Therapeutic Applications in Pediatric Intensive Care
Mary Lieh-Lai
Ashok Sarnaik
Introduction
The worst thing about medicine is that one kind makes another necessary.
–Elbert Hubbard
Management of a critically ill child poses a special challenge to the clinician. Most children admitted to an intensive care unit (ICU) have multisystem involvement. The clinician must not only be aware of age-related changes in pharmacokinetics and pharmacodynamics but also be cognizant of various drug–drug and drug–disease interactions. Because of the ongoing pathophysiologic changes, the physician must also deal with changes in volume of distribution and elimination kinetics during the clinical course. A critically ill child is often exposed to 20 or more medications during his or her hospital stay. Practically, every organ system and disease entity is represented in the pathophysiologic derangements of the patients in the ICU. A comprehensive discussion of management of an individual disease entity is beyond the scope of this chapter. Instead, we offer a working outline of management of most commonly encountered disease processes in critically ill children.
Central Nervous System
Increased intracranial pressure
Introduction
Management
Osmotherapy
Barbiturates
Hypertonic saline and glycerol
Acetazolamide and furosemide
Corticosteroids
Status epilepticus
Introduction
Management algorithm
Initial survey
The role of metabolic abnormalities and toxins in status epilepticus
Drug therapy of status epilepticus
Lorazepam
Midazolam
Diazepam
Phenytoin
Fosphenytoin
Phenobarbital
Levetiracetam
Pentobarbital
Isoflurane
Etomidate
Propofol
Paraldehyde
Myasthenia gravis
Introduction
Management
Edrophonium
Neostigmine
Corticosteroids
Intravenous immunoglobulin
Neuromuscular blocking agents
Introduction
Depolarizing neuromuscular blocking agent
Succinylcholine
Nondepolarizing or competitive neuromuscular blocking agents
Pancuronium
Vecuronium
Cisatracurium
Mivacurium
Rocuronium
Complications of neuromuscular blocking agents
Monitoring of neuromuscular blockade: train of four
Sedation
Introduction
Measures of sedation
Ramsay scale
COMFORT scale
Bispectral index (BIS)
Penn State Sedation Algorithm
Drugs used for sedation
Benzodiazepines
Propofol
Etomidate
Ketamine
Barbiturates
Dexmedetomidine
Complications of sedation
Pain and analgesia
Introduction
Measures of Pain
CHEOPS
Oucher scale
FLACC
Analgesics
Opioids
Nonsteroidal anti-inflammatory drugs (NSAIDs)
Syndrome of inappropriate antidiuretic hormone (SIADH) secretion
Definition
Management
Conivaptan
Central diabetes insipidus
Introduction
Management
Disorders of the Respiratory System
Status asthmaticus
Introduction
Management
β2-Adrenergic agents
Ipratropium bromide
Corticosteroids
Magnesium sulfate
Laryngotracheobronchitis and postextubation stridor
Introduction
Management
Dexamethasone
Budesonide
Pulmonary hypertension
Introduction and definitions
Management
Nitric oxide
Prostacyclin
Bosentan
Sildenafil
Acute Circulatory Failure
Introduction
Augmentation of preload
Crystalloids
Colloids
Reduction of preload
Furosemide
Bumetanide
Nesiritide
Enhancement of myocardial contractility
Sympathomimetics
Dopamine
Dobutamine
Epinephrine
Isoproterenol
Phosphodiesterase inhibitors
Milrinone
Afterload reduction
Nitroglycerin
Nitroprusside
Angiotensin-converting enzyme inhibitors
Miscellaneous agents
Prostaglandin E1
Vasopressin
β-Blockers: labetalol, esmolol, carvedilol
Fenoldopam
Anticoagulation and Thrombolytics
Introduction
Drugs used
Unfractionated heparin
Low-molecular-weight heparin
Oral anticoagulation
Recombinant tissue plasminogen activator
Monitoring of anticoagulation
Gastrointestinal Hemorrhage
Introduction
Management
Vasopressin
Octreotide
Central Nervous System Injury and Increased Intracranial Pressure
Management of intracranial hypertension is the cornerstone of modern neurointensive care. Primary brain injury results from a direct insult from the initial event such as trauma, infection, metabolic derangement, and ischemia. Secondary brain damage results from pathophysiologic alterations in response to the primary event. Increased intracranial pressure (ICP) is one of the most important factors which further aggravate the brain damage.
