In recent years, translational research with various animal models has been helpful to answer basic questions about the effect of antenatal inflammation on maturation and development of the fetal lung and immune system. The fetal lung and immune systems are very plastic and their development can be conditioned and influenced by both endogenous and/or exogenous factors. Antenatal inflammation can induce pulmonary inflammation, leading to lung injury and remodeling in the fetal lung. Exposure to antenatal inflammation can induce interleukin-1α production, which enhances surfactant protein and lipid synthesis thereby promoting lung maturation. Interleukin-1α is therefore a candidate for the link between lung inflammation and lung maturation, preventing respiratory distress syndrome in preterm infants. Antenatal inflammation can, however, cause structural changes in the fetal lung and affect the expression of growth factors, such as transforming growth factor-beta, connective tissue growth factor, fibroblast growth factor-10, or bone morphogenetic protein-4, which are essential for branching morphogenesis. These alterations cause alveolar and microvascular simplification resembling the histology of bronchopulmonary dysplasia. Antenatal inflammation may also affect neonatal outcome by modulating the responsiveness of the immune system. Lipopolysaccharide-tolerance (endotoxin hyporesponsiveness/immunoparalysis), induced by exposure to inflammation in utero, may prevent fetal lung damage, but increases susceptibility to postnatal infections. Moreover, prenatal exposure to inflammation appears to be a predisposition for the development of adverse neonatal outcomes, like bronchopulmonary dysplasia, if the preterm infant is exposed to a second postnatal hit, such as mechanical ventilation oxygen exposure, infections, or steroids.
Preterm birth, defined as less than 37 completed weeks (259 completed days) of gestation, remains one of the largest health problems in the world despite advances in perinatal clinical care. Up to 13% of all live deliveries are preterm in developed countries. Spontaneous preterm birth can be initiated by various mechanisms, including uterine overdistension, uteroplacental ischemia, allergenic phenomena, endocrine disorders, abnormal allograft reaction, cervical disease, infection, or inflammation. Chorioamnionitis, an intraamniotic (IA) inflammation of the chorioamniotic membranes, is one of the leading factors associated with preterm birth. The incidence of histologic chorioamnionitis has been reported to be up to 45-60% in very low birthweight (VLBW) infants. Microorganisms associated with chorioamnionitis are mainly of low virulence, including Ureaplasma and Mycoplasma species. Not only bacteria but also viruses have been identified as possible inducers of chorioamnionitis. Recent studies suggested that not a single microorganism but multiple bacterial species may be responsible for the induction of intrauterine inflammation. However, microbial presence in the intraamniotic environment does not necessarily induce preterm birth. In up to 70% of women undergoing cesarean section at term, microbial presence in the placental membranes could be detected.
Chorioamnionitis can be subdivided into clinical chorioamnionitis and histologic chorioamnionitis. Clinical chorioamnionitis usually presents itself with a systemic inflammatory response of the mother with increased leukocyte counts, increased C-reactive protein, local symptoms (uterine tenderness and vaginal discharge), and fever, which is treated by delivery of the fetus and antibiotic therapy. However, the predominant form of chorioamnionitis, referred to as histologic chorioamnionitis, shows no maternal symptoms, making the prenatal diagnosis of histologic chorioamnionitis a major clinical challenge. Histologic chorioamnionitis can be diagnosed by histologic examination of the placenta and fetal membranes. Histologic examination is based on the response of the immune system with the detection of polymorphonuclear leukocytes. In addition, microbial invasion of the amniotic cavity (MIAC) may be confirmed by microbiologic (positive culture/polymerase chain reaction for pathogens) or molecular biologic (immunofluorescence and fluorescent in situ hybridization) assays performed on the same tissues. Through histologic examination, chorioamnionitis can be further differentiated into “acute” or “chronic” chorioamnionitis, depending on whether the membranes are infiltrated by neutrophils (acute) or lymphocytes (chronic) of fetal and maternal origin. In contrast to acute chorioamnionitis, chronic chorioamnionitis has cellular and humoral components, which reflect antifetal rejection. Histologic examination is generally regarded as the “gold standard” in the diagnosis of histologic chorioamnionitis. However, the diagnosis “histologic chorioamnionitis” can only be made if the inflammatory response is already present, which is not always the case after MIAC. The inflammatory response can be suppressed because of medication like corticosteroids, possible immaturity of the fetal immune system or in case of very early microbial invasion of the amnion. A combined diagnostic approach using polymerase chain reaction (PCR), culture, cytokine analysis and histologic examination could therefore drastically improve the accuracy of the diagnosis of IA inflammation/infection.
