Tubular Dysfunction
This section deals with tubular disorders that present commonly in the neonatal period, including those for which early onset of treatment during the neonatal period may modify or delay the evolution toward renal failure. The reader is referred to other sources for discussion of uncommon disorders not included here (858).
Hypercalciuria, Nephrocalcinosis, and Nephrolithiasis
Normal values of the urinary calcium-to-creatinine ratio in full-term infants are less than 0.86 mg/mg (2.42 mMol/ mMol) between 5 days and 7 months (859). Nephrocalcinosis and nephrolithiasis are increasingly recognized in infants with hypercalciuria, most often in premature infants (see next section) (Table 42-19). Prenatal development of nephrocalcinosis has been detected in neonatal familial hyperparathyroidism (860). Hypercalciuria may result from increased calcium intake or ingestion, decreased tubular reabsorption (861) (see sections on Renal Tubular Acidosis and Hypokalemic Salt Losing Nephropathies), or increased bone calcium reabsorption or uptake. Nephrocalcinosis and nephrolithiasis also may result from the precipitation of calcium phosphate or oxalate in the absence of hypercalciuria (see Primary Oxaluria type I and Oxalosis). Finally, nephrolithiasis may result from high urinary concentrations of cystine (see Cystinuria), uric acid (see Uric Acid), xanthine (see Xanthinuria), 2,8-dihydroxyadenine (see Adenine Phosphoribosyltransferase Deficiency), or acyclovir (see Nephrotoxicity), and may occur in association with UTI (see Urinary Tract Infection) and with obstructive urinary tract malformations (844,845,846). Nephrocalcinosis may decrease renal tubular function, whereas
nephrolithiasis may cause hematuria, colic, dysuria, UTI, hydronephrosis, ARF, and CRF. The treatment of asymptomatic urolithiasis is high fluid intake and specific therapy when available; lithotripsy and surgical treatment are discussed in Chapter 43.
nephrolithiasis may cause hematuria, colic, dysuria, UTI, hydronephrosis, ARF, and CRF. The treatment of asymptomatic urolithiasis is high fluid intake and specific therapy when available; lithotripsy and surgical treatment are discussed in Chapter 43.
TABLE 42-19 MECHANISMS OF NEPHROCALCINOSIS AND NEPHROLITHIASIS IN INFANCY | ||
---|---|---|
|
Nephrocalcinosis and Nephrolithiasis in Premature Infants
Nephrocalcinosis or nephrolithiasis, detected by ultrasonography in 20% to 60% of premature infants with a GA less than 32 weeks, is predominantly as a result of hypercalciuria (862,863,864,865,866,867). Hypercalciuria may result from increased calcium intake or gastrointestinal absorption, decreased renal tubular calcium reabsorption, and abnormal regulation of bone mineral content (Table 42-19) (868). Chronic use of discontinuous daily calcium infusions is associated with recurrent periods of hypercalcemia and hypercalciuria. High enteral calcium intake may be associated with hypercalciuria in the absence of hypercalcemia (869). Insufficient phosphate intake may result in hypophosphatemia, hypercalcemia, hypercalciuria, and osteopenia of prematurity (870). Increasing the phosphate intake in these patients reduces calcemia and calciuria (871). Several authors have found a significant association between administration of furosemide and hypercalciuria in LBW infants (863,865,872). Hypercalciuria also may be induced by spironolactone (873,874) or by thiazides in patients with increased sodium intake or extracellular volume (868,875,876). Addition of acetate to the TPN reduces calciuria without affecting serum levels of calcium, PTH, or vitamin D (877). Acute and chronic acidosis induce bone calcium reabsorption and hypercalciuria.
