Objective
The objective of the study was to determine the bidirectional transfer of pravastatin across the dually perfused term human placental lobule and its distribution between the tissue and maternal and fetal circuits.
Study Design
The transfer of pravastatin was determined in the maternal-to-fetal (n = 11) and fetal-to-maternal (n = 10) directions. Pravastatin was coperfused with its [ 3 H]-isotope and the marker compound antipyrine (20 μg/mL) and its [ 14 C]-isotope. The concentration of pravastatin in the perfused tissue and the maternal and fetal circuits was determined using liquid scintillation spectrometry. Inside-out vesicles prepared from placental brush border membranes were utilized to investigate the role of efflux transporters in the transplacental transfer of pravastatin.
Results
Pravastatin was transferred from the maternal to the fetal circuit and vice versa. In the maternal-to-fetal direction, the distribution of pravastatin at the end of experiment was as follows: 14 ± 5% of the drug was retained by the tissue, 68 ± 5% remained in the maternal circuit, and 18 ± 4% was transferred to the fetal circuit. The normalized transfer of pravastatin (clearance index) to antipyrine in the fetal-to-maternal direction (0.48 ± 0.07) was higher than its transfer in the maternal-to-fetal direction (0.36 ± 0.07, P < .01). Furthermore, pravastatin inhibited the adenosine triphosphate (ATP)–dependent uptake of the paclitaxel and estrone sulfate.
Conclusion
The transfer of pravastatin across the dually perfused placental lobule suggests that fetal exposure to pravastatin is plausible. The higher transfer of pravastatin in the fetal-to-maternal direction than the reverse as well as its inhibition of the ATP-dependent uptake of [ 3 H]-paclitaxel and [ 3 H]-estrone sulfate strongly suggest the involvement of efflux transporters in decreasing its transfer across the placenta and support pravastatin’s favorable pharmacokinetic profile in pregnancy.
Preeclampsia complicates up to 5% of pregnancies and is a major cause of maternal and neonatal morbidity and mortality. It also shares pathogenic similarities and many risk factors with adult cardiovascular diseases. To date, prevention of preeclampsia using various supplements and classes of medications had limited success. On the other hand, prevention of cardiovascular mortality and other cardiovascular events in nonpregnant patients using 3-hydroxy-3-methyl-glutaryl-coenzyme A (HMG-CoA) reductase inhibitors (statins) has been widely accepted.
The beneficial effects of the cholesterol-lowering drug pravastatin in reversing several pathophysiological pathways in cardiovascular disease which are also associated with preeclampsia such as angiogenic imbalance, endothelial injury, inflammation, and oxidative stress, provided biological plausibility for its use in the prevention of preeclampsia.
The use of pravastatin is contraindicated in pregnant patients (pregnancy category X) and current recommendations suggest discontinuing the medication before conception or immediately if the patient becomes pregnant. Pravastatin is one of the HMG-CoA reducatese inhibitors; thus, it decreases the synthesis of cholesterol which is important for normal fetal growth and development.
To date, data obtained from experimental animals and limited data from humans suggest that pravastatin does not increase the risk of major congenital anomalies. However, the use of other HMG-CoA reductase inhibitors (simvastatin, lovastatin, atorvastatin, cerivastatin) may be associated with an increase in the incidence of skeletal malformations and was attributed to the difference in the physicochemical properties between the hydrophilic pravastatin vs the other lipophilic HMG-CoA reductase inhibitors.
The molecular weight of pravastatin is 446.5 and its protein binding is between 43% and 54%. The low permeability of pravastatin across biological membranes including the human placenta is to be expected because of its hydrophilic properties. On the other hand, it appears that both uptake and efflux transporters contribute to pravastatin distribution across biological membranes. Thus, it was demonstrated that the permeability of pravastatin through intestinal membranes was higher than that expected from its hydrophilicity, suggesting the involvement of uptake transporters. Furthermore, the renal clearance of pravastatin exceeds the glomerular filtration rate, suggesting that renal tubular secretion contributes to the elimination of this compound.