If MIAC is present, the fetus can be exposed through direct contact with amniotic fluid or through the placental–fetal circulation. The fetal response to MIAC is commonly referred to as the fetal inflammatory response syndrome (FIRS), which typically involves systemic inflammation and an increase of fetal plasma interleukin (IL) 6 concentrations. Other proinflammatory cytokines, such as IL-1 and tumor necrosis factor (TNF) α have also been found to be elevated in the blood of newborns diagnosed with FIRS. FIRS can lead to fetal multiorgan impairment involving the umbilical cord, lungs, brain, eyes, thyroid, heart, gut, liver, thymus, and the fetal immune system. Although fetal and neonatal effects of IA inflammation/infection are wide-ranging. this review will specifically focus on the interaction between IA inflammation/infection, and the fetal lungs and immune system.
Animal models to study intraamniotic inflammation/infection
In the last years, translational research with various animal models has been helpful to answer some basic questions about the effect of IA inflammation/infection on different fetal organs. Rodent, as well as rabbit, guinea pig, and sheep models have been used to study the effects of pre- (eg, inflammation) and postnatal (eg, mechanical ventilation) events on pulmonary development and injury. However, the various animal models differ in their developmental biology compared with humans. For example, the alveolar phase of lung development starts prenatally in humans and sheep, but postnatally in rodents. This entails that rodent alveolar development commences during exposure to atmospheric oxygen levels and respiratory activity, compared with the relative hypoxia and absence of respiratory functionality in utero in sheep and humans. Moreover, the importance of amniotic fluid/fetal lung fluid for human fetal lung development has been well described. Despite these developmental advantages of large animals over rodents, rodent models offer the possibility to use transgenic approaches to study candidate genes in the pathogenesis of IA inflammation/infection. Therefore, both animal models are useful to study the effects of intraamniotic infection/inflammation on lung development. In future large animal research, conditional overexpression or knockdown of candidate genes affected by IA inflammation/infection would allow a more accurate identification of genes leading to morbidity in preterm infants.
Effects of intraamniotic inflammation/infection on the fetal lung
The pathologic sequelae in the fetal lung following diagnosed histologic chorioamnionitis are an example of the complexity of the effect of inflammation on an immature developing organ. The impact of the immune system on the developing lung is further influenced by corticosteroid exposure before preterm birth, and postdelivery management, such as ventilation and oxygen supplementation. Preterm birth is highly associated with the development of respiratory distress syndrome (RDS) and bronchopulmonary dysplasia (BPD), caused by structural and functional immaturity of the premature lung. Watterberg et al were the first to show an intriguing ambivalent association between chorioamnionitis and respiratory morbidity. In ventilated preterm infants exposed to prenatal inflammation, they were able to show a decrease in RDS but a subsequent increase in BPD, which was later defined as a need for oxygen at 36 weeks postmenstrual age or home discharge. Many studies, but not all, have reproduced the association between a short-term beneficial effect of histologic chorioamnionitis on the incidence and severity of RDS, and a susceptibility of the lung for further postnatal injury, which may predispose for BPD. These clinical observations and some of the underlying pathophysiologic mechanisms have been confirmed in different experimental models of IA inflammation/infection ( Figure 1 ).

Effects of intraamniotic inflammation/infection on the fetal lung
The pathologic sequelae in the fetal lung following diagnosed histologic chorioamnionitis are an example of the complexity of the effect of inflammation on an immature developing organ. The impact of the immune system on the developing lung is further influenced by corticosteroid exposure before preterm birth, and postdelivery management, such as ventilation and oxygen supplementation. Preterm birth is highly associated with the development of respiratory distress syndrome (RDS) and bronchopulmonary dysplasia (BPD), caused by structural and functional immaturity of the premature lung. Watterberg et al were the first to show an intriguing ambivalent association between chorioamnionitis and respiratory morbidity. In ventilated preterm infants exposed to prenatal inflammation, they were able to show a decrease in RDS but a subsequent increase in BPD, which was later defined as a need for oxygen at 36 weeks postmenstrual age or home discharge. Many studies, but not all, have reproduced the association between a short-term beneficial effect of histologic chorioamnionitis on the incidence and severity of RDS, and a susceptibility of the lung for further postnatal injury, which may predispose for BPD. These clinical observations and some of the underlying pathophysiologic mechanisms have been confirmed in different experimental models of IA inflammation/infection ( Figure 1 ).
