Rapid development of the embryo from the trilaminar embryonic disc during the third week (see Fig. 4.3 H ) is characterized by:
- •
Appearance of primitive streak
- •
Development of notochord
- •
Differentiation of three germ layers
The third week of development coincides with the week after the first missed menstrual period, that is, 5 weeks after the first day of the last normal menstrual period. Cessation of menstruation is often the first indication that a woman may be pregnant. Approximately 5 weeks after the last normal menstrual period, a normal pregnancy can be detected with ultrasonography ( Fig. 4.1 ).

Frequent symptoms of pregnancy are nausea and vomiting, which may occur by the end of the third week; however, the time of onset of these symptoms varies. Vaginal bleeding at the expected time of menstruation does not rule out pregnancy because sometimes there is some loss of blood from the implantation site of the blastocyst. Implantation bleeding results from leakage of blood from the closing plug into the uterine cavity from disrupted lacunar networks in the implanted blastocyst (see Chapter 3 , Figs. 3.2 A and 3.5 A ). When bleeding is interpreted as menstruation, an error occurs in determining the expected delivery date of the fetus.
Gastrulation: Formation of Germ Layers
4
Gastrulation is a formative process by which the three germ layers, which are precursors of all embryonic tissues, and the axial orientation are established in embryos. During gastrulation, the bilaminar embryonic disc is converted into a trilaminar embryonic disc (see Fig. 4.3 H ). Extensive cell-shape changes, rearrangement, movement, and alterations in adhesive properties contribute to the process of gastrulation.
Gastrulation is the beginning of morphogenesis (development of body form) and is the most significant event occurring during the third week. During this week, the embryo is referred to as a gastrula . Bone morphogenetic proteins and other signaling molecules such as FGFs, Shh (sonic hedgehog), Tbx16, Tgifs, and Wnts play a critical role in gastrulation.
Each of the three germ layers (ectoderm, mesoderm, and endoderm) ( Fig. 4.2 ) gives rise to specific tissues and organs:
- •
Embryonic ectoderm gives rise to the epidermis, central and peripheral nervous systems, eyes and internal ears, neural crest cells, and many connective tissues of the head.
- •
Embryonic mesoderm gives rise to all skeletal muscles, blood cells, the lining of blood vessels, all visceral smooth muscular coats, serosal linings of all body cavities, ducts and organs of the reproductive and excretory systems, and most of the cardiovascular system. In the body (trunk or torso), excluding the head and limbs, it is the source of all connective tissues, including cartilage, bones, tendons, ligaments, dermis, and stroma (connective tissue) of internal organs.
- •
Embryonic endoderm is the source of the epithelial linings of the respiratory and alimentary (digestive) tracts, including the glands opening into the gastrointestinal tract, and glandular cells of associated organs such as the liver and pancreas.

Primitive Streak
4
The first morphologic sign of gastrulation is the formation of the primitive streak on the surface of the epiblast of the bilaminar embryonic disc ( Fig. 4.3 A to C ). By the beginning of the third week, this thickened linear band of epiblast appears caudally in the median plane of the dorsal aspect of the embryonic disc ( Fig. 4.4 A and B , and see Fig. 4.3 C ). The primitive streak results from the proliferation and movement of cells of the epiblast to the median plane of the embryonic disc. As soon as the primitive streak appears, it is possible to identify the embryo’s craniocaudal axis, cranial and caudal ends, dorsal and ventral surfaces, and right and left sides. As the streak elongates by addition of cells to its caudal end, its cranial end proliferates to form the primitive node (see Figs. 4.3 E and F and 4.4 A and B ).


