Objective
We aimed to induce neural stem (NSC) and progenitor cells (NPC) from human placental tissues.
Study Design
Placental stem cells from first-trimester placental chorionic villi and term chorion were isolated. Neural differentiation was initiated with plating on collagen, retinoic acid, and/or human brain-derived neurotrophic factor and epidermal and fibroblast growth factor. Differentiation into neurons, oligodendrocytes, and astrocytes was monitored by immunohistochemistry. Two-dimensional polyacrylamide gel electrophoresis, high-performance liquid chromatography, and tandem mass spectrometry were used to identify proteins involved in the differentiation.
Results
Differentiated cells were mostly immediately postmitotic with some more but not fully mature postmitotic neurons. Neurons had dopaminergic or serotonergic character. Some cells differentiated into predominantly immature oligodendrocytes. Upon differentiation, neuron-specific proteins were up-regulated, whereas placental proteins were reduced.
Conclusion
Stem cells derived from human placenta can be differentiated into neural progenitors.
Brain injury in the premature infant is the most serious problem in perinatal medicine and includes a variety of neuropathologic lesions. The great majority of neonatal brain damage cases are an acquired form of neuronal injury. Three major etiologies have been described: hypoxia-ischemia (eg, perinatal asphyxia), maternal-fetal infection, and excitotoxicity. Additional risk factors for the development of periventricular white matter injury (PWMI) include prematurity, bradycardia, intrauterine growth retardation, and preeclampsia.
Most preterm births are due to spontaneous labor or preterm premature rupture of membranes, often mediated by ascending infection and subsequent chorioamnionitis. PWMI is a major cause for severe neurological impairment after preterm birth. The pathology ranges from focal cystic necrotic lesions (periventricular leukomalacia [PVL]) to diffuse myelination disturbances (diffuse PWMI). The incidence of PVL is low (<5% of all cases with perinatal brain damage), whereas focal or diffuse noncystic white matter injury is the predominant form. Neurological deficits of children affected by PWMI range from mild developmental disabilities to severe cerebral palsy. Oligodendrocyte progenitors are extremely vulnerable during a hypoxia-ischemia insult. Infants with extreme low birthweight have a reduced cortical volume, probably because of neuronal cell death.
Stem cells have been considered as grafts for neural cell replacement in neurodegenerative diseases. Stem cells are undifferentiated cells, capable of long-term self-renewal and multilineage differentiation. Candidate donor cells include embryonic stem cell (ESC)–derived neural progenitors and fetal or adult neural stem cells (NSCs) and neural progenitor cells (NPCs).
ESCs are derived from the inner cell mass of the blastocyst, the stage of embryonic development prior to implantation into the uterine wall. They have the capability of long-term self-renewal and are pluripotent; that is, they can be differentiated into cell types from all 3 germ layers (endoderm, mesoderm, ectoderm).
NSCs typically grow in vitro as free-floating aggregates (neurospheres) in response to growth factors like epidermal growth factor (EGF) and basic fibroblast growth factor (FGF)-2. Neurospheres contain multipotent stem cell-like and more restricted progenitor cell populations at different stages of differentiation. These cells can be differentiated in vitro into neurons, oligodendrocytes, and astrocytes.
Clinical use of fetal brain and postmortem adult brain tissue is problematic because of ethical concerns. There is a growing number of ESC lines available, yet the choice of ESC lines is limited. In addition to the ethical concerns involved in ESC-based therapy, tumor (teratoma) formation after transplantation of undifferentiated ESCs represents a major concern. Whereas undifferentiated ESCs have been reported to be nonimmunogenic, differentiated human ESCs were shown to express human leucocyte antigens that might cause graft rejection. Per definition, ESCs can not serve as an autologous cell source.
Alternatively, recent studies have suggested that mesenchymal stem cells (MSCs) from adult and fetal tissues have the potential to differentiate into neural cells. Sources for MSC include bone marrow, peripheral blood, umbilical cord blood, amniotic fluid, and Wharton’s jelly. The use of placental tissue and fetal membranes (amnion or chorion) was suggested as an abundant, ethically acceptable and easily accessible source of cells with neurogenic differentiation potential for autologous transplantation for peripartum neuroregeneration. A major advantage of this autologous cell source is the lack of graft rejection.
Amnion-derived cells have been isolated and differentiated into multiple lineages, including neural lines and their engraftment and neuroprotection confirmed in animal transplantation models. However, in preterm delivery, the amniotic membrane is typically the tissue in which the underlying infection is located. It might therefore be advantageous to use other placenta-derived tissue as a source for cellular grafts. There is little experience with chorion-derived stem cells.
