Point-of-care ultrasound (POCUS) refers to non-radiologist clinicians who perform an ultrasound during a clinical encounter with their patient. Rather than performing a comprehensive exam of an organ or body part, the POCUS sonographer uses the ultrasound to answer a specific, focused clinical question, or as an aid in performing a procedure. Ultrasound delivers no ionizing radiation and usually does not require sedation; additionally, POCUS delivers immediate results at the point of care.
POCUS has been used in adult emergency medicine for over 20 years, and is making its way into pediatric emergency rooms and critical care settings. While many pediatric hospitalists are familiar with procedural ultrasound, diagnostic POCUS is relatively new to the field. The purpose of this chapter is to familiarize hospitalists with POCUS in general, including basic physics and use of the machine as well as review indications that have been used or show promise for future use in pediatric medicine.
Developing a productive and sustainable pediatric hospital medicine POCUS program requires significant expertise, resources, and time. No standards for credentialing and quality assurance exist for pediatric hospitalists, yet both are fundamental. Training standards have been published by the American College of Radiology and the American Institute of Ultrasound in Medicine, but pediatric emergency medicine physicians have found consensus guidelines from the American College of Emergency Physicians more geared to the focused POCUS exam, and therefore more applicable to them.1 Programs should establish minimum numbers of exams and didactic hours, and work with the hospital’s credentialing committee. Partnerships with radiology can form the basis for quality assurance and aid in credentialing. Local pediatric or adult emergency medicine programs may have expertise in purchasing and maintaining appropriate portable equipment, and often set the standard in training, monitoring, and clinical use of POCUS.
The point person for the program needs to be well trained in ultrasonography, understand the goals of POCUS, and be able to instruct and evaluate other clinicians as well as set competency standards. Evidence of improved patient outcomes should dictate which specific examinations are taught and performed. Each program must decide which examinations suit their particular needs, but there is no substitute for routine, frequent use with expert feedback in building competency.
Although advances in ultrasound technology have produced more affordable portable machines with excellent image quality, the purchase and maintenance of equipment is expensive. Leaders of the ultrasound program must consider multiple factors before meeting with vendors. Machine choice depends on specific examinations considered in the initial training (and examinations that may be added later), the sophistication of the user, power supply, size and maneuverability of the unit and its stand (which depends on location of use and storage), interfaces with hospital radiology systems and the internet, durability, costs, and service package. Service packages must be scrutinized, including the speed of replacing parts, and how software updates are handled. Programs may want to start with a limited number of machines to gain experience before full purchase. While sophisticated bells and whistles may be appealing for the technically savvy, complicated machines may intimidate the novice, and may not add value depending on the goals of the program.
Probes (transducers) are defined by their footprint and frequency (Figure 186-1). The footprint is the part of the probe that touches the patient’s skin and sends out the ultrasonic waves. A wide footprint gives a wider field of view but may not fit into small spaces, e.g. intercostals. High-frequency probes have better spatial resolution, yielding better image quality but less penetration. Low-frequency probes have better penetration and therefore can image deeper structures, but have less resolution. A program that uses the ultrasound solely for procedures only needs a high-frequency probe. Most programs will need at least two probes for each machine, depending on the structures imaged.
Ultrasound is a sound wave that is beyond the audible frequency range of the human ear, >20,000 Hz, but ultrasound for medical use usually ranges from 2 to 15 MHz. Electricity causes crystals in the probe head to vibrate (the piezoelectric effect). This energy is transmitted through the medium as a longitudinal wave, with particles in the medium vibrating back and forth in the direction of the wave. Properties of the medium—stiffness and density—determine the velocity of the wave. Reflected waves return to the transducer and make the crystals vibrate, inducing an electric current which is translated into images on the screen. The strength and timing of the returning waves is translated into color (gray scale) and position on the screen, respectively. Dense tissues (bone, diaphragm) are highly reflective, or echogenic, and appear white on the screen, while less dense tissues (fluid) are anechoic and appear black. Different shades of gray denote the in-between structures (liver, spleen). Air is the enemy of ultrasound, as it scatters the sound waves, hiding the structures beneath it.
While the user will have to learn how to turn the machine on and off, input patient information, attach the probes, maximize image quality, switch among scanning modes, save the image, and send the image somewhere for review or documentation, only maximizing image quality is discussed in this section. Most units have presets, which optimize image quality for a particular exam, e.g. cardiac, renal, pediatric, but adjustments are needed to achieve the best image. The most important controls are frequency, gain (and time gain compensation), focus, and depth.
