In the clinical arena of transplantation, tolerance remains, for the most part, a concept rather than a reality. Although modern immunosuppression regimens have effectively handled acute rejection, nearly all organs except the liver commonly suffer chronic immunologic damage that impairs organ function, threatening patient and allograft survival. In addition to the imperfect control of the donor-directed immune response, there are additional costs. First, there is the burden of mortality from infection and malignancy that can be directly attributed to a crippled immune system. Second, there are insidious effects on renal function, cardiovascular profile (hypertension, hyperglycemia, and dyslipidemia), bone health, growth, psychological and neurocognitive development, and overall quality of life. It is likely that the full consequences of lifelong immunosuppression on our pediatric transplant recipients will not be fully appreciated until survival routinely extends beyond 1 or 2 decades after transplantation. Therefore, it can be argued that the holy grail of transplantation tolerance is of the utmost importance to children who undergo solid organ transplantation.
In 1956, John Murray performed the first successful kidney transplant. A kidney from one identical twin was transplanted into the other. Four years previously, the first attempt at pediatric kidney transplantation occurred in France. In this case, a 16-year-old boy received a kidney from his mother after a nephrectomy of his right kidney and subsequent discovery that his left kidney was missing. Although the mother was ABO compatible, the outcome, rejection after 21 days and death of the boy, demonstrated the powerful effects of the immune system’s allorecognition, a factor not at work in genetically identical transplants. At this point in history, the mechanisms underlying allorecognition were poorly understood, but 1 year later, in 1953, Peter Medawar performed the seminal experiment demonstrating the potential to overcome the alloimmune response and induce a state of immunologic tolerance. Although much progress has been made in controlling alloimmunity through the use of ever-improving immunosuppressive therapies, the discoveries of Medawar still have yet to be translated such that tolerance can be successfully achieved routinely in the clinical transplant setting.
Therefore, in the clinical arena of transplantation, tolerance remains, for the most part, a concept rather than a reality. As delineated in many of the organ-specific articles in this issue, the current paradigm of lifelong immunosuppression leaves a lot to be desired, particularly for children who face a lifelong burden. Although modern immunosuppression regimens have effectively handled acute rejection, nearly all organs except the liver commonly suffer chronic immunologic damage that impairs organ function, threatening patient and allograft survival. In addition to the imperfect control of the donor-directed immune response, there are additional costs. First, there is the burden of mortality from infection and malignancy that can be directly attributed to a crippled immune system. Second, there are insidious effects on renal function, cardiovascular profile (hypertension, hyperglycemia, and dyslipidemia), bone health, growth, psychological and neurocognitive development, and overall quality of life. It is likely that the full consequences of lifelong immunosuppression on pediatric transplant recipients will not be fully appreciated until survival routinely extends beyond 1 or 2 decades after transplantation. Therefore, it can be argued that the holy grail of transplantation tolerance is of the utmost importance to children who undergo solid organ transplantation.
The alloimmune response
Responses to alloantigens are primarily mediated by host T cells. As naive alloreactive T cells must be activated to cause rejection, they require antigen to be presented on antigen-presenting cells (APCs). Antigen presentation can occur via direct or indirect antigen presentation. In direct antigen presentation, donor APCs leave the graft, migrate to regional lymph nodes, and activate host cells that recognize donor major histocompatibility complex (MHC). Indirect antigen presentation involves recipient APCs presenting peptides derived from donor MHC or other donor-specific proteins, and presenting them to host T cells. Direct allorecognition is believed to be largely responsible for mediating acute rejection. However, chronic rejection is more likely mediated via the indirect pathway, because self-APCs are resident and donor APCs eventually die out. Current strategies for suppressing the immune response to transplanted organs attempt to address both pathways of antigen presentation by suppressing the activation of T cells. However, it is not clear whether tolerance-induction strategies will adequately address both pathways, as protocols that affect direct presentation may not prevent slowly developing chronic rejection mediated by self-APCs continuing to present donor organ antigens.