Other factors may contribute to the development of nephrocalcinosis. One study using multivariate analysis showed that risk factors for nephrocalcinosis included duration of ventilation, toxic gentamicin or vancomycin levels, low fluid intake during week 3, and male gender (878). The risk for lithiasis and nephrocalcinosis increases with high saturation levels, which result from low urine output or high urinary oxalate or urate (879,880). To prevent hypercalciuria, VLBW infants should receive enough phosphate and should receive neither discontinuous calcium infusions nor excessive doses of vitamin D. If chronic diuretic therapy is indicated, the drug of choice is a thiazide (or metolazone if CRF), without sodium supplementation, and renal US should be done to assess for possible nephrocalcinosis or nephrolithiasis. Nephrocalcinosis appears to resolve spontaneously in 40% to 50% of cases on follow up, but can be associated with recurrent UTI, renal colic, and hematuria. Patients with persistent calcifications at 1 to 2 years of age show signs of tubular dysfunction, including a decrease in tubular reabsorption of phosphate, increase in FENa, and limitation of distal renal tubular acidification (881). Thus, hypercalciuric patients with nephrolithiasis or nephrocalcinosis should receive a thiazide without sodium supplementation; if an additional diuretic is required, amiloride or triamterene may be considered (882).
Primary Hyperoxaluria TYPE I and Oxalosis
Primary hyperoxaluria type I, i.e., glycolic aciduria is an autosomal recessive disorder as a result of a functional deficiency of the hepatic peroxisomal enzyme alanine: glyoxylate aminotransferase (AGT) (883), which leads to oxalosis, i.e., progressive accumulation of calcium oxalate in various tissues. The AGT gene has been cloned and mapped to chromosome 2q36-37 (884,845,886). The diagnosis is
strongly suggested by a high oxalate-to-creatinine ratio in the urine (887,888,889,890) in the absence of secondary causes for oxaluria (e.g., TPN or vitamin B6 deficiency), and is further supported by measuring glycolic aciduria and L-glyceric aciduria (889,891). Definitive diagnosis is possible by measuring AGT in a liver biopsy or by DNA analysis. Approximately 12% of patients present in infancy, with anorexia, failure to thrive, vomiting, dehydration, and fever; presentation in the neonatal period is rare (892,893,894). Renal damage includes nephrocalcinosis, urolithiasis, and renal failure. In pyridoxine-responsive patients, therapy includes pyridoxine, inhibitors of calcium oxalate precipitation, large fluid intake (2 L/m2/d), and later dialysis and renal transplantation (895,896,897). In pyridoxine-resistant patients, the treatment of choice is either early liver transplantation or combined hepatorenal transplantation for end stage renal disease (898).
strongly suggested by a high oxalate-to-creatinine ratio in the urine (887,888,889,890) in the absence of secondary causes for oxaluria (e.g., TPN or vitamin B6 deficiency), and is further supported by measuring glycolic aciduria and L-glyceric aciduria (889,891). Definitive diagnosis is possible by measuring AGT in a liver biopsy or by DNA analysis. Approximately 12% of patients present in infancy, with anorexia, failure to thrive, vomiting, dehydration, and fever; presentation in the neonatal period is rare (892,893,894). Renal damage includes nephrocalcinosis, urolithiasis, and renal failure. In pyridoxine-responsive patients, therapy includes pyridoxine, inhibitors of calcium oxalate precipitation, large fluid intake (2 L/m2/d), and later dialysis and renal transplantation (895,896,897). In pyridoxine-resistant patients, the treatment of choice is either early liver transplantation or combined hepatorenal transplantation for end stage renal disease (898).
Disorders of Purine Metabolism
Two disorders of purine metabolism may lead to nephrolithiasis in infancy: classic xanthinuria and adenine phosphoribosyltransferase deficiency.
