The household medicine cabinet is filled with products that are potentially toxic, many of which have been obtained over the counter. According to the 2011 American Association of Poison Control Centers’ National Poison Data System (NPDS) Annual Report, analgesics, vitamins, antihistamines as well as cough and cold preparations account for approximately 21% of all exposures in children younger than 6 years.1 Oral hypoglycemics, which are also ubiquitous, are another common household danger that cause significant morbidity and mortality in children.
Historically, iron preparations have been a leading cause of pediatric mortality from exploratory drug overdose. In 1997, the Food and Drug Administration (FDA) issued a regulation requiring warning labels and childproof packaging for iron-containing products with 30 mg or more of iron per dosage unit.2 Since the implementation of childproof packaging and warning labels, the trend in NPDS data suggests that iron poisoning and fatalities are decreasing.3,4 In 2004, the iron packaging regulations were repealed, thus ferrous sulfate tablets and prenatal vitamins remain a toxic threat.
Different formulations of iron contain disparate amounts of elemental iron (Table 166-1). Mild toxicity, expressed in terms of milligrams of elemental iron per kilogram of body weight, is seen with doses as low as 20 mg/kg elemental iron, whereas systemic illness generally occurs with doses in the range of 40 to 60 mg/kg. The pharmacokinetics of iron in an overdose remains somewhat of a mystery.5 Peak iron levels are thought to occur between 2 and 6 hours after ingestion. Serum iron levels generally correlate with clinical severity: mild symptomatology (vomiting, diarrhea) is seen with levels less than 300 μg/dL, moderate symptoms with levels of 300 to 500 μg/dL, and severe manifestations (shock, acidosis, seizures, coma) with levels exceeding 500 μg/dL.6
Iron toxicity is described in phases. Early toxicity is caused by local irritant effects, whereas later findings are thought to be due to mitochondrial damage, particularly in the liver (Table 166-2).
Phase | Time from Ingestion | Symptoms | Pathophysiology |
---|---|---|---|
1 | 0-6 hr | Emesis, diarrhea, abdominal pain | Direct local irritant effect on gastrointestinal mucosa |
Can progress to hypovolemic shock | |||
2 | 6-12 hr | Improvement of phase 1 symptoms | Iron entering cells |
3 | 12-24 hr | Gastrointestinal fluid losses lead to hypovolemic shock Wide anion gap acidosis Depressed myocardial function Increased pulmonary vascular resistance Liver failure with hypoglycemia and coagulopathy | Iron concentrates intracellularly in mitochondria and disrupts oxidative phosphorylation, thereby resulting in free radical formation and lipid peroxidation Free iron may exhibit a direct inhibitory effect on the formation of thrombin and thrombin’s effect on fibrinogen in vitro |
4 | 2-6 wk and beyond | Emesis | Emesis secondary to pyloric stricture, liver failure secondary to hepatic cirrhosis |
Liver failure |
The signs and symptoms of iron toxicity (nausea and vomiting, anion gap metabolic acidosis, and acute liver injury) are very common in other disorders, and represent a large nontoxicologic differential diagnosis. Other possible toxicologic etiologies that cause anion gap metabolic acidosis include toxic alcohols, salicylates, isoniazid, massive ibuprofen ingestions, and metformin toxicity. Acetaminophen toxicity causes acute liver injury in a similar pattern to iron. Amanita phalloides mushroom poisoning is another important toxicologic cause of acute liver injury.
An abdominal radiograph is indicated to look for the presence and location of iron tablets. Completely dissolved iron tablets/capsules/liquid preparations may not be radiopaque; consequently, a negative radiograph does not rule out the possibility of toxic iron ingestion.7 However, patients with negative abdominal radiographs may be discharged after a 6-hour period of observation if they remain asymptomatic.8 Baseline laboratory studies, including serum iron levels (if available), hepatic studies (serum transaminases, albumin, and coagulation studies), and electrolytes, including the serum bicarbonate concentration, should be obtained 4 to 6 hours after ingestion for any patient with symptoms of toxicity or a significant history of ingestion. Leukocytosis (>15,000/mm3) and hyperglycemia (>150 mg/dL) are sometimes seen after iron poisoning, but these laboratory findings are neither sensitive nor specific. Metabolic acidosis should be regarded with concern.