Definition of tolerance
Tolerance, a state of normal allograft function without histologic evidence of immunologic damage in the complete absence of immunosuppression, can be induced or occur spontaneously. Tolerance induction strategies refer to treatment regimens specifically designed to achieve the tolerant state that is typically delivered around the time of transplantation. In contrast, spontaneous or operational tolerance most often refers to achievement of the tolerant state without an induction regimen that is uncovered through successful withdrawal of immunosuppression. As such, biomarkers capable of identifying and/or monitoring the tolerant state are much needed to enhance the success and decrease the risk of discontinuation of immunosuppression.
Definition of tolerance
Tolerance, a state of normal allograft function without histologic evidence of immunologic damage in the complete absence of immunosuppression, can be induced or occur spontaneously. Tolerance induction strategies refer to treatment regimens specifically designed to achieve the tolerant state that is typically delivered around the time of transplantation. In contrast, spontaneous or operational tolerance most often refers to achievement of the tolerant state without an induction regimen that is uncovered through successful withdrawal of immunosuppression. As such, biomarkers capable of identifying and/or monitoring the tolerant state are much needed to enhance the success and decrease the risk of discontinuation of immunosuppression.
Mechanisms of tolerance
Immunologic tolerance is based on the fundamental premise of immunity, namely self- versus non-self discrimination. Because productive immune responses rely on the immune system’s ability to recognize foreign antigens to protect the host, an elaborate process for ensuring proper recognition of foreign from self-antigens has evolved. To prevent one from responding to one’s own cells and proteins, the immune system uses several mechanisms to induce self-tolerance. These mechanisms are mediated centrally and in the periphery, and are depicted in Fig. 1 .
Central Tolerance
Central tolerance is the primary and most potent checkpoint to educate T and B cells during their development such that cells with high affinity receptors to self-antigens are deleted before they enter the periphery (see Fig. 1 ). For T cells, this process involves education in the thymus. Immature T lineage cells emerge from hematopoietic progenitors in the bone marrow and enter the thymus without expressing either the T cell receptor (TCR) or coreceptors, CD4 and CD8. On entry, these double-negative (DN) thymocytes (they lack CD4 and CD8) commit to 1 of 3 T-cell lineages by undergoing a succession of TCR gene rearrangements. Murine studies indicate that different lineages populate in succession with γδ T cells comprising the first wave during embryogenesis followed by αβ T cells in a second wave. The commitment to the γδ, αβ, or natural killer (NK) T-cell lineage is independent of peptide/MHC interactions.
After lineage commitment, DN thymocytes undergo further development to express CD4 and CD8 receptors, thereby becoming double-positive (DP) thymocytes. It is at this stage that a thymocyte’s fate is determined by the nature of its interaction with self-peptides that are presented on the self-MHC of thymic stromal cells. The overall avidity of the thymocyte’s TCR for self MHC/peptide complexes is based on the TCR structure and its density on the thymocyte cell surface. Thymocytes with TCRs that interact with self MHC/peptides are positively selected and evolve into mature T cells that express either the CD4 or CD8 receptor (single positive T cells). If a T cell then reacts too strongly with self-antigens presented on bone marrow–derived APCs, it is deleted in the thymus. This mechanism of self-tolerance is extraordinarily effective but clearly dependent on the expression of self-peptides by bone marrow–derived APCs in the thymus.
Although it is not entirely clear how, many peripheral tissue-specific antigens are expressed and presented in the thymus to ensure central T-cell tolerance to antigens that will subsequently be encountered in the periphery. Insulin is a prime example. The expression of peripheral proteins in the thymus is driven in part by a gene called AIRE (autoimmune regulator). Mutations in the AIRE gene result in a disease known as autoimmune polyglandular syndrome type I. This condition emphasizes the importance of AIRE in ensuring that peripheral antigens are presented in the thymus to accomplish deletion of autoreactive T cells before their entry into the periphery.
Similarly, B cells are tested for reactivity to self-antigens before they enter the periphery. Immature B cells developing in the bone marrow sample antigen through their antigen receptor, surface IgM (sIgM). If signaling through sIgM is sufficiently weak, immature B cells can be rendered permanently unresponsive or anergic. However, if immature B cells are strongly self-reactive, there are 2 possible scenarios to ensure central tolerance. The first is deletion of these self-reactive B cells. The second is receptor editing, a process by which a new receptor with altered specificity is generated through another sequence of B cell receptor gene rearrangements.