Classic Xanthinuria
Classic xanthinuria is an autosomal recessive disorder with two genotypes. Type I is an isolated defect of the xanthine dehydrogenase (XDH) gene, which has been mapped to chromosome 2p22-23 (899,900). Classic xanthinuria type II is a combined defect of XDH and aldehyde oxidase (900a), which results from a functional defect of the human molybdenum cofactor sulfurase gene (HMCS) gene (901). Both disorders cause severe neonatal encephalopathy, lens dislocation, and microcephaly. Additionally, xanthinuria may result from the administration of allopurinol (inhibitor of xanthine oxidase) to a patient with high uric acid production, i.e., Lesch-Nyhan syndrome or treatment of malignancy. Classic xanthinuria may present with complications of xanthine urolithiasis or with ARF; some patients eventually develop CRF, duodenal ulcers, myopathy, or arthropathy, whereas others remain asymptomatic. The association of ARF with a history of hematuria or red-brown deposits on the diaper or in the urine sediment should raise the suspicion of classic xanthinuria (902). US may show the lithiasis. Classic xanthinuria is diagnosed by demonstrating high levels of xanthine and hypoxanthine and low-to-undetectable levels of uric acid in plasma and urine. Early treatment of xanthinuria with high fluid intake, a diet low in purines and adequate therapy of nephrolithiasis and its complications yields an excellent prognosis.
Adenine Phosphoribosyltransferase Deficiency
Adenine phosphoribosyltransferase deficiency is an autosomal recessive disorder as a result of mutations of the adenine phosphoribosyltransferase deficiency gene, which has been cloned and mapped to chromosome 16q24 (903). Two types have been characterized: patients with type I (mainly Caucasians) have no enzyme activity in their erythrocyte lysates, whereas those with type II (Japanese) have some residual activity. DNA analysis shows that these two types correspond to different mutations (904). The defect causes an increase in urinary excretion of 2,8-dihydroxyadenine, which is very poorly soluble. The disease presents with the complications of lithiasis, which can develop even in the neonatal period. The diagnosis can be suspected by visualizing round brown crystals in the urine sediment and confirmed by measuring erythrocyte adenine phosphoribosyltransferase deficiency activity and by DNA analysis. The treatment of this disorder includes a high fluid intake, allopurinol, alkali, and a diet low in purines. The prognosis depends on renal function at the time of the diagnosis; patients with ESRD require renal transplantation in addition to disease-specific therapy.
Familial Hypocalciuric Hypercalcemia
Familial hypocalciuric hypercalcemia (FHH) may correspond to three different genotypes. Type I, the most common, is an autosomal dominant disorder resulting from a defect in the human calcium sensor receptor (CaSR) gene, which has been cloned and mapped to chromosome 3q21-q24 (905,906,907). Type II has been mapped to chromosome 19p13.3, and type III to chromosome 19q13 (908,909,910). Inactivating mutations of the CaSR gene increase both the parathyroid calcium set point, i.e., serum calcium concentration that results in a 50% reduction in maximum PTH release and the set point for renal tubular reabsorption of calcium. Analysis of several families has shown that FHH is the heterozygous, benign form, whereas neonatal severe hyperparathyroidism is the life-threatening homozygous form (911,912). In contrast, activating mutations of the CaSR gene result in autosomal dominant hypocalcemia, which is associated with urolithiasis (see Table 42-19) (861).
The diagnosis of FHH is based on family history of hypercalcemia, a low urine calcium-to-creatinine ratio (less than 0.03 mg/mg), a low fractional excretion of calcium (less than 0.016, or 1.6%), and high magnesium and low phosphate concentrations in the serum (913,914,915). Hypercalcemia and hypermagnesemia result from an increase in their tubular reabsorption and in their release from bone, whereas hypophosphatemia results from a decrease in renal tubular reabsorption. In most patients with FHH, PTH levels are within normal limits for normocalcemic controls but inappropriately high for the serum Ca2+ concentration. The differential diagnosis includes other causes of neonatal hypercalcemia (see section on Nephrocalcinosis and Nephrolithiasis and Chap. 36), and multiple endocrine neoplasia syndromes (see Chapter 39). FHH is usually a benign disorder that fails to respond to parathyroidectomy (916). However, some patients with FHH have recurrent pancreatitis. Some neonates with FHH have transient self-limited hyperparathyroidism (917). In contrast, those with neonatal severe hyperparathyroidism
have severe hypercalcemia, high serum alkaline phosphatase activity, and typical radiographic bone changes; these patients may require total parathyroidectomy followed by administration of 1,25(OH)2D3 (918,919).
have severe hypercalcemia, high serum alkaline phosphatase activity, and typical radiographic bone changes; these patients may require total parathyroidectomy followed by administration of 1,25(OH)2D3 (918,919).