Whole-bowel irrigation with a polyethylene glycol balanced electrolyte solution may be considered as a means of gastrointestinal decontamination.9 Iron is not adsorbed by activated charcoal. Further therapeutic guidelines, including deferoxamine chelation, are presented in Table 166-3.
Symptom | Laboratory Finding | Treatment |
---|---|---|
Asymptomatic | Negative KUB | Observe for 6 hr—discharge home if no symptoms develop |
Asymptomatic | Radiopaque pills on KUB | WBI and repeat KUB |
Determine electrolytes and SI 4-6 hr after ingestion | ||
Start deferoxamine if peak SI >500 μg/dL or metabolic acidosis | ||
Symptomatic | Radiopaque pills on KUB | As above |
or | Start deferoxamine by continuous infusion at a rate of up to 15 mg/kg/hr | |
Peak SI >500 μg/dL | ||
or | ||
Patient is symptomatic and | ||
SI cannot readily be obtained | ||
Symptomatic | Anion gap acidosis | Aggressive fluid resuscitation Consider CVVH if refractory acidosis |
Hypotension, shock | Watch for cardiogenic pulmonary edema | |
Dopamine and/or norepinephrine may be needed for refractory hypotension | ||
Consider exchange transfusion | ||
Symptomatic | Hypoglycemia | Watch for hypoglycemia and correct as needed |
Acute liver failure | Coagulopathy | FFP, cryoprecipitate as needed |
Intravenous deferoxamine remains the mainstay of treatment after gut decontamination. Oral deferoxamine is not recommended. Deferoxamine may be administered intramuscularly, but the injections are painful and may cause local tissue inflammation. Once therapy with deferoxamine is instituted, clinical monitoring should include serial measurements of blood pressure, serum iron concentration (if available), hepatic transaminases, electrolytes, and the prothrombin time. Of note, serum iron levels may be falsely lowered by the presence of deferoxamine. Therapeutic end points for deferoxamine therapy include resolution of clinical symptoms and metabolic acidosis as well as narrowing of the anion gap. In the presence of deferoxamine, iron levels may not be accurate, but persistently elevated levels should raise concern. Recurrent symptomatology may warrant reinstitution of deferoxamine, which should be undertaken carefully and at a lower dose.
The most dreaded complications of therapy with deferoxamine are Yersinia enteroeolitica septicemia and mucormycosis. The mechanism of this complication is unclear, but deferoxamine may provide the iron siderophore complex growth factor needed by the bacteria to induce overgrowth.10,11 Pulmonary toxicity, which is manifested as tachypnea, hypoxemia, fever, eosinophilia, preceding urticaria, and pulmonary infiltrates, may be seen in patients receiving both prolonged (>24 hours) and high (>15 mg/kg/hr) doses of intravenous deferoxamine.12-14 Hypotension, too, appears to be a dose-related effect. Ocular and otic toxicity has also been reported.
Additional treatment modalities such as continuous veno-venous hemofiltration15 and exchange transfusion16 are under investigation for the severely iron-poisoned patient.
Patients in whom signs of mild poisoning (continued vomiting, diarrhea) develop should be admitted for inpatient management. Patients who demonstrate serious systemic signs of toxicity (shock, central nervous system depression) should be considered candidates for an intensive care setting. Discharge from inpatient medical care may occur when symptoms and laboratory abnormalities have resolved and no clinical deterioration is noted after cessation of deferoxamine therapy.
Over-the-counter antihistamines are ubiquitous and used for cold and allergy symptoms and as sleep aids. Most of the toxicity associated with an overdose of antihistamines is due to their anticholinergic effects. Two of the non-sedating antihistamines, terfenadine (Seldane) and astemizole (Hismanal), have been discontinued because of significant cardiac toxicity when combined with drugs that impair their metabolism through cytochrome 3A4, such as ketoconazole or erythromycin.17 Cardiotoxicity has not been reported with the use of fexofenadine (Allegra), cetirizine (Zyrtec), loratadine (Claritin), or azelastine (Astelin), all of which have limited anticholinergic side effects.18 A list of some common antihistamines is provided in Table 166-4.