Concurrently, a narrow groove, the primitive groove , develops in the primitive streak that is continuous with a small depression in the primitive node, the primitive pit . The primitive groove and pit result from the invagination (inward movement) of epiblastic cells, which is indicated by arrows in Fig. 4.3 E .
Shortly after the primitive streak appears, cells leave its deep surface and form mesenchyme , an embryonic connective tissue consisting of small, spindle-shaped cells loosely arranged in an extracellular matrix (intercellular substance of a tissue) of sparse collagen (reticular) fibers ( Fig. 4.5 B ). Mesenchyme forms the supporting tissues of the embryo , such as most of the connective tissues of the body and the connective tissue framework of glands. Some mesenchyme forms mesoblast (undifferentiated mesoderm), which forms intraembryonic mesoderm (see Fig. 4.3 D ).

Cells from the epiblast, as well as from the primitive node and other parts of the primitive streak, displace the hypoblast, forming embryonic endoderm in the roof of the umbilical vesicle (see Fig. 4.3 H ). The cells remaining in the epiblast form the embryonic ectoderm .
Research data suggest that signaling molecules (nodal factors) of the transforming growth factor-β superfamily induce the formation of mesoderm. The concerted action of other signaling molecules (e.g., Wnt3a, Wnt5a, and FGFs) also participates in specifying the fates of the germ cell layers. Moreover, transforming growth factor-β (nodal), a T-box transcription factor (veg T), and the Wnt signaling pathway appear to be involved in the specification of the endoderm.
Mesenchymal cells derived from the primitive streak migrate widely. These pluripotential cells differentiate into diverse types of cells, such as fibroblasts, chondroblasts, and osteoblasts (see Chapter 5 ). In summary, cells of the epiblast, through the process of gastrulation, give rise to all three germ layers in the embryo, the primordia of all its tissues and organs.
Fate of Primitive Streak
The primitive streak actively forms mesoderm by the ingression (entrance) of cells until the early part of the fourth week; thereafter, production of mesoderm slows down. The primitive streak diminishes in relative size and becomes an insignificant structure in the sacrococcygeal region of the embryo ( Fig. 4.6 D ). Normally the primitive streak undergoes degenerative changes and disappears by the end of the fourth week.
Remnants of the primitive streak may persist and give rise to a sacrococcygeal teratoma ( Fig. 4.7 ). A teratoma is a type of germ cell tumor that may be benign or malignant. Because they are derived from pluripotent primitive streak cells, the tumors contain tissues derived from all three germ layers in varying stages of differentiation. These teratomas are the most common tumor in neonates and have an incidence of approximately 1 in 35,000. Most affected infants (80%) are female. The teratomas are usually diagnosed on routine antenatal ultrasonography; most tumors are benign (nonmalignant). They are usually surgically excised promptly, with the prognosis dependent on many factors. A presacral teratoma may cause intestinal (bowel) or urinary obstruction, and surgical excision of such masses can have long-term sequelae in terms of the normal function of these same systems.


Notochordal Process and Notochord
4
Some mesenchymal cells migrate through the primitive streak and, as a consequence, acquire mesodermal cell fates. These cells migrate cranially from the primitive node and pit, forming a median cellular cord, the notochordal process . This process soon acquires a lumen, the notochordal canal ( Fig. 4.8 C to E ). The notochordal process grows cranially between the ectoderm and endoderm until it reaches the prechordal plate (see Fig. 4.8 A and C ), a small circular area of columnar endodermal cells where the ectoderm and endoderm are fused. The prechordal plate gives rise to the endoderm of the oropharyngeal membrane , located at the future site of the oral cavity ( Fig. 4.9 C ). The prechordal plate serves as a signaling center (Shh and PAX6) for controlling the development of cranial structures, including the forebrain and eyes.