Fanconi Syndrome
Fanconi syndrome is characterized by generalized dysfunction of the proximal tubule. The cardinal signs are renal glucosuria, renal phosphaturia, and generalized aminoaciduria; other features, present inconsistently, include proximal RTA (pRTA), tubular proteinuria, increased urinary excretion of urate, sodium, potassium, and calcium, and decreased ability to concentrate the urine and to secrete PAH (919a). In some cases, distal tubular dysfunction is present, and the disease evolves toward renal failure, with less evidence of tubular dysfunction.
Because several transport systems are deficient in this syndrome, the pathophysiologic process presumably involves a global disturbance, such as an alteration of the integrity of the tubular membranes or of sulfhydryl-requiring enzymes (919a).
Idiopathic cases of Fanconi syndrome most often are sporadic, although autosomal recessive, autosomal dominant, and X-linked recessive transmission have been described (920). Fanconi syndrome occurs in association with a variety of disorders (Table 42-20), the most common of which is cystinosis (921). Disorders associated with late-onset renal dysfunction will not be reviewed here. General discussion of inborn errors of the metabolism can be found in Chapter 41.
The clinical presentation of Fanconi syndrome includes polyuria, polydipsia, dehydration, and failure to thrive. Signs include acidosis, hypophosphatemia, and rickets. The diagnosis is confirmed by the demonstration of glucosuria in the presence of a normal glycemia (less than 120 to 150 mg/dL), decreased tubular reabsorption of phosphate, and generalized hyperaminoaciduria. The prognosis of Fanconi syndrome depends on the underlying disorder.
TABLE 42-20 CAUSES OF FANCONI SYNDROME IN INFANCY | ||||||||||||||||||||||||||||
---|---|---|---|---|---|---|---|---|---|---|---|---|---|---|---|---|---|---|---|---|---|---|---|---|---|---|---|---|
|
The treatment includes administration of sodium citrate or bicarbonate, and as required, water, potassium, phosphate, and carnitine (922). Once rickets has developed, careful administration of vitamin D is required; excess vitamin D may result in hypercalciuria and nephrocalcinosis. Indomethacin, a PGSI, has been given successfully to some patients (923,924). Specific dietary therapy for fructose intolerance, galactosemia, or tyrosinemia results in disappearance of the Fanconi syndrome. In many other diseases, treatment serves only to slow the deterioration of renal function.
Special Considerations
Cystinosis
The reported incidence of cystinosis ranges between 1:20,000 and 1:326,000 (858). It is an autosomal recessive lysosomal transport disorder, characterized by accumulation of cystine, as a result of a defect in the carrier-mediated transport of cystine from the lysosomes to the cytosol (925). Cystinosis results from inactivating mutation of the CTNS gene, mapped to the short arm of chromosome 17p13 (926), which encodes cystinosin, a lysosomal cystine transporter. The infantile, i.e., nephropathic type of cystinosis is the most severe form (858,927) and is characterized by fluid and electrolyte loss, aminoaciduria, glycosuria, phosphaturia, RTA, rickets, and growth restriction, with onset of symptoms generally between 6 and 12 months of age. At birth, patients with cystinosis appear normal except for lighter skin and hair pigmentation than siblings. In some patients, the initial presentation may suggest a diagnosis of Bartter syndrome or of nephrogenic diabetes
insipidus (NDI) (927). Retinopathy may be detected within the first weeks of life (928), whereas characteristic corneal opacities appear only after 1 year. Laboratory findings include urinary excretion of typical cystine crystals, besides features of Fanconi syndrome. Progressive deterioration of the GFR leads to ESRD at a median age of 9.2 years (929).
insipidus (NDI) (927). Retinopathy may be detected within the first weeks of life (928), whereas characteristic corneal opacities appear only after 1 year. Laboratory findings include urinary excretion of typical cystine crystals, besides features of Fanconi syndrome. Progressive deterioration of the GFR leads to ESRD at a median age of 9.2 years (929).