Azelastine (Astelin) |
Bilastine (Bilaxten) |
Brompheniramine (Dimetane)* |
Cetirizine (Zyrtec) |
Chlorpheniramine (Chlor-Trimeton)* |
Cyclizine (Marezine)* |
Dimenhydrinate (Dramamine)* |
Diphenhydramine (Benadryl)* |
Doxylamine* |
Fexofenadine (Allegra) |
Hydroxyzine (Atarax, Vistaril)* |
Loratadine (Claritin) |
Meelizine (Antivert)* |
Promethazine (Phenergan)* |
Absorption of antihistamines from the gastrointestinal tract is rapid, with the peak drug effect usually seen in 1 hour. However, after large ingestions, symptoms may not occur for several hours and may last for days. Patients who have taken an overdose of antihistamines may present with the classic anticholinergic toxidrome (Chapter 165). In addition, seizures are common after large overdoses of antihistamines. Some antihistamines, especially diphenhydramine, can cause wide-QRS tachyarrhythmias from sodium channel blockade19 as well as QT interval prolongation, placing patients at risk for malignant arrhythmias such as torsades de pointes.20 Antihistamines are often combined with other drugs in commercial products, so concomitant toxicity from sympathomimetic agents, acetaminophen, or dextromethorphan (see Chapter 169) may be encountered.
Other toxicologic causes of an anticholinergic toxidrome as seen in antihistamine ingestion include poisoning from agents such as tricyclic antidepressants (i.e. amitriptyline, nortriptyline), atypical antipsychotic medications (i.e. quetiapine, olanzapine), skeletal muscle relaxants (i.e. cyclobenzaprine), antispasmodics (i.e. Donnatal, Lomotil) or ingestion of anticholinergic alkaloid plants (i.e. jimsonweed, deadly nightshade, Amanita muscaria mushrooms).
The toxic effects of antihistamines are due mostly to anticholinergic phenomena through inhibition of both central and peripheral muscarinic cholinergic receptors. The diagnostic course for patients with a suspected overdose of antihistamines depends somewhat on the symptomatology and dose ingested. The mean dose in symptomatic children was 17.3 mg/kg in a study of 184 cases; deaths have been reported with doses as low as 33 mg/kg.21 The delirium associated with anticholinergic poisoning may lead to evaluation for central nervous system infection. Acetaminophen levels are of particular importance because many cold products contain both antihistamines and acetaminophen. Diphenhydramine may result in a false-positive urine immunoassay for phencyclidine (PCP). Electrocardiographic evaluation, specifically checking for widening of the QRS, is warranted to look for signs of impending arrhythmia as well as the possibility of coingestants.
Activated charcoal may reduce the absorption of antihistamine drugs if administered soon after ingestion. Inpatient care should be directed at symptoms (Table 166-5). Benzodiazepines may help decrease agitation and tachycardia. Physostigmine should be considered for both diagnosis and symptom management.22 The benefits of physostigmine, which include restoration of gastrointestinal motility and improvement in mental status, must outweigh the potential risks (worsening of cardiac conduction delays). Physostigmine may be used safely when the patient has a narrow QRS complex on electrocardiography and no evidence of ingestion of other agents (e.g. class IA or IC antiarrhythmics, tricyclic antidepressants) that may cause intraventricular conduction delays. Intravenous access and cardiovascular monitoring must be established before the use of physostigmine. The initial dose of physostigmine, 0.02 mg/kg in children or 1 to 2 mg in adults, may be administered by slow intravenous infusion every 5 minutes until the anticholinergic symptoms are reversed. Additional physostigmine should not be administered if significant cholinergic symptoms develop, particularly bradycardia or excessive salivation. A dose of atropine, approximately half the dose of physostigmine, should be readily available. Redosing of physostigmine may be needed every 30 to 60 minutes. Patients considered at high risk for the development of seizures may be coadministered a prophylactic dose of benzodiazepine.