The present study focused on purifying and expanding NSCs and NPCs from MSCs from first-trimester placenta or term chorion to obtain a cellular graft for neuroregeneration in perinatal brain damage. We show that placenta-derived MSCs contain a subpopulation of cells that can be differentiated into neural cells. This differentiation process is accompanied by changes in gene and protein expression.
Materials and Methods
Isolation and culture of placenta-derived stem cells
We isolated MSCs from the stromal layer of third-trimester chorion (chorion MSC) and from the chorionic villous stroma of first-trimester placenta (villous stroma MSC) as described previously. First-trimester (7-14 weeks of gestation) tissue was obtained from aborted fetuses of healthy donors. Third-trimester (34-39 weeks of gestation) tissue was collected after cesarean sections of healthy donors. Tissue was obtained after informed consent. The local institutional review board approved all experiments.
Preinduction/preselection for the neural lineage
Preinduction and preselection of expanded placenta-derived stem cells was performed at passages 2-5. Three neural differentiation protocols were used ( Figure 1 ).

Method A: preinduction of placental stem cells into neurosphere-like bodies
Cells were dissociated with 0.05% Trypsin/0.53 mM EDTA (Invitrogen, Carlsbad CA) and cultured (1.5-3 × 10 5 cells/cm 2) in low-attachment tissue culture plates (NUNC, Rochester NY) in neurobasal medium (2% B27 supplement, 2 mM Glutamax-I, antibiotic/antimycotic solution; Invitrogen; 20 ng/mL human EGF (hEGF), 20 ng/mL human FGF-2 (hFGF-2); BD Biosciences, Franklin Lakes NJ; 2 μg/mL heparin; Fluka/Sigma-Aldrich, Buchs, Switzerland). Spheres (150-200 μM diameter) were passaged by mechanical trituration (50× with a 2 mL plastic pipette; 4 passages).
Method B
Placenta stem cells were cultured for 12-14 days in adherent conditions (α-minimal essential medium, 20% fetal bovine serum [FBS]; 10 ng/mL FGF-2, 10 ng/mL EGF, antibiotic/antimycotic solution).
Method C
Placenta stem cells (1.5 × 10 7 cells) were enriched for stage-specific embryonic antigen-4 (SSEA-4)-positive cells: The mouse antihuman SSEA-4 antibody (3.0 μg/mL; Chemicon, Temecula, CA) was added for 30 min, the cells washed 3 times with phosphate-buffered saline (PBS; without Ca2+/Mg2+, 2% fetal bovine serum [FBS], Invitrogen; 1 mM EDTA) and incubated with a fluorescein isothiocyanate [FITC]-labeled secondary antibody (3.0 μg/mL; Serotec, Düsseldorf, Germany) before selection with magnetic beads (human FITC selection kit; Stem Cell Technologies, Vancouver, BC, Canada).
Terminal neural differentiation
Induction of terminal neural differentiation was initiated by culturing EGF/FGF-2-pretreated cells (1 × 10 4 cells/cm 2 ) on collagen-I–coated dishes (Biocoat, BD Biosciences) in neurobasal medium (including 10% FBS, 1 μM all-trans-retinoic acid [RA; Sigma], 15 ng/mL human brain-derived neurotrophic factor [hBDNF, Invitrogen]) for 1 week.
Cells selected for SSEA-4 (method C) were expanded on collagen-coated dishes (3 × 10 2 cells/cm 2 , neurobasal medium with 10 ng/mL hEGF, 10 ng/mL hFGF-2, 1 μM RA, 15 ng/mL hBDNF) for 1 week followed by 1 week of withdrawal of hEGF and hFGF-2.
Immunocytochemistry of neural differentiation markers
Cells were fixed in 4% paraformaldehyde (weight per volume in PBS), and immunocytochemistry was carried out as described. Cells were counterstained with 4,6-diamidino-2-phenylindole (Sigma-Aldrich). Primary antibodies and dilutions used are listed in Table 1 . Indocarbocyanine (Cy3)-, FITC- or phycoerythrin (PE)-labeled secondary antibodies were then applied (1:200, from Sigma-Aldrich; GE Healthcare, Piscataway, NJ; and Abcam, Cambridge, MA).