Choose the highest frequency probe that allows visualization of the structure (deeper structures require lower frequencies) and if the probe has a frequency range, adjust to maximize resolution while maintaining adequate depth.
The gain is controlled by the amplifier in the unit. Increasing the gain makes the signals appear brighter, and decreasing the gain makes them darker. The image should be bright enough to see weak signals, but not so bright as to obscure subtle areas of altered echo texture. The time gain compensation (also called depth gain compensation because the longer a wave takes to return, the farther or deeper it is) allows one to adjust the gain at specified depths without making other parts of the screen too bright or too dark.
The depth of the focus can be adjusted manually. The focus is where the ultrasound beams converge and is the region where the best image quality is obtained.
The depth is the maximum depth of the structures displayed on the screen. Decreasing the depth not only makes the shallower structures bigger on the screen, it also increases the temporal resolution (the ability to see events occurring closely in time, such as fetal heart movements).
For most imaging the sonographer stands to the right of the patient with the probe in his right hand, and adjusts the machine with his left hand. The probe has a probe marker (dot, ridge, light) on its lateral edge, which corresponds to a dot or logo on the left side of the screen. By convention, the probe marker points to the right side of the patient when scanning transversely (Figure 186-2) and cephalad when scanning longitudinally (also referred to as sagittally) (Figure 186-3). Everything is reversed in cardiac imaging. The sonographer stands to the left of the patient, the probe marker points to the left of the patient, and the mark on the screen is on the right. Since both markers are reversed, the picture looks the same. Basically what is next to the marker on the probe is next to the marker on the screen. The top of the screen corresponds to where the probe is placed on the patient (skin), and the bottom is the opposite from that (think of the waves penetrating through the body). In a typical transverse orientation, the left side of the screen represents the right side of the patient and is similar to a CT scan. In a typical longitudinal orientation, left to right on the screen corresponds to cephalad to caudad on the patient.
The most common imaging modality is B-mode (brightness), which presents a real-time two-dimensional image as described in the orientation section above. M-mode (motion) is obtained through one slice of tissue such as a heart valve; the position of the border of a structure (its distance from the probe) is plotted vertically on the screen, with the horizontal axis representing time. This allows velocity to be deduced. Although there are a few forms of Doppler ultrasound (pulsed wave, continuous wave, color), basically Doppler ultrasound takes advantage of the frequency shift of moving objects and can detect velocity. In color Doppler, objects (e.g. blood) of increasing velocity are displayed in increasing intensities of red as they move toward the probe and increasing intensities of blue as they move away from the probe. Note that the colors have nothing to do with oxygenation. Also note that the chosen colors are opposite of the spectral absorption lines observed in astronomy (i.e. red-shifted stars are moving away from us).
The focused assessment by sonography in trauma (FAST) exam was one of the first applications of POCUS in the emergency room. The original focus of the FAST exam was detection of blood in the abdomen, but this evolved to include the heart. The four primary views are the right upper quadrant, left upper quadrant, subxiphoid, and suprapubic. Ideally, the exam takes less than 1 to 2 minutes. Both abdominal and cardiac probes can be used—the small footprint of the cardiac probe is helpful when visualization between ribs is necessary; the lower frequency abdominal probe is useful when visualizing obese patients where depth is needed.
Fresh blood appears anechoic (black) on ultrasound, and clotted blood appears gray. Morrison’s pouch, the hepatorenal recess, is the most common place for intra-abdominal blood to collect.
A sagittal view is obtained by placing the probe either in the midaxillary line on the lower rib cage or below the right costal margin. The probe may need to be moved laterally or posteriorly to avoid gas in the hepatic flexure. Observe Morrison’s pouch. Scan for black fluid in potential spaces. Observe the right costophrenic angle (Figure 186-4 and Figure 186-5).
Place the probe under the xiphoid almost parallel with the skin surface, directed toward the patient’s left shoulder. Ideally, the probe’s lateral surface makes an angle of 15 degrees with the skin (Figure 186-6). The left lobe of the liver and all four chambers of the heart are viewed. Observe for black fluid around the heart. The parasternal long axis view may be used when the subxiphoid view is not definitive (see the Cardiac section). Consider a pneumothorax if cardiac images cannot be obtained.
This view may be difficult to obtain. Place the probe parallel with the ribs in the mid or posterior axillary line. Scan potential spaces between diaphragm and spleen and spleen and kidney for free fluid (Figure 186-7).
FIGURE 186-7.
Left upper quadrant view with blood.