Symptom | Treatment | Comments |
---|---|---|
Agitation/delirium | Physostigmine IV over 5-min period (adults, 1-2 mg; pediatric, 0.02 mg/kg) | Electrocardiographic conduction delay (prolonged PR, wide QRS) is relative contraindication for physostigmine |
Benzodiazepines IV | ||
Seizures | Benzodiazepines IV | In the setting of tricyclic antidepressant overdose, use of physostigmine has been associated with seizures and intractable cardiac arrest |
Physostigmine IV over 5-min period (adults, 1-2 mg; pediatric, 0.02 mg/kg) | ||
Hyperthermia | Cool bath, fans, sedation | |
Ventricular arrhythmia | Sodium bicarbonate bolus (1-2 mEq/kg), then drip (in D5W) | Monitor electrocardiogram continuously; monitor serum electrolytes and arterial blood gases |
Lidocaine bolus (1 mg/kg), then drip as needed (20-50 μg/kg/min) | Goal serum pH, 7.45-7.55 | |
Continue 24 hr past end points of cessation of dysrhythmias, normalization of QRS complexes | ||
Other tachyarrhythmia | Supportive treatment if hemodynamically stable | See above |
May consider beta-blocker (propranolol) | ||
May consider physostigmine if refractory | ||
Torsades de pointes | Cardioversion | |
Magnesium sulfate IV (adults, 2-6 g; pediatric, 25-50 mg/kg) | ||
Overdrive pacing | ||
Rhabdomyolysis | Fluid resuscitation, urinary alkalinization, and maintenance of urine output at 1-2 mL/kg/hr |
Patients displaying persistent changes in mental status, abnormalities in vital signs, electrocardiographic changes, or seizures should be admitted to the hospital. The severity of symptomatology may warrant admission to an intensive care setting.
Discharge from inpatient medical care may occur when the patient has become asymptomatic and no further drug absorption is anticipated.
Acetaminophen, also known as paracetamol, is responsible for a large number of toxic ingestions in children each year.1 Hepatic injury is the most frequent cause of morbidity and mortality after an overdose of acetaminophen. Extensive clinical research and experience have allowed clinicians to better predict and prevent the development of hepatotoxicity.23,24
Most forms of acetaminophen are rapidly absorbed after ingestion. Extended-release acetaminophen preparations have similar pharmacokinetics as the regular-release formulations, with peak levels occurring less than 4 hours after ingestion.25 Recent FDA approval of intravenous acetaminophen presents a novel formulation of acetaminophen with potential for toxicity.26