Peripheral Tolerance
Although central tolerance is very effective at eliminating T and B cells with self-reactivity, not all peripheral self-antigens are expressed in the bone marrow and thymus. Moreover, some T or B cells with limited avidity to self-antigens may escape the deletion process. Therefore, peripheral tolerance mechanisms are in place to further ensure that immune responses to self are not initiated or are controlled. Peripheral tolerance either involves induction of activation-induced cell death (AICD), induction of anergy, or regulation of responses to self through regulatory lymphocytes (T or B cells). AICD is a mechanism used to delete T cells specific for self-antigen in the periphery (see Fig. 1 ). T cells that recognize self-antigens with high affinity in the periphery are initially activated and then die via apoptosis. Similarly, mature B cells with a high affinity for self-antigen and thus, highly cross-linked receptors undergo clonal deletion in the periphery.
Anergy is a state of functional inactivation of T or B cells in the periphery. T cell anergy can result from antigenic stimulation in the absence of costimulation. Activation signals generated by engagement of the TCR alone are insufficient to generate IL-2 production and therefore result in an abortive proliferative response. Engagement of CD28 by B7 molecules on APCs along with the TCR is required to induce the multiple pathways that ultimately activate IL-2 gene transcription leading to T cell activation and proliferation. It has been shown that IL-2 production and subsequent signaling through its receptor, IL-2R, is necessary for T cells to escape anergy, as blocking IL-2/IL-2R engagement even after stimulation through TCR and CD28 still results in induction of T cell anergy.
Regulation of immune responses by regulatory T cells (T reg cells) is a third mechanism of peripheral tolerance. T reg cells are active in tolerance to self as well as in regulating adaptive immune responses to foreign antigens. In an adaptive immune response, T reg cells control the type and magnitude of the immune response to foreign antigen to ensure that the host remains undamaged. T reg cells also are integral to maintaining a lack of response to self-antigens or tolerance. There are 2 types of T reg cells, natural and adaptive.
Natural T reg cells are committed to the regulatory lineage in the thymus and may express TCR reactive to peripheral self-antigens not encountered in the thymus. Natural T reg cells are characterized by their expression of CD4 and high levels of CD25 on their surface as well as intracellular expression of the transcription factor Foxp3. Foxp3 is the orchestrator of the cellular and molecular programs involved in mediating T reg cell functions. It is a transcriptional regulatory factor that either directly or indirectly controls hundreds of genes, including signal transduction factors, cytokines, cell-surface receptors, enzymes for cell metabolism, and other proteins. Many of these genes are involved in activation and differentiation of T cells, such as the development of naive T cells into activated effectors. The importance of Foxp3 is underscored by the finding that mutations in this gene in humans causes the immune dysfunction, polyendocrinopathy, enteropathy, X-linked (IPEX) syndrome characterized by lethal autoimmunity.
Adaptive T reg cells are generated from naive T cells in the periphery as determined by the local cytokine milieu. In the presence of transforming growth factor-β (TGF-β), activation of naive T cells leads to expression of Foxp3 which represses the transcriptional apparatus for effector T cells, thereby converting the naive cells into T reg cells. If naive T cells are stimulated by TGF-β in the presence of IL-6, they are converted to Th17 cells, effector T cells that secrete IL-17 and carry out immune tissue injury. However, the presence of retinoic acid, produced by a specialized set of dendritic cells, can block differentiation of naive CD4+ T cells into Th17 cells. IL-2 also promotes T reg cell development. Thus, there is tremendous plasticity in the peripheral development of adaptive T reg cells from naive CD4+ T cells. A complex network of cytokines and developmental lineages has evolved to initiate and regulate proinflammatory responses in the T-cell compartment.