Mesenchymal cells from the primitive streak and notochordal process migrate laterally and cranially, among other mesodermal cells between the ectoderm and endoderm, until they reach the margins of the embryonic disc. These cells are continuous with the extraembryonic mesoderm covering the amnion and umbilical vesicle (see Fig. 4.3 C and D ). Some mesenchymal cells from the primitive streak that have mesodermal fates migrate cranially on each side of the notochordal process and around the prechordal plate (see Fig. 4.5 A and C ). Here they meet cranially to form cardiogenic mesoderm in the cardiogenic area , where the heart primordium begins to develop at the end of the third week (see Figs. 4.8 B and 4.12 B ).
Caudal to the primitive streak there is a circular area, the cloacal membrane , which indicates the future site of the anus (see Fig. 4.8 E ). The embryonic disc remains bilaminar here and at the oropharyngeal membrane because the embryonic ectoderm and endoderm are fused at these sites, thereby preventing migration of mesenchymal cells between them (see Fig. 4.9 C ). By the middle of the third week, intraembryonic mesoderm separates the ectoderm and endoderm (see Fig. 4.9 D and G ) everywhere except:
- •
At the oropharyngeal membrane cranially (see Fig. 4.9 C )
- •
In the median plane cranial to the primitive node (see Fig. 4.5 A and B ), where the notochordal process is located (see Fig. 4.6 )
- •
At the cloacal membrane caudally (see Fig. 4.8 A and E )
Instructive signals from the primitive streak region induce notochordal precursor cells to form the notochord , a cellular rod-like structure (see Fig. 4.9 E ). The molecular mechanism that induces these cells involves (at least) Shh signaling from the floor plate of the neural tube.
The notochord:
- •
Defines the primordial longitudinal axis of the embryo and gives it some rigidity
- •
Provides signals that are necessary for the development of axial musculoskeletal structures and the central nervous system (CNS)
- •
Contributes to the intervertebral discs interposed between the bodies of adjacent vertebrae