Acetaminophen is metabolized almost exclusively in the liver; approximately 90% is glucuronidated or sulfated, 5% is excreted unchanged in urine, and the remaining 5% is oxidized by liver cytochrome enzymes, specifically CYP2E1, CYP1A2, and CYP3A4. Oxidation produces the reactive electrophile N-acetyl-p-benzoquinone imine (NAPQI). In conditions of therapeutic dosing, NAPQI is detoxified by glutathione, whereas in conditions of decreased glutathione stores, large doses of acetaminophen, or induction of cytochrome enzymes, NAPQI overwhelms the capacity of glutathione. Free NAPQI binds to hepatocytes and causes hepatocellular damage.
The risk for hepatocellular injury can be assessed by the dose ingested, the acetaminophen level, or both. In acute ingestion, patients who take more than 200 mg/kg (children) or 7.5 g (adults) are at risk for acetaminophen toxicity. Some patients who chronically exceed the recommended doses of acetaminophen appear to be particularly at risk for hepatotoxicity; such patients include those with preexisting liver disease, children with acute febrile illnesses, and patients who chronically ingest inducers of CYP2E1.27-29
Patients who have been exposed to toxic doses of acetaminophen may have a paucity of findings on physical examination. Acetaminophen toxicity is divided into four clinical stages, and the findings of each phase are presented in Table 166-6.30
Stage | Time after Ingestion | Clinical Findings |
---|---|---|
I | 0.5 to 24 hr | Anorexia, nausea, vomiting, malaise, pallor, diaphoresis |
II | 24 to 48 hr | Resolution of early symptoms; right upper quadrant abdominal pain and tenderness; oliguria, elevated hepatic transaminases, prothrombin time, bilirubin, |
III | 72 to 96 hr | Peak of liver function abnormalities |
Reappearance of anorexia, nausea, vomiting | ||
Onset of fulminant hepatic failure with metabolic acidosis, coagulopathy, and renal dysfunction | ||
Changes in mental status, encephalopathy | ||
IV | 4 days to 2 wk | Resolution of hepatic dysfunction or progression to oliguric renal failure and death |
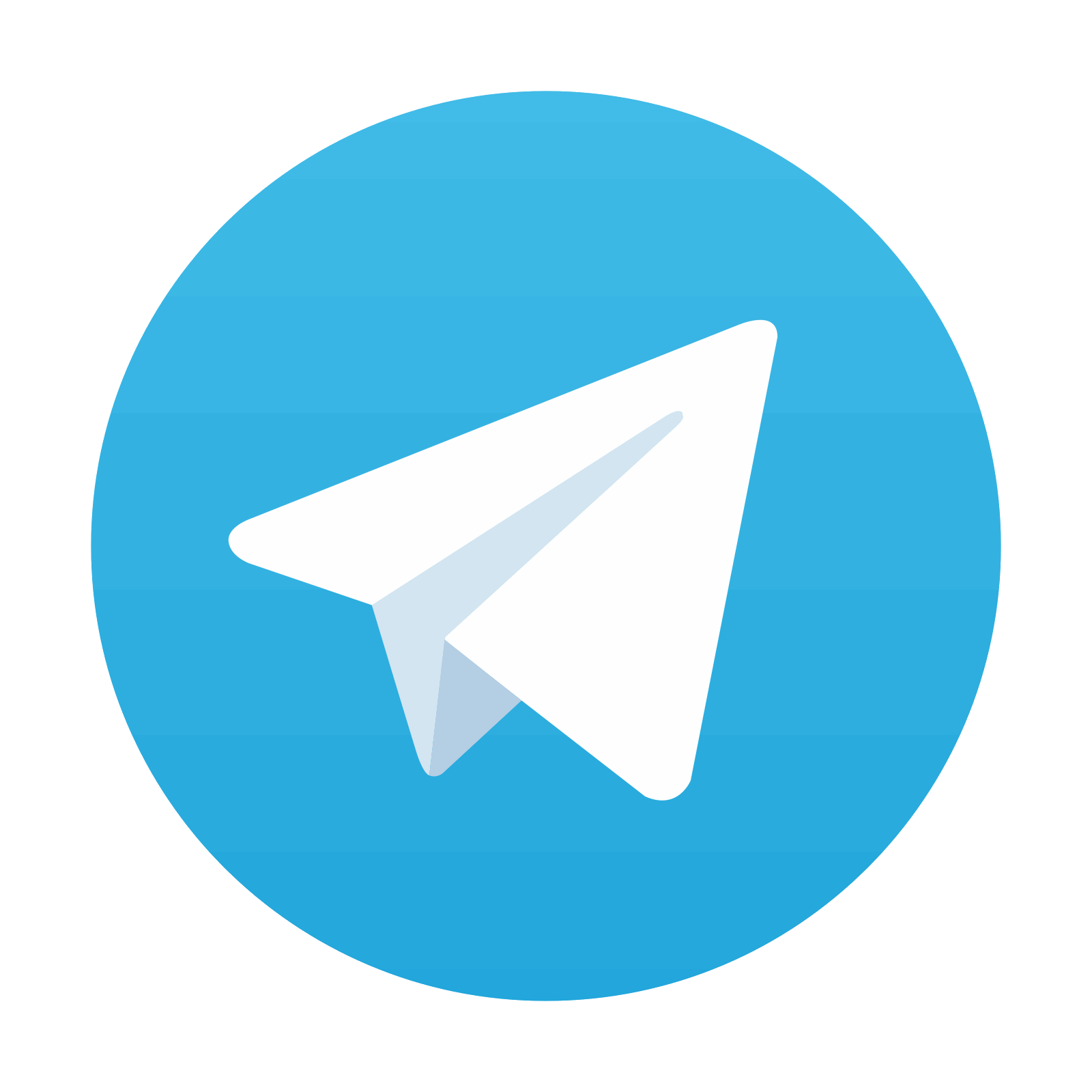
Stay updated, free articles. Join our Telegram channel

Full access? Get Clinical Tree
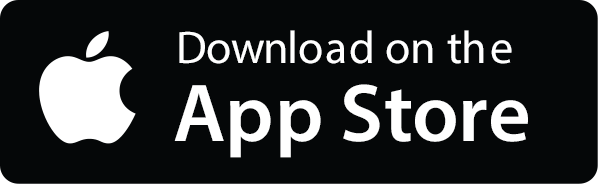
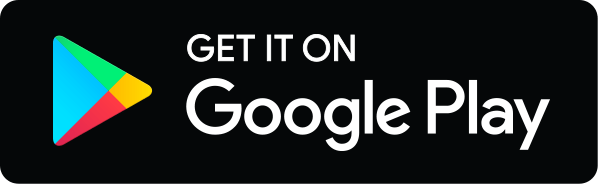