Tolerance in solid organ transplantation: induced versus spontaneous
In the clinical solid organ transplantation arena, it is useful to recognize that the tolerant state can be induced or occur spontaneously. Attempts to induce tolerance are discussed followed by experiences that have unmasked spontaneous tolerance. Induction strategies refer to treatment regimens typically delivered around the time of transplantation designed to achieve tolerance. Protocols exploiting 1 or more of the mechanisms of tolerance have been tried most often in the context of living donor kidney transplantation as candidates are not critically ill and the time of transplantation is known. No induction protocols have, as yet, included pediatric transplant candidates reflecting the preliminary nature of these efforts as well as the hesitation to expose children to highly experimental therapies. Nevertheless, it is instructive to consider the approaches that have been tried.
Induction of tolerance: central mechanisms
In transplantation, induction of central tolerance has been attempted through the induction of donor-recipient chimerism. In murine models, bone marrow chimeras, achieved by hematopoietic stem cell transplantation after host myelosuppression, are tolerant to skin grafts from the same donor. In these model systems, donor stem cells were shown to migrate to the thymus, leading to negative selection or elimination of newly emerging donor-specific T cells. These and other similar studies in nonhuman primates set the stage for recent clinical trials aimed at achieving chimerism as a tolerance induction strategy.
There are several key components to regimens designed to induce donor-recipient chimerism. First the recipient must undergo some form of nonablative myelosuppression or conditioning to facilitate engraftment of donor hematopoietic cells while allowing ultimate survival of and repopulation by host hematopoietic cells. Total lymphoid irradiation and cyclophosphamide are 2 approaches that are actively being tested. Second, the immunosuppression regimen is designed to prevent rejection of the donor organ as well as graft-versus-host disease (GVHD), a risk of achieving chimerism particularly in the setting of HLA mismatch. T cell depletion, most commonly using a polyclonal preparation against T cells, has been the favored induction approach, followed by a period of standard immunosuppression before complete withdrawal. There are 1 or more infusions of donor bone marrow cells, either unsorted or enriched for hematopoietic stem cells.
Currently, a few centers have used the chimerism approach to induce central tolerance in conjunction with adult kidney transplantation, with mixed success. The group from Stanford University has used total lymphoid irradiation with induction using rabbit antithymocyte globulin followed by maintenance immunosuppression with corticosteroids and cyclosporine. Of 4 HLA-mismatched, adult, living donor kidney transplant recipients treated, none achieved long-term chimerism or tolerance. More recently, the same group reported on a single adult patient who underwent HLA-identical combined stem cell and kidney transplantation after total lymphoid irradiation followed by maintenance immunosuppression with cyclosporine and mycophenolate mofetil. This patient showed persistent mixed chimerism, experienced neither allograft rejection nor GVHD, and was successfully withdrawn from all immunosuppression maintaining normal kidney function for 34 months after transplantation. However, 2 other patients achieved only transient chimerism such that immunosuppression has been maintained. The protocol has been modified with intensification of the irradiation given for conditioning to enhance chimerism but results are, as yet, unpublished ( clinicaltrials.gov identifier NCT00319657).
The best results to date have been achieved by the group at Massachusetts General Hospital. Initially, they reported on 6 adults undergoing combined bone marrow and kidney transplantation for multiple myeloma and end-stage renal disease (ESRD), again from HLA-identical donors. All 6 patients developed evidence of mixed chimerism. Notably, the 2 patients who achieved full and durable donor chimerism developed GVHD that required treatment with maintenance immunosuppression. The other 4 showed only transient chimerism. When these 4 patients were weaned off immunosuppression, 1 experienced acute rejection that was treated with immunosuppression and the other 3 were tolerant to their kidney allografts, remaining off immunosuppression for 1.3 to 7 years after transplantation. Subsequently, the protocol was modified to accommodate ESRD candidates with single haplotype HLA-mismatched donors. All 5 adults enrolled in the protocol developed only transient mixed chimerism. One of the 5 recipients suffered irreversible humoral rejection leading to graft loss. However, 4 have successfully withdrawn from immunosuppression, maintaining stable kidney function for 2.0 to 5.3 years after transplantation or 1.2 to 4.6 years after cessation of immunosuppression. All 4 tolerant recipients showed donor-specific hyporesponsiveness with high levels of Foxp3 transcripts in allograft tissue. Currently, a further modification of the protocol to accommodate complete HLA-mismatched donor-recipient combinations is undergoing testing ( clinicaltrials.gov identifier NCT00801632).