PCR detection assays have been established, providing basis for rapid molecular diagnosis of cystinosis (930). Prenatal diagnosis is made by direct measurement of cystine in cultured amniocytes or chorionic villi cells. The mutational spectrum varies with different populations (931). Genetic counseling could be offered to families of specific ethnic backgrounds by screening of the most common mutation, e.g., G339R in Jewish-Moroccan and Amish-Mennonite populations (931).
The treatment of cystinosis in infancy includes alkalinization and supplementation of water, potassium, carnitine (922), phosphate, and vitamin D. Indomethacin, a PGSI, may reduce urinary losses and improve growth; however, it also may transiently decrease GFR (923,924). The treatment of choice is the administration of cysteamine, i.e., β-mercaptoethylamine hydrochloride (which is poorly tolerated and has persistent nauseating odor) or phosphocysteamine (which lacks the foul taste and odor of cysteamine). These agents help deplete cells of cystine (932). Treatment improves growth and delays the progression toward renal failure (933,934), especially if initiated soon after birth (935).
Arthrogryposis, Renal Tubular Dysfunction, and Cholestasis Syndrome
This is an autosomal recessive syndrome seen predominantly in Pakistanis. Renal tubular dysfunction ranges from isolated RTA to complete Fanconi syndrome, and hepatic histology shows various combinations of cholestasis, intrahepatic biliary hypoplasia, giant cell hepatitis, lipofuscin deposition, and fibrosis. Additional features observed in some patients include failure to thrive, NDI, neurogenic muscular atrophy (resulting in arthrogryposis), cerebral malformations, ichthyosis, dysmorphism, nerve deafness, and abnormality in platelet morphology (936). At least 2 candidate genes (FIC1 and HNF1) have been identified (937).
Glycogen Storage Disease with Fanconi Syndrome (Fanconi-Bickel Syndrome)
More than 112 patients have been described with Fanconi- Bickel syndrome, i.e., glycogen storage disease [GSD] with Fanconi syndrome. This syndrome is caused by homozygous or compound heterozygous mutations within GLUT2, the gene encoding the most important facilitative glucose transporter in hepatocytes, pancreatic β cells, enterocytes, and renal tubular cells. It is characterized by the association of hepatomegaly secondary to glycogen accumulation, glucose and galactose intolerance, fasting hypoglycemia, Fanconi syndrome, and severely stunted growth (938). In the kidney, glycogen accumulation is limited to the proximal tubule, with maximal levels in the straight part. This syndrome should be differentiated from phosphorylase b kinase deficiency (939) or from GSD type I (often as a result of glucose-6-phosphatase deficiency), which is associated with late onset of proximal tubular dysfunction in approximately 15% of the cases (940).