Mechanisms involved in lung maturation after intraamniotic inflammation
The maturation effect of IA inflammation is likely mediated by proinflammatory cytokines, such as IL-1 (α and β), IL-6, IL-8, and TNF-α, which directly affect fetal lung cells, including alveolar type II cells. Bry et al reported that intraamniotic IL-1α could enhance messenger RNA (mRNA) transcription of surfactant protein (SP) SP-A, SP-B and surfactant lipids in fetal rabbits. This resulted in a better compliance of the lung in IL-1α exposed fetal rabbits, indicative of a maturational response. Interestingly, the maturational effect of IL-1α appears to depend on the gestational age. Increased mRNA transcription of surfactant proteins could only be seen in rabbit lung explants from 19/22-day fetuses, as SP mRNA’s decreased in IL-1α exposed lung explants from 27/30-day fetuses (term = 30 days). Similar to fetal rabbits, the fetal sheep lung responded with increased levels of SP mRNA’s (SP-A, SP-B, SP-C, and SP-D) and lipids (saturated phosphatidylcholine), and improved compliance after IA injection of IL-1α, IL-1β, or lipopolysaccharides (LPS). The maturation response to IL-1α was greater than that to IL-1β, which was similar to LPS response. Conversely, blockade of IL-1 signaling following LPS exposure partially inhibited fetal lung and systemic inflammation and lung maturation. Interestingly, in transgenic mice, perinatal expression of IL-1β in lung epithelial cells with a doxycycline-inducible system caused a lung disease that was clinically and histologically similar to BPD, underlining the important role of IL-1β in IA inflammation-mediated lung injury. Also in other antenatal inflammation/infection sheep models, in which the fetus was exposed to Ureaplasma species or intravenous LPS, a maturation effect on the surfactant system was described in combination with elevated IL-1β levels.
It remains unclear by which mechanisms IL-1α and β achieve its maturational effects. Although both LPS and antenatal corticosteroids induce a maturational response, intraamniotic LPS did not enhance fetal plasma cortisol, indicating that other mediators than cortisol are responsible for LPS induced lung maturation. Interestingly, combined prenatal exposure to LPS and the corticosteroid betamethasone—a common clinical situation—has an additive effect on surfactant pool size and increases lung compliance in vivo. Prenatal betamethasone treatment before IA LPS exposure suppressed lung inflammation, but only modestly induced maturation responses in the fetal lung. However, when prenatal betamethasone treatment was started after the exposure to IA LPS, lung inflammation was not prevented and the largest maturation response was achieved. This demonstrated that the time point of steroid application in relation to the initiation of infection seems be important in relation to the maturational effects of corticosteroids.
Lung developmental pathways affected by intraamniotic inflammation
Although clinical and experimental data consistently show that exposure to IA inflammation prevents RDS, the correlation between intraamniotic inflammation and impaired lung development as a risk factor for BPD, is more controversial. Lung development is orchestrated by several pathways, which have been elaborately characterized. Conversely, how these lung developmental pathways are affected by IA inflammation is still poorly understood. BPD is characterized by a disruption of lung alveolarization and vascularization, leading to fewer but larger alveoli and a simplified vascular structure. Several aberrant and interacting signaling pathways of the lung mesenchyme contribute to BPD development. IA inflammation can spread to the fetal lung, leading to lung injury and remodeling. In fetal sheep this resulted in persistent structural changes in the fetal lung, similar to BPD. Antenatal inflammation altered the distribution of elastin, a mesenchymal structural protein required for normal alveolar septation. The potential of inflammatory signaling to disturb the expression of growth factors, which are critical for lung morphogenesis, is illustrated by the down-regulation of FGF-10 (fibroblast growth factor) by nuclear factor kappa-light-chain-enhancer of activated B cells (NF-κB)-activation. Throughout lung development, FGF-10 and BMP-4 (bone morphogenetic protein) are consistently expressed at the distal tips of outgrowing lung buds, and are crucial factors for branching morphogenesis, repair, remodeling, and regeneration.