Although the risks inherent in current chimerism approaches to induce central tolerance are substantial because of the requisite conditioning regimen and the risk of GVHD, this strategy may be especially promising for the pediatric population. Children have the advantage of a larger thymus which, in preclinical studies, correlates with better prospects to achieve a more robust state of tolerance. With aging, the thymus involutes leading to diminished capacity to educate T cells and thereby participate in central tolerance mechanisms.
Induction of tolerance: peripheral mechanisms
Even though commercial drug development programs do not aim to induce tolerance, recently developed therapies strongly reflect various approaches that may enhance mechanisms of peripheral tolerance. The strategy of T-cell depletion, most frequently using rabbit antithymocyte globulin has gained substantial popularity as induction immunosuppression for adult organ transplantation. Although the primary aim is to reduce the rate of acute rejection, depletion may indeed be pro-tolerogenic as T cell repopulation in the presence of the donor organ is believed to facilitate donor-specific hyporesponsiveness. A second attractive approach has been costimulatory blockade that leads to anergy of donor-specific T cells. LEA29Y, a humanized monoclonal antibody targeting the CD28-B7 costimulation pathway, is currently being tested in adult kidney and liver transplantation registration trials as a maintenance immunosuppression agent to replace calcineurin inhibitors ( clinicaltrials.gov identifiers NCT00455013, NCT00035555, NCT00256750, NCT00114777, and NCT00555321). Another costimulatory blockade agent, anti-CD154, that interferes with CD40/CD40L interaction, held substantial promise for tolerance induction until trials revealed an increased risk of thromboembolic events and commercial development has ceased.
Another avenue of peripheral tolerance induction that is reflected in novel immunosuppression strategies is the enhancement of T reg cells. There is evidence for expansion of alloantigen cross-reactive natural T reg cells and conversion of alloantigen-specific non-T reg cells as part of the mechanisms that regulate antidonor responses. Recent studies have pointed to the potential of certain immunosuppression regimens that seem to induce production of T reg cells. In vitro, rapamycin has been shown selectively expand CD4+CD25+Foxp3+ T reg cells. The underlying target for rapamycin, mTOR or mammalian target of rapamycin, is a key player in signals generated via the TCR. Constitutive activation of the mTOR pathway antagonizes expression of FoxP3, thus inhibiting T reg development. Conversely, blocking mTOR leads to de novo differentiation of naive CD4+ T cells into CD4+FoxP3+ regulatory T cells. These findings have been confirmed in vivo; murine studies have shown that rapamycin promotes T reg cell development, whereas calcineurin inhibitors inhibit their development.
In the clinical setting, however, it is unclear whether the T reg mechanism prevails in spontaneous or operational tolerance. One study by Yoshizawa and colleagues reported donor alloantigen-specific T reg cells as defined by CD4+CD25+ expression in 14 pediatric liver transplant recipients who had been successfully weaned off immunosuppression. However, functional studies did not suggest that T reg cells were the primary means of anti-donor regulation. Even in the absence of CD4+CD25+ T reg cells, effector (CD4+CD25−) T cells showed donor-specific hyporesponsiveness during in vitro mixed lymphocyte reactions. Moreover, several studies exploring biomarkers of operationally tolerant kidney and liver transplant recipients have not shown a definitive role for T reg cells (see section on Markers of Tolerance in Solid Organ Transplantation).