Antigen | Host | Manufacturer | Dilution |
---|---|---|---|
Nestin | Mouse | Acris | 1:100 |
β-Tubulin III (Tuj-1) | Mouse | Neuromics | 1:200 |
TUC-4 (TOAD) | Rabbit | Chemicon | 1:500 |
NeuN | Mouse | Chemicon | 1:100 |
Microtubule associated proteins (MAP1B/MAP5) | Mouse | Kamiya | 1:100 |
MAP2 (2a/2b) | Mouse | Acris | 1:500 |
Neurofilament light chain (NF-L, 70 kDa) | Mouse | Abcam | 1:100 |
Neurofilament medium chain (NF-M; 145 kDa) | Chicken | Novus Biochemicals | 1:200 |
Taurine transporter 1 (TAU1) | Rabbit | Alpha Diagnostics | 1:100 |
Tyrosine hydroxylase (TH) | Mouse | Sigma-Aldrich | 1:500 |
GABA | Rabbit | Sigma-Aldrich | 1:5000 |
Glutamic acid decarboxylase (GAD 65) | Rabbit | Chemicon | 1:500 |
Choline acetyltransferase (ChAT) | Mouse | Chemicon | 1:100 |
Tryptophan hydroxylase (TPH) | Mouse | Sigma-Aldrich | 1:100 |
Dopamin-beta-hydroxylase (DBH) | Rabbit | Chemicon | 1:250 |
O1 | Mouse | Chemicon | 1:100 |
O4 | Mouse | Chemicon | 1:100 |
Chondroitin sulfate proteoglycan (NG2) | Mouse | Chemicon | 1:150 |
Myelin basic protein (MBP) | Rabbit | Chemicon | 1:200 |
Galactocerebroside (GalC) | Rabbit | Acris | 1:100 |
Glial fibrillary acidic protein (GFAP) | Mouse | Chemicon | 1:1000 |
Proteomics: 2-dimensional difference gel electrophoresis (DIGE) assay
The cells were lysed in DIGE labeling buffer (30 mM Tris, pH 8.5, 7 M urea, 2 M thiourea, 4% [weight/volume] ([3-cholamidopropyl] dimethyl-ammonio)-1-propane-sulfonate [CHAPS], 1 μg total protein per microliter). Ten microliters of the lysate were labeled with 0.4nmol Cy2, Cy3 or Cy5 (GE Healthcare) dyes by incubation on ice for 30 min in the dark. The reactions were quenched with 1 μL of 10 mM lysine for 10 min on ice.
The labeled protein extracts were cup-loaded on 24 cm immobilized pH gradient (IPG) strips (pH 3-10; General Electric) and rehydrated (7 M urea, 2 M thiourea, 4% [weight/volume] CHAPS, 0.5% [volume/volume] Pharmalyte 3-10 [GE Healthcare], complete protease inhibitors [Roche, Basel, Switzerland], 1% [weight/volume] dithiothreitol [DTT]).
Isoelectric focusing was carried out for 18 hours on an IPGphor apparatus (GE Healthcare) with 45,000 Vh. The IPG strips were reduced in equilibration buffer (10 mM Tris [pH 8.8], 6 M urea, 30% [volume/volume] glycerol, 2% [weight/volume] sodium dodecyl sulfate [SDS], 2% [weight/volume] DTT) for 10 minutes and alkylated in equilibration buffer containing 5% [weight/volume] iodoacetamide for another 10 minutes.
For the second dimension, the strips were sealed with 0.5% agarose in SDS running buffer on top of 10-14% acrylamide gradient gels. The gels were run in Ettan Dalt II tanks (GE Healthcare) at 25°C. Gels were scanned (Typhoon scanner; GE Healthcare) and the images analyzed using Progenesis SameSpots 2.0.2733 (Nonlinear Dynamics, Newcastle upon Tyne, UK). Regulated proteins were identified from separate preparative 2-dimensional (2D) gels loaded with 500 μg total protein and labeled using Colloidal Coomassie. Spots were picked (GelPal; Genetix, New Milton, Hampshire, UK) and digested overnight at 37°C (19 ng trypsin [Promega, Madison WI] in 47 mM Tris, pH 9.0).
The peptides were sequenced using liquid chromatography and tandem mass spectrometry (LCQ Deca XP; Thermo Scientific, Waltham, MA) and proteins were identified using Mascot (Matrix Science, Boston, MA). Significantly different protein expression between the differentiation states was identified by 1-way repeated-measures analysis of variance followed by pairwise multiple comparison (Holm-Sidak, P < .05).