Antenatal inflammation can also induce vascular remodeling in pulmonary vessels, including hypertrophy of the smooth muscle, enhanced deposition of collagen, and inhibition of endothelial cell proteins and endothelial growth factor expression, including VEGF (vascular endothelial growth factor), angiopoietin-1, TGF-β (transforming growth factor beta), endoglin, CTGF (connective tissue growth factor), eNOS (endothelial nitric oxide synthase), PECAM-1 (platelet endothelial cell adhesion molecule 1), TIE-2 (tyrosine kinase with immunoglobulin-like and EGF-like domains 2), or VEGF receptor 2 ( Figure 1 ). Like all of the previously mentioned factors, VEGF has an important function in vascular and alveolar lung development, and was reduced in the lungs of infants dying with BPD. Physiologic observations in preterm sheep exposed to intrauterine inflammation are in line with these findings, showing decreased oxygenation and signs of pulmonary arterial hypertension. Experiments on fetal mouse lung explants suggested that the altered microvasculature observed in the lungs of BPD patients could be the result of aberrant capillary formation after IA inflammation/infection.
The TGF-β/Smad signaling pathway is one of the key pathways involved in lung development, airway inflammation, airway remodeling and lung fibrosis. All of these processes contribute to the development of BPD. Antenatal inflammation can induce TGF-β expression, activate Smad signaling, and alter CTGF expression in the fetal sheep lung. CTGF, a downstream mediator of TGF-β, plays an important role in airway/vascular remodeling and has been implicated in the pathogenesis of BPD. Previous studies have demonstrated that hyperoxia up-regulates CTGF expression in neonatal rat lungs, although IA LPS exposure reduced pulmonary CTGF expression in ventilated preterm lambs. Increased CTGF expression has been reported to induce BPD features in preterm mouse pups. Although increased CTGF and TGF-β activity has been associated with inhibition of lung development, equally convincing evidence suggests that there must be a minimum level of activity of both CTGF and TGF-β for normal lung development. Throughout lung development TGF-β functions as a negative regulator to control branching morphogenesis by inhibiting FGF-10, and is expressed at the bifurcation sites of branching airways to facilitate the outgrowth of separate airways. These insights underline that this delicate balance of growth factors is necessary during normal lung morphogenesis.
Other signaling pathways involved in lung development and remodeling include the Wnt (Wingless-Int) and Shh (Sonic Hedgehog) signaling pathways. Wnt-signaling modulates cell proliferation, polarity, and differentiation, and is essential for branching and epithelial-mesenchymal interactions during early lung development. After lung inflammation and fibrosis Wnt-signaling regulates remodeling and reparative reactions. The Shh pathway is closely involved in the regulation of lung development, by regulating the expression of growth factors (FGF-10 and BMP-4), which can influence branching and myofibroblast differentiation. Recent data showed that intraamniotic LPS exposure disrupted both Wnt and Shh pathways, reduced BMP-4 and FGF-10 expression, and impaired the expression of matrix proteins crucial for normal alveolar lung development. Shh expression is induced by retinoic acid, which is decreased in infants who develop BPD. Moreover, IA LPS exposure decreased retinoic acid in fetal sheep lungs. This decrease of retinoic acid could therefore cause a decrease of the Shh pathway, leading to the disrupted lung development seen in BPD.
All of the above mentioned growth factors and their signaling pathways form a delicately regulated network, which interact with each other to control lung development. Interactions between TGF-β, Shh, Wnt, FGFs, and BMPs have been described at multiple levels, both in normal lung development, remodeling, and disease. Because of their importance in both lung development and remodeling, and likely also the development of BPD, regulatory molecules of these pathways may be of special interest for the development therapeutic interventions for BPD. CTGF, for example, can induce Wnt-signaling, reciprocally switch on Smad signaling, and inhibit VEGF. Caveolin-1 (Cav-1) is a negative regulator of TGF-β receptors, which is regulated by TGF-β. Through its effect on TGF-β receptor turnover, Cav-1 is implicated as another possible modulator of lung injury and remodeling by affecting signaling pathways like the Smad, Stat, and the a-SMase/ceramide pathway, all involved in remodeling. A strong down-regulation of Cav-1 has been reported after intraamniotic LPS exposure in fetal sheep lungs.