Because intestinal and kidney transporters are important components of absorption and tubular secretion, the interaction of pravastatin with transporters in other tissues, including the placenta is possible. The placenta contains multiple drug transporters, some of which are efflux transporters that appear to be specifically dedicated to the removal of exogenous and endogenous toxic compounds from the fetal back to the maternal circulation and thus protect the developing fetus. They include the adenosine triphosphate (ATP)-biding cassette transporters such as P-glycoprotein (P-gp), several multidrug-resistant associated proteins (MRPs), and breast cancer–resistant protein (BCRP). They are usually located on the apical brush border membrane of the syncytiotrophoblasts.
Therefore, the aim of this investigation was to determine the bidirectional transfer of pravastatin across term human placenta, the involvement of efflux transporters, and distribution of pravastatin between placental tissue and maternal and fetal circuits. The aims were achieved by using the ex vivo technique of dual perfusion of placental lobule and in vitro preparations of inside-out vesicles from placental apical membranes.
Materials and Methods
Chemicals
[ 3 H]-pravastatin (specific activity, 5 Ci/mmol), [ 3 H]-paclitaxel (specific activity, 45.1 Ci/mmol), and [ 3 H]-estrone sulfate (specific activity, 45.6 Ci/mmol) were purchased from American Radiolabeled Chemicals (St. Louis, MO), Moravek Biochemicals, Inc (Brea, CA), and PerkinElmer (Boston, MA), respectively.
The nonradioactive pravastatin and all other chemicals including radioactive [ 14 C]-antipyrine (specific activity, 6.5 mCi/mmol) were purchased from Sigma-Aldrich (St. Louis, MO).
Clinical material
Placentas from uncomplicated term (37-42 weeks) singleton pregnancies (n = 21) were obtained immediately after vaginal or abdominal deliveries from the Labor and Delivery Ward of the John Sealy Hospital, the teaching hospital of the University of Texas Medical Branch (Galveston, TX) according to a protocol approved by the institutional review board. Exclusion criteria included maternal infections, systemic diseases, multifetal pregnancies, and drug or alcohol abuse during pregnancy.
Transplacental transfer and distribution of pravastatin
To determine the bidirectional transfer of pravastatin from the maternal-to-fetal and fetal-to-maternal circulations and the distribution of pravastatin in each compartment, we used the technique of dual perfusion of placental lobule as previously described by Miller et al and earlier reports from our laboratory.
Following the control period, the maternal and fetal perfusates were replaced with fresh medium. Human serum albumin, at a concentration of 3 mg/mL, was added to the media of both the maternal and fetal circuits. The nonionizable, lipophilic marker compound antipyrine 20 μg/mL and its [ 14 C]-isotope (1.5 μCi) were cotransfused with pravastatin to account for interplacental variations and to normalize the transfer of pravastatin to that of antipyrine.
Antipyrine and pravastatin were added either to the maternal or fetal reservoirs according to the transfer direction investigated (ie, from the maternal to fetal [M→F] or fetal to maternal [F→M], respectively). The initial concentration of pravastatin in the donor circuit was 50 ng/mL. This concentration corresponds to the reported serum levels of pravastatin following the administration of 40 mg dose daily.
The perfusion system was utilized in its closed-closed configuration (recirculation of the media). The concentrations of compounds were determined in 0.5 mL aliquots taken from the maternal and fetal arteries and veins at 0, 5, 10, 15, 30, 40, 50, 60, 90, 120, 150, 180, 210, and 240 minutes. The amount of radioactivity in both the maternal and fetal perfusate were determined by liquid scintillation spectrometry in the [ 3 H]- and [ 14 C]-channels simultaneously (1900TR; Packard Instruments, Inc, Shelton, CT).
At the end of each experiment, the perfused area was dissected from the adjoining placental tissue, weighed, and homogenized in a volume of saline equal to 4 times its weight. One milliliter of 1 M NaOH was added to 1 mL of the homogenate, and the samples were incubated for 12 hours at 60°C in the dark to allow for luminescence decay. A scintillation cocktail was added to each sample, counted, and the concentration of pravastatin calculated.