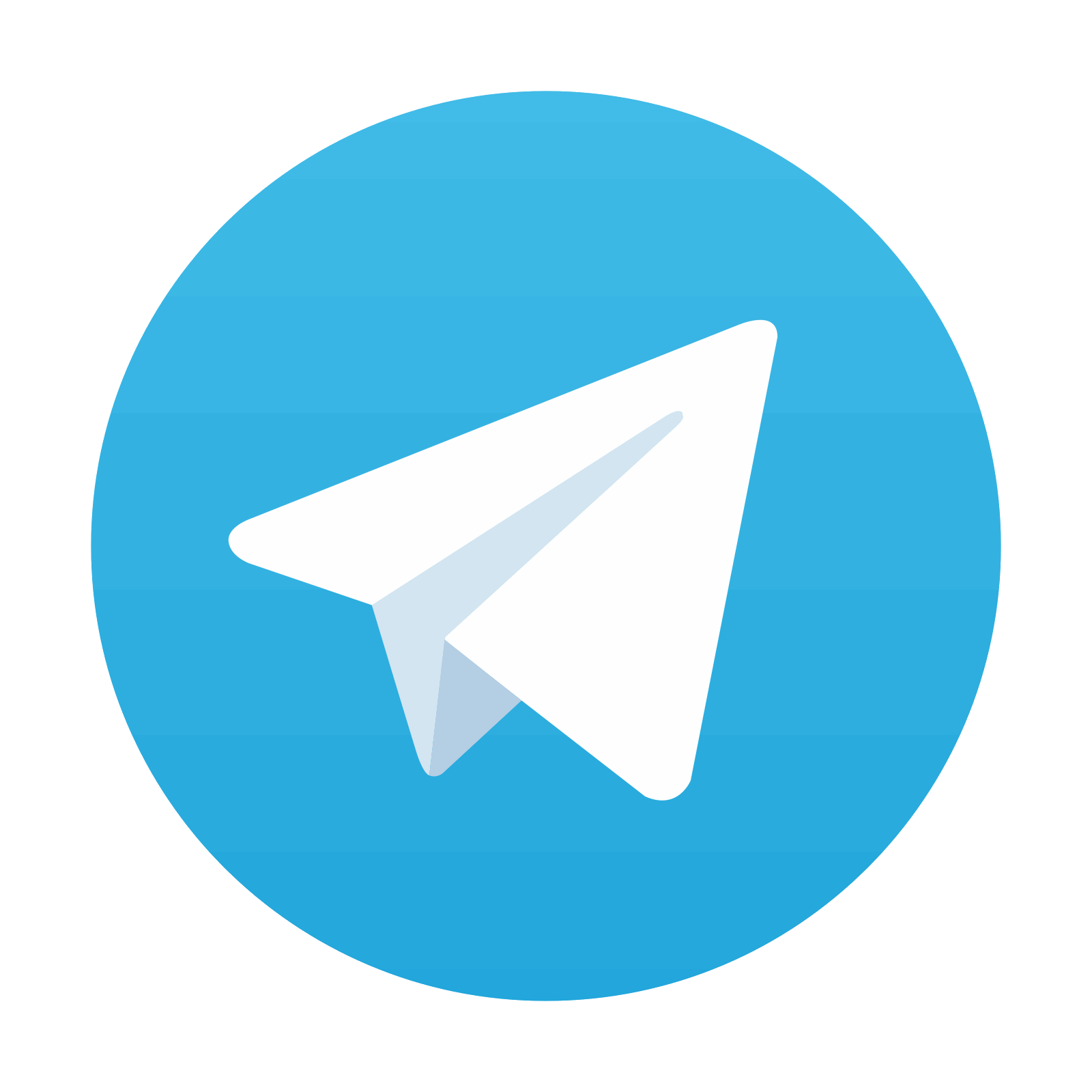
Stay updated, free articles. Join our Telegram channel

Full access? Get Clinical Tree
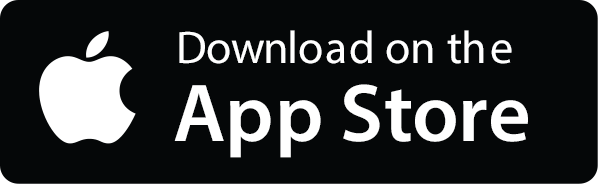
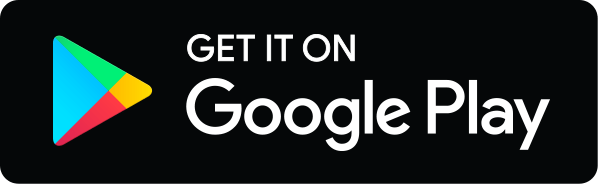