Antenatal inflammation can induce immune tolerance
The fetal lung is not only vulnerable to IA inflammation/infection because of its structural immaturity, but also because of the immaturity of its immune defenses. A normal healthy lung is a primary barrier against microbial invaders, for which it relies on the synergistic cooperation between the alveolar and circulatory compartments and the immune system. The fetal immune system may, however, not always mount a defense to microbial invaders. Evidence for this is provided by the observation that chronic exposure of 70 days to Ureaplasma parvum in utero did not cause sustained effects on air space or vascular development in premature lambs. More specifically, it seems that the fetal immune system is unable to mount an inflammatory response against (components of) microbes if it has dealt with an immunologic challenge in utero. For example, blood monocytes, which had been isolated from fetal sheep exposed to IA LPS, did not respond to a second immunologic challenge in vitro. This same observation was recently confirmed in blood monocytes of preterm infants that had been diagnosed with histologic chorioamnionitis.
In the fetal sheep lung, maturation of blood-derived monocytes into alveolar macrophages could be induced by a single dose of IA LPS. These macrophages were able to respond to inflammatory stimuli similar to adult macrophages. Interestingly, repetitive injections of LPS initiated a paralysis of the fetal immune system in the lung and blood, called LPS-tolerance (endotoxin hyporesponsiveness/immunoparalysis) ( Figure 2 ). This immunoparalysis could be mediated by a reduced expression of the major histocompatibility complex (MHC) class II antigen on fetal blood monocytes and a systemic or local elevated expression of the 2 immunosuppressive cytokines, IL-10 and TGF-β, in amniotic fluid, cord blood, or in fetal lung. This tolerance could also be detected after stimulation with other proinflammatory ligands of Toll-like receptors, which are key activators of the immune system, or even by chronic exposure to living microorganisms like Ureaplasma species. LPS-tolerance was associated with differential expression of isomers of the IL-1 receptor associated kinase (IRAK) family. IRAK-4 promotes the signal transduction to activation of NF-κB, whereas IRAK-M inhibits the signal transduction. In LPS-tolerant cells we found a low expression of IRAK-4 and a high expression of IRAK-M in the presence of unchanged Toll-like receptors. LPS tolerance could be an adaptive mechanism of the fetal immune system to react to repetitive inflammation. Because the fetal pulmonary response to injury, like inflammation, depends on the intensity of the injury, it could be possible that immune tolerance represents a natural defensive mechanism to prevent organ injury ( Figure 2 ). Such a mechanism would explain why a prolonged exposure to IA LPS or microorganisms only caused a moderate persistent inflammation and did not lead to progressive structural abnormalities in the lung.
Immune suppression induced by prenatal infection could be a serious disadvantage for preterm infants, who will be more vulnerable to postnatal second hits such as sepsis, ventilation induced injury, and respiratory infections ( Figure 2 ). Preterm infants who have been diagnosed with histologic chorioamnionitis are more likely to develop early onset sepsis, an observation that was recently confirmed in a clinical study. Moreover, BPD patients who were born preterm are frequently admitted to hospitals with respiratory infections during the first years of their lives, not only because of abnormal lung function but also due to long-term immune dysfunction. A key contributor to the dysfunction of the fetal immune system could be the involution of the thymus, which has been observed after IA exposure to inflammation. Thymus size is markedly decreased in size in preterm infants diagnosed with clinical chorioamnionitis. In a histologic study on thymi of fetuses with severe clinical chorioamnionitis, a reduced corticomedullary ratio, a decreased number of thymocytes, and histologic features of degenerative processes were described. Interestingly, thymic involution by fetal stress has been also associated with the development of BPD in preterm infants. A small thymus detected at birth by routine chest radiograph was predictive for the development of BPD in VLBW preterm infants, suggesting that immune mechanisms that contribute to lung injury and BPD begin antenatally. By using a model of LPS-induced IA inflammation, the induction of a fetal inflammatory response resulted in a change in thymus structure with increased thymic levels of CD4, CD8, and CD4/CD25 positive T lymphocytes. FoxP3 positive regulatory T lymphocytes, which have an important suppressive role in the immune system, were decreased in the fetal sheep thymus after intrauterine LPS exposure. Although the observations between the human and animal study with regard to T lymphocyte levels appear contradictory, this may have been the result of different inflammation intensities, which was probably significantly higher in the study of Toti and colleagues. It appears that, during fetal life, the developing immune system is especially vulnerable to a variety of factors, including infection and inflammation, affecting immune reactions later in life.