Effect of pravastatin on the uptake of [ 3 H]-paclitaxel and [ 3 H]-estrone sulfate by the placental vesicles
To assess the involvement of efflux transporters and the functional kinetics of transport mediated by transporters such as P-gp, MRP, and BCRP; we evaluated the effect of pravastatin on the ATP-dependent uptake of [ 3 H]-paclitaxel and [ 3 H]-estrone sulfate, which are known prototypic substrates of these efflux transporters. The method for preparing inside-out vesicles (IOVs) from placental brush border membranes is that originally described by Ushigome et al and modified in our laboratory as previously reported.
The effect of a range of pravastatin concentrations (0–100 μM) on the uptake of 40 nM [ 3 H]-paclitaxel and 100 nM [ 3 H]-estrone sulfate was determined in 140 μL of buffer (46 mM MOPs [3-(N-morpholino) propane sulfonic acid]-Tris, 65 mM KCl, 8 mM MgCl 2 , pH 7.4) using 40 or 80 μg of membrane protein, respectively. The reactions were initiated by the addition of either 4 mM ATP or 4 mM adenosine monophosphate (AMP) for control. The reactions were terminated after 1 or 3 minutes, respectively, by the addition of 3 mL ice-cold buffer followed by rapid filtration (Brandel cell harvester; Brandel, Gaithersburg, MD) to isolate the vesicles. The amount of [ 3 H]-substrates retained on the filter was determined by liquid scintillation analysis. Active uptake was calculated as the difference in the amount of [ 3 H]-substrate in the presence of ATP and AMP. The median inhibitory concentration (IC 50 ) was determined from plots of the percent of the [ 3 H]-substrate uptake vs the log of pravastatin concentration.
Data and statistical analysis
The M→F and F→M transfer rates (percentages) were calculated as follows: (C recipient /C donor ) × 100, where C recipient is the final concentration of drug in the recipient circuit and C donor is the initial concentrations of drug in the donor circuit. The clearance index (Cl index ) is the ratio between the transfer rate of the drug to that of the marker compound antipyrine.
Statistical analyses were performed using GraphPad Prism, version 5.01 (GraphPad Software Inc, La Jolla, CA). All reported values are expressed as mean ± SD. The statistical significance of the differences observed between groups were calculated by the Mann-Whitney U test and considered significant with a value of P < .05.
Results
Placental transfer of pravastatin in the maternal to fetal direction and its distribution
In this investigation, the transplacental transfer of antipyrine was approximately 50% and is consistent with previous reports from our laboratory. Pravastatin rapidly crossed the placenta and appeared in the fetal circuit within the initial 5 minutes of its perfusion. The concentration of pravastatin in the fetal circuit at the end of the 4 hour experimental period was 8.8 ± 1.8 ng/mL, and the fetal/maternal concentration ratio was 0.26 ± 0.06. The normalized transfer of pravastatin (Cl index ) to antipyrine in the M→F direction was 0.36 ± 0.07 ( Figure 1 ).

Full access? Get Clinical Tree
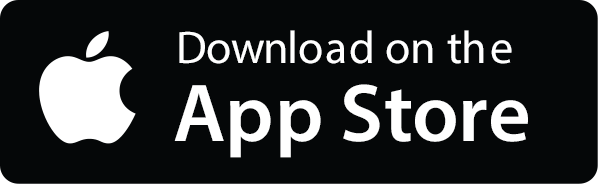
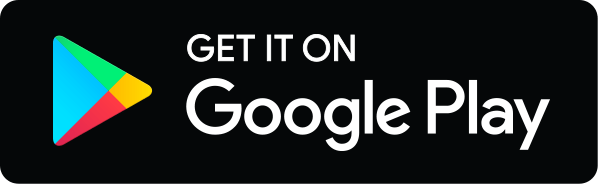