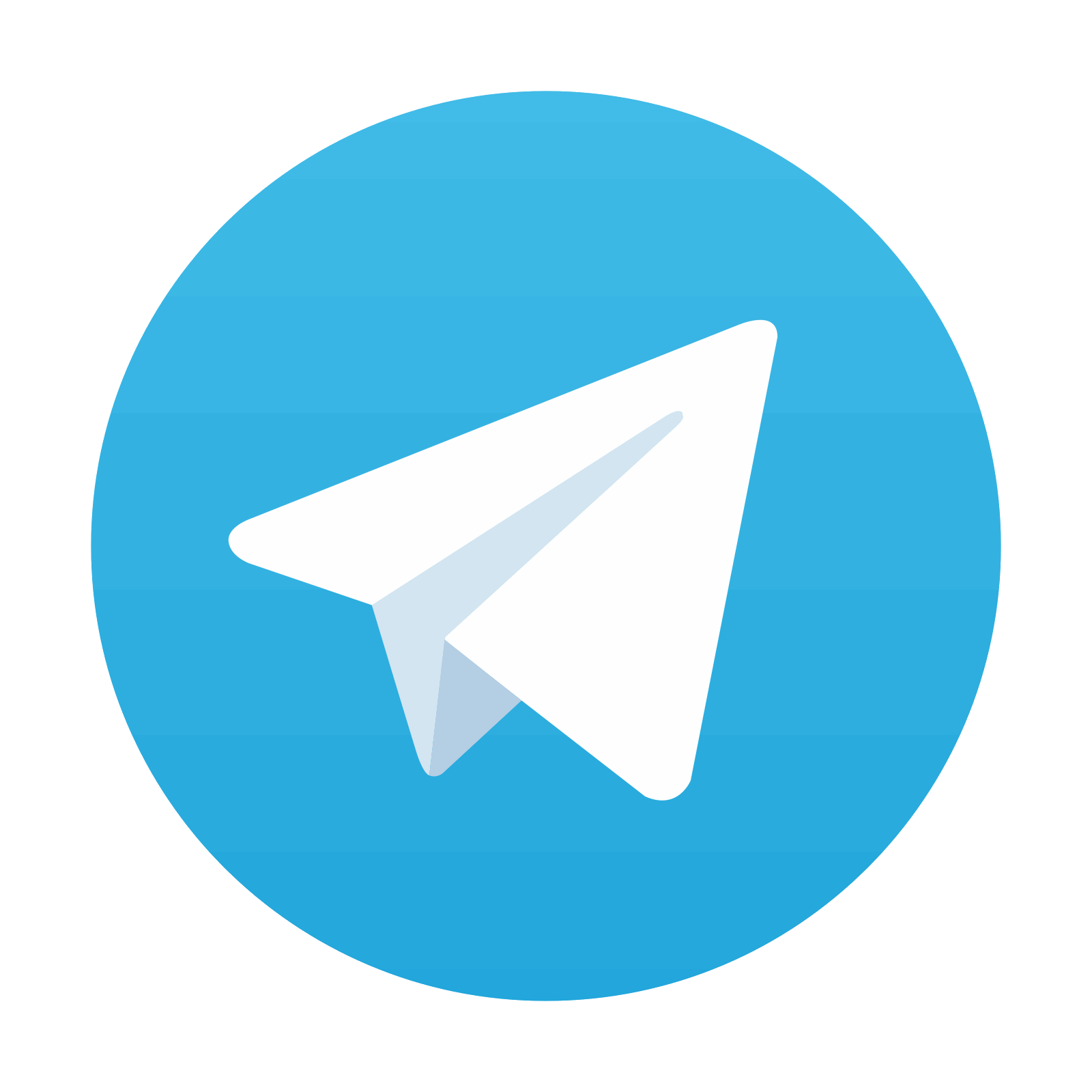
Stay updated, free articles. Join our Telegram channel

Full access? Get Clinical Tree
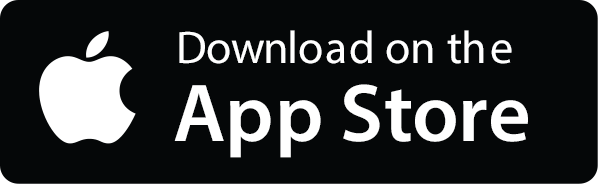
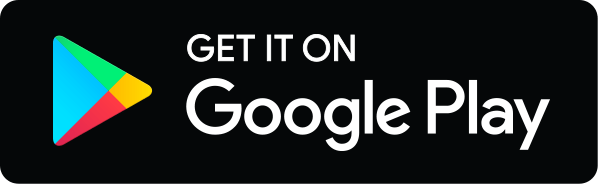