Initially, the notochordal process elongates by invagination of cells from the primitive pit. The primitive pit, extends into the notochordal process, forming a notochordal canal (see Fig. 4.8 C ). The notochordal process now becomes a cellular tube that extends cranially from the primitive node to the prechordal plate (see Figs. 4.6 and 4.8 A to D ). Later, the floor of the notochordal process fuses with the underlying embryonic endoderm (see Fig. 4.8 E ). These fused layers gradually undergo degeneration, resulting in the formation of openings in the floor of the notochordal process, which brings the notochordal canal into communication with the umbilical vesicle (see Fig. 4.9 B ). As these openings become confluent, the floor of the notochordal canal disappears (see Fig. 4.9 C ), and the remains of the notochordal process form the flattened, grooved notochordal plate (see Fig. 4.9 D ). Beginning at the cranial end of the embryo, the notochordal plate cells proliferate and undergo infolding, which creates the notochord (see Fig. 4.9 F and G ). The proximal part of the notochordal canal persists temporarily as the neurenteric canal (see Fig. 4.9 C and E ), forming a transitory communication between the amniotic and umbilical vesicle cavities. When development of the notochord is complete, the neurenteric canal normally is obliterated .
The notochord becomes detached from the endoderm of the umbilical vesicle, the latter once again becoming a continuous layer (see Fig. 4.9 G ).
The notochord extends from the oropharyngeal membrane to the primitive node (see Fig. 4.6 B and D ). It degenerates as the bodies of the vertebrae form, but small portions of it persist as the nucleus pulposus of each intervertebral disc (see Chapter 14 ).
The notochord functions as the primary inductor (signaling center) in the early embryo. The developing notochord induces the overlying embryonic ectoderm to thicken and form the neural plate (see Fig. 4.9 C ), the primordium of the CNS.
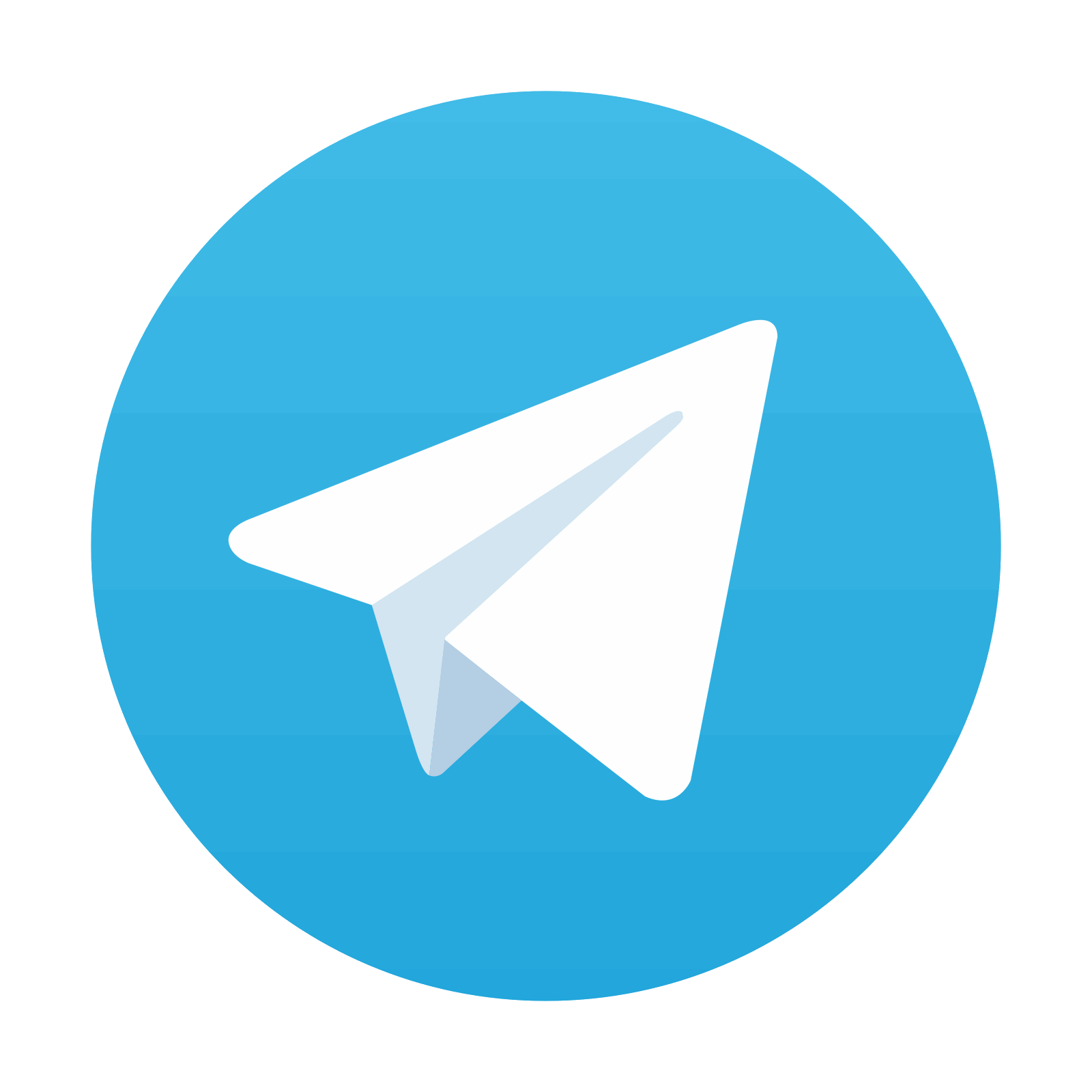
Stay updated, free articles. Join our Telegram channel

Full access? Get Clinical Tree
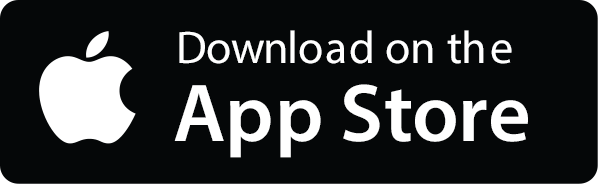
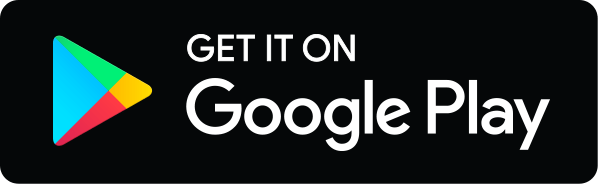