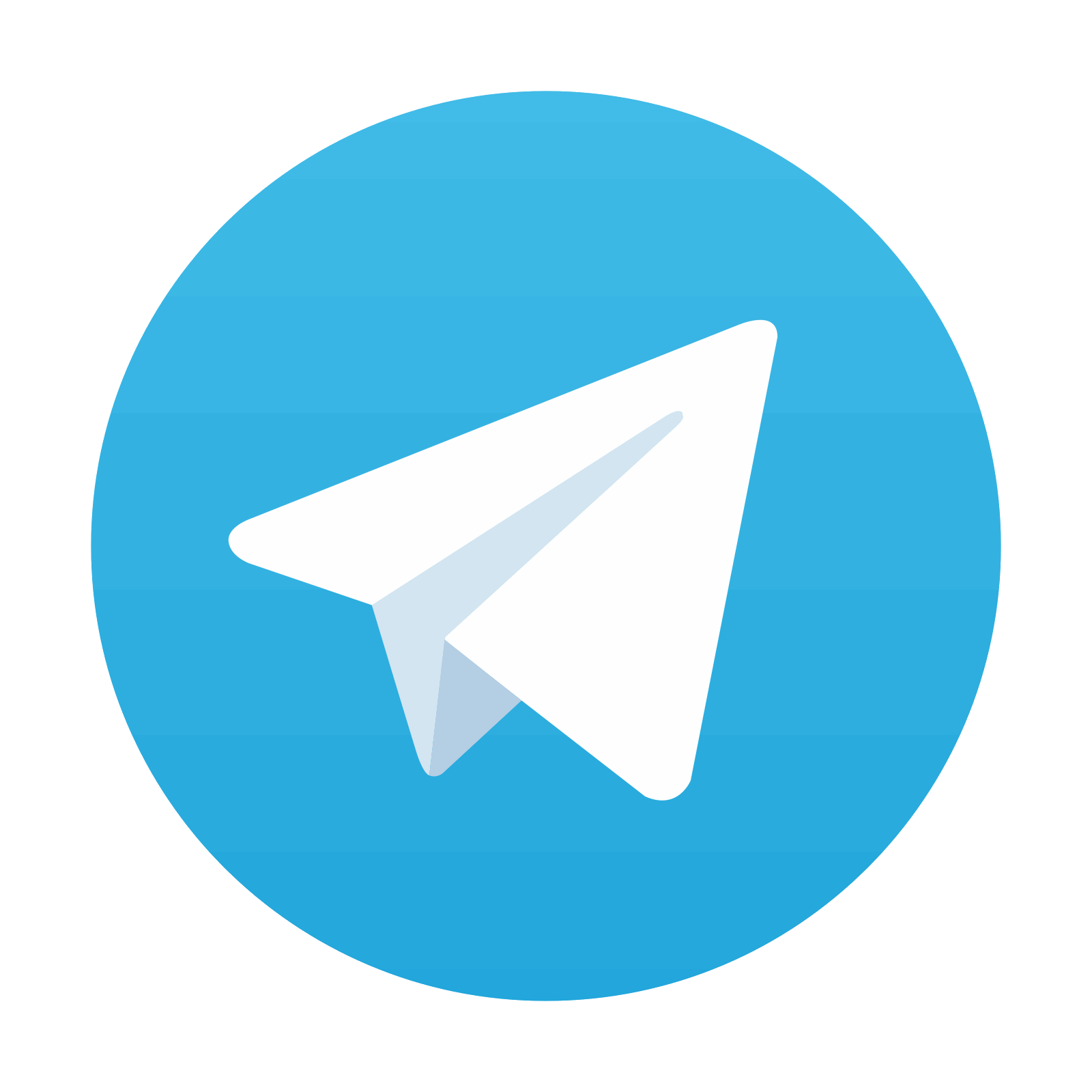
Stay updated, free articles. Join our Telegram channel

Full access? Get Clinical Tree
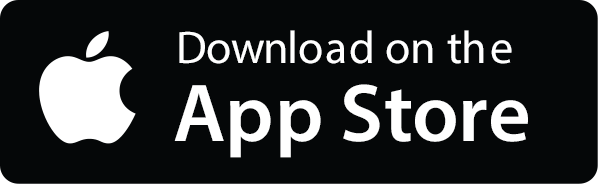
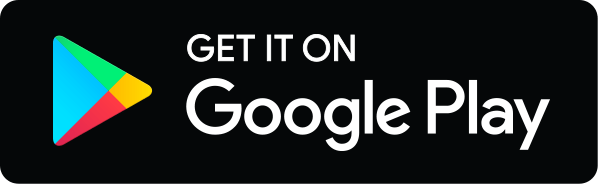