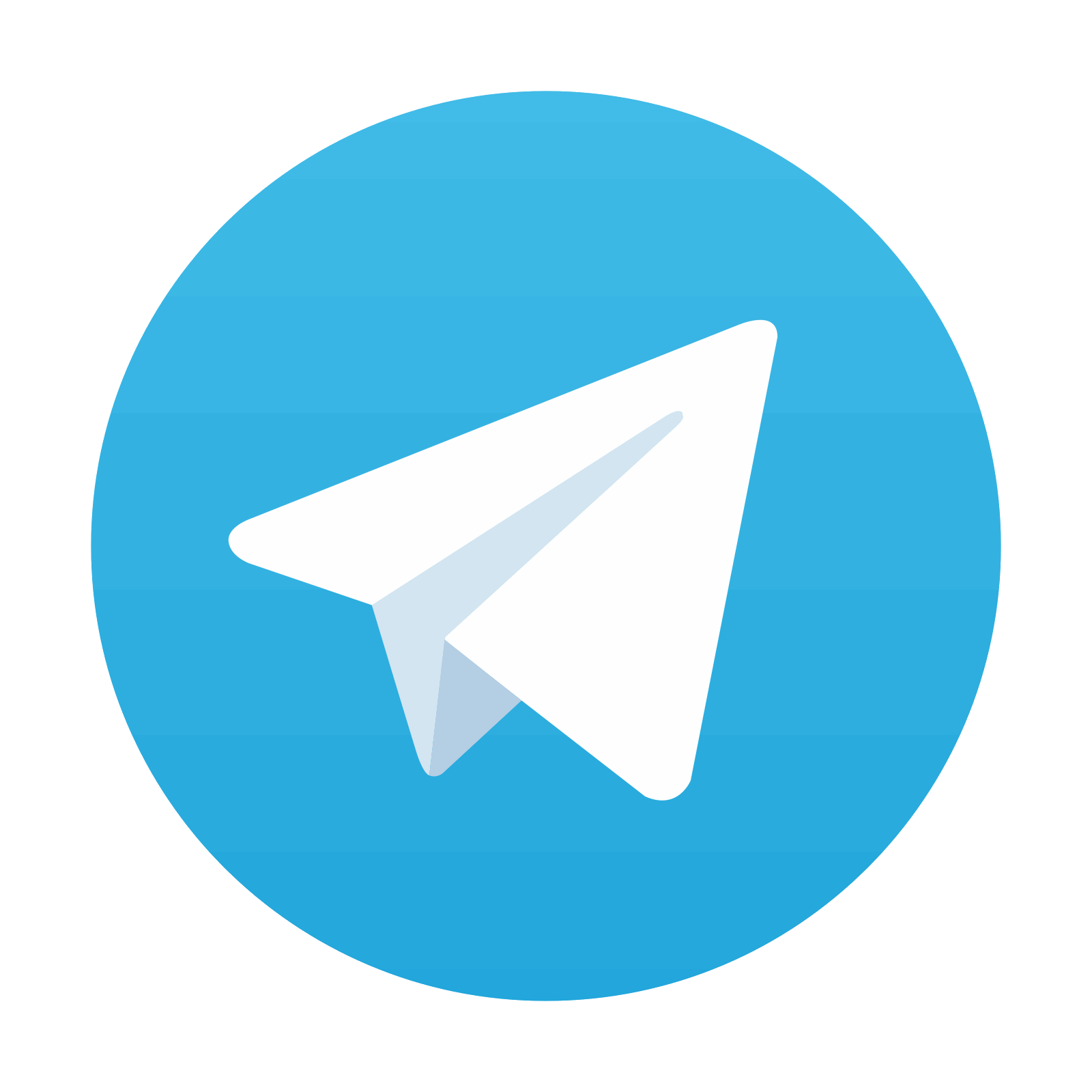
Stay updated, free articles. Join our Telegram channel

Full access? Get Clinical Tree
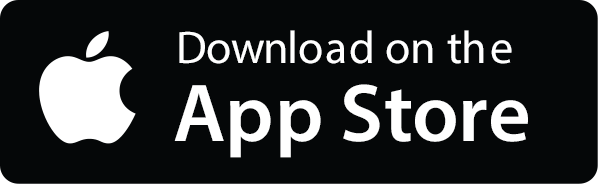
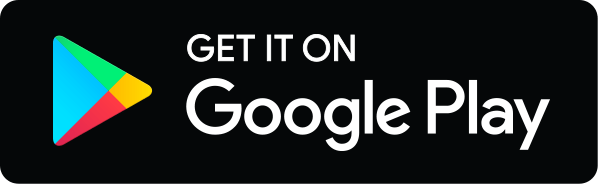