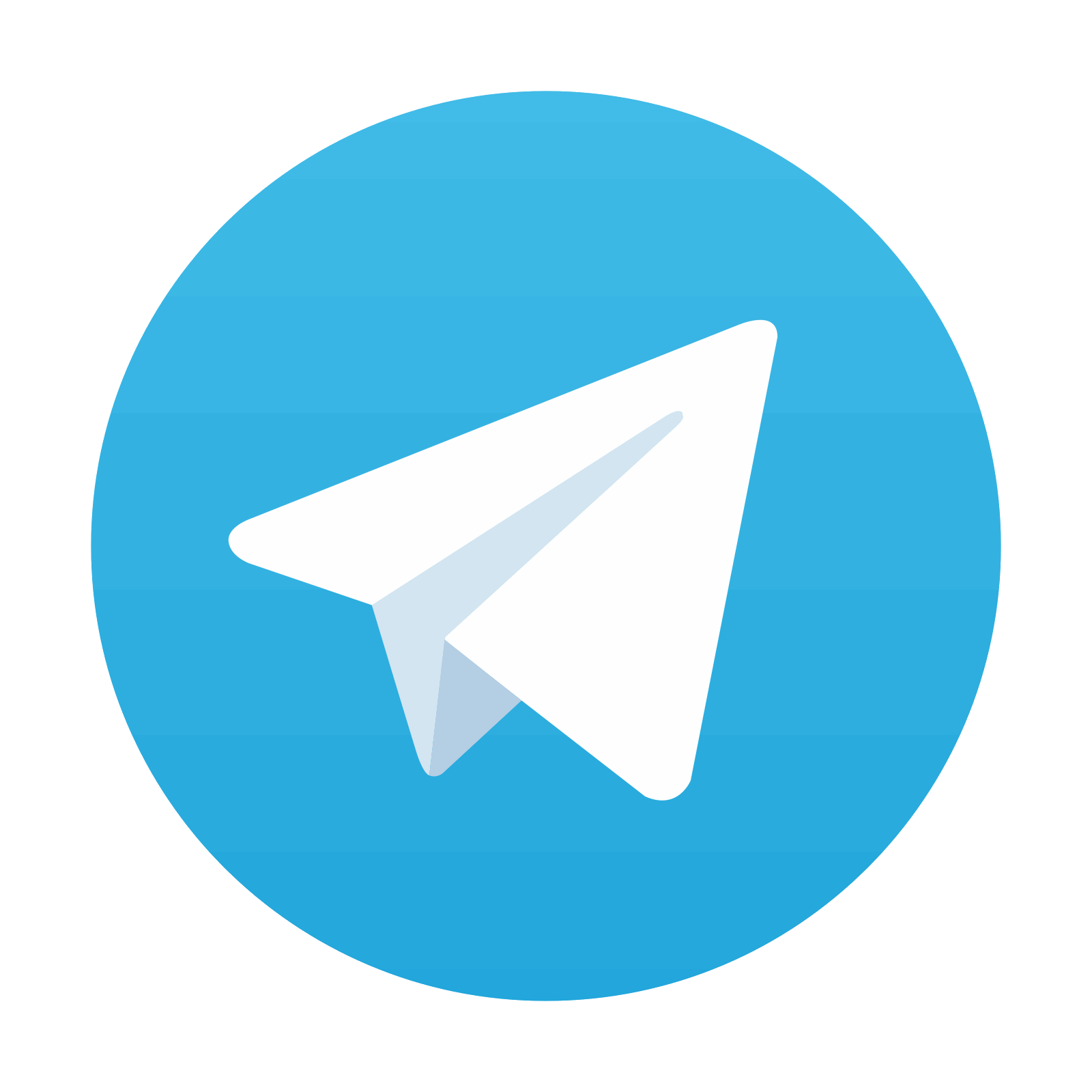
Stay updated, free articles. Join our Telegram channel

Full access? Get Clinical Tree
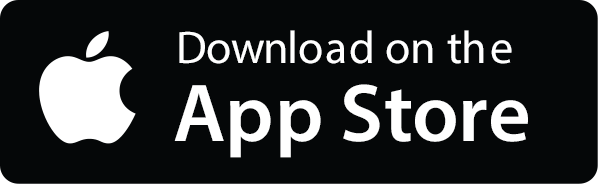
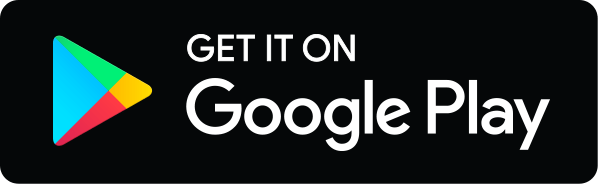
