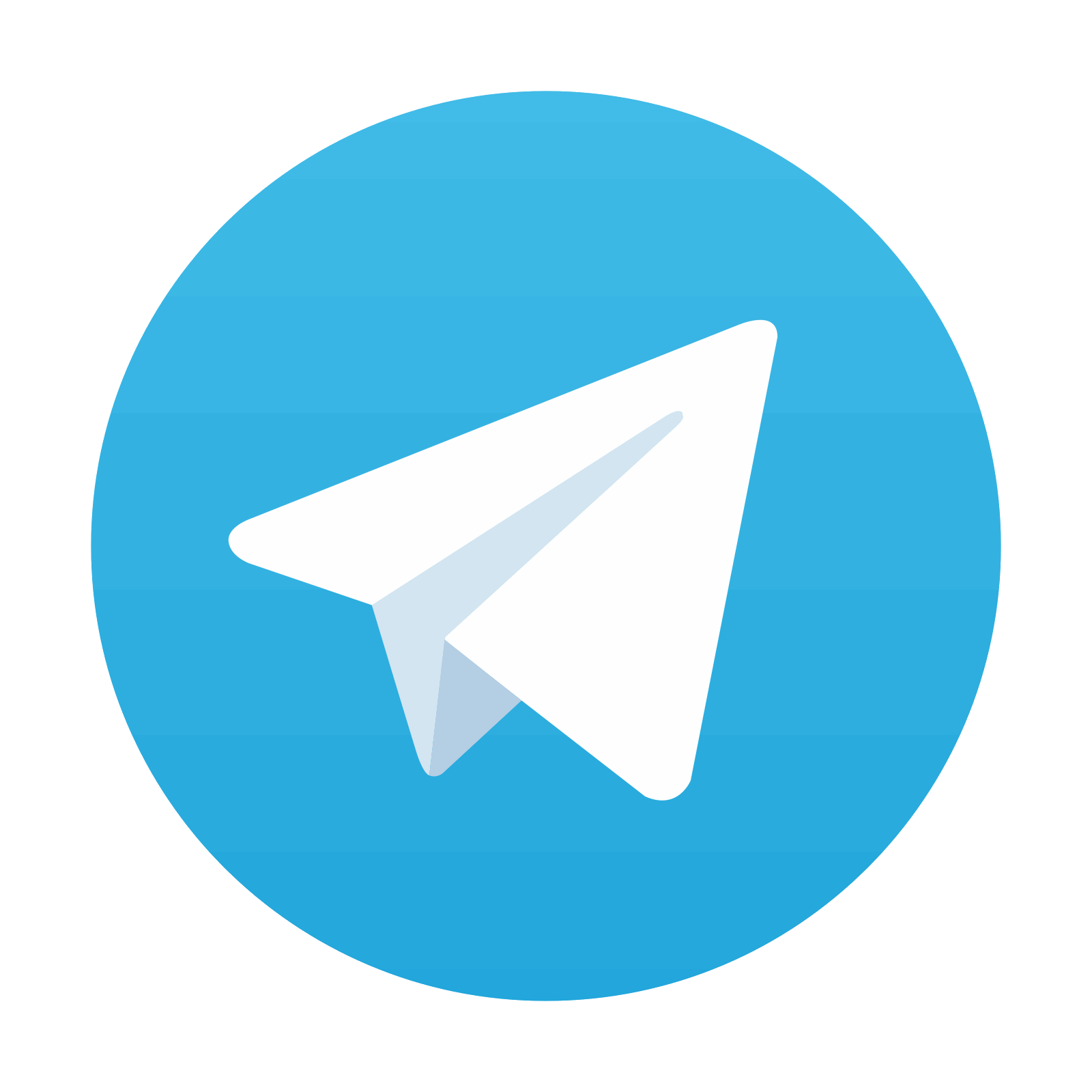
Stay updated, free articles. Join our Telegram channel

Full access? Get Clinical Tree
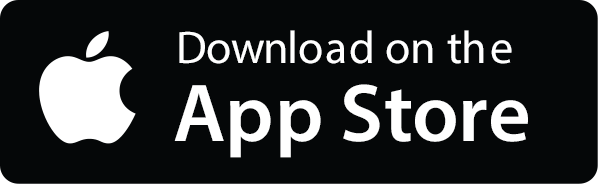
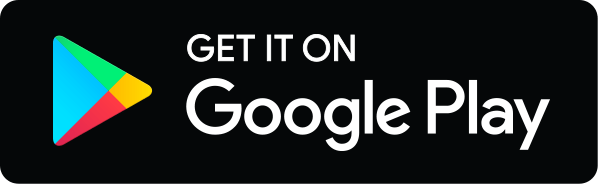