Osmotherapy
Osmotherapy takes advantage of the unique nature of the blood–brain barrier (BBB), which freely allows the transport of water across the capillary endothelium while remaining relatively impermeable in varying degrees to osmotic agents (1). Osmotherapy is most effective in situations where water accumulation is mainly intracellular and the BBB is well preserved. However, disruption of the BBB is neither absolute nor uniform even in conditions such as trauma and infections. Osmotherapy therefore is still effective to varying degrees in decreasing total brain water in such situations.
Mannitol
By far the most commonly used osmotic agent is mannitol (2). Intravenous administration of 0.25 to 0.5 g per kg of 20% mannitol solution reduces ICP within 30 minutes for periods up to 4 to 6 hours. Because the effectiveness of an osmotic agent depends upon the steepness of the osmolal gradient it creates, intermittent administration of a bolus of mannitol over 10 to 15 minutes is preferred to continuous infusion. The dose often needs to be repeated based upon clinical response and/or ICP measurement. In addition to its osmotic effects, mannitol may have other salutary effects on cerebral metabolism by its rheologic and free radical scavenging effects.
The aim of osmotherapy is to decrease brain bulk without causing total body dehydration and hypovolemia. It is important to monitor serum electrolytes, osmolality, and indicators of circulation. Mannitol is also associated with acute tubular necrosis. Inadequate renal function may result in hypervolemia and hyperosmolality that are poorly
tolerated by patients requiring osmotherapy. On occasion, mannitol can be combined with intravenous (IV) furosemide (0.5 to 1 mg per kg) to maximize its effects.
tolerated by patients requiring osmotherapy. On occasion, mannitol can be combined with intravenous (IV) furosemide (0.5 to 1 mg per kg) to maximize its effects.
Hypertonic Saline and Glycerol
Other osmotic agents such as glycerol and hypertonic saline have been used in brain-injured patients (3,4). Neither of these agents has been shown to be clearly superior to mannitol in randomized controlled trials. Hypertonic saline, however, has been shown to be safe and effective in controlling cerebral edema and seizures associated with severe symptomatic hyponatremia from SIADH and water intoxication. A small (3 to 4 mEq per L) but rapid rise in serum sodium is most effective for this purpose. With an apparent volume of distribution for sodium being 0.6 L per kg, an infusion of 4 to 5 mL per kg of 3% saline given over 10 to 15 minutes will raise serum sodium by approximately 3 to 4 mEq per L. This dose can be repeated two to three times as necessary (5). For hyponatremia associated with syndrome of inappropriate antidiuretic hormone, IV furosemide (1 mg per kg) given before hypertonic saline is effective in eliminating free water in addition to raising serum sodium.
Hypertonic saline has been recommended as an alternative to mannitol for the treatment of intracranial hypertension in brain-injured patients (6). Of the administered drug, mannitol is much more likely to be excreted compared with sodium. If a sustained increase in serum osmolality is desired, hypertonic saline is preferable. If the therapeutic aim is to expose the brain to elevated serum osmolality, mannitol is the preferred agent. There is no convincing evidence that hypertonic saline is superior to mannitol in this setting.
Barbiturates
High-dose barbiturate therapy is often used in conjunction with osmotherapy for management of intracranial hypertension associated with traumatic brain injury (2). Barbiturates are proposed to exert their beneficial effects by decreasing cerebral metabolic rate and cerebral vasoconstriction, decreasing lactate and excitatory amino acids, and inhibiting free radical mediated lipid peroxidation (7,8,9). Pentobarbital is by far the most commonly used agent for this purpose. We recommend using pentobarbital in a dose that best controls ICP. This can be accomplished by administering an IV bolus of 3 to 5 mg per kg followed by continuous infusion of 1 to 2 mg per kg per hour. Additional boluses or a higher infusion rate may be necessary. In general, serum levels of 30 to 40 μg per mL are required in most severely brain-injured patients. However, higher levels may be necessary. Barbiturate therapy is associated with significant hypotension and myocardial depression. Most patients require concomitant administration of inotropic agents such as dopamine and dobutamine for hemodynamic stability when high-dose barbiturate therapy is used.
Acetazolamide and Furosemide
A combination of acetazolamide and furosemide has been proposed to decrease ICP in pseudotumor cerebri (10). Both these drugs reduce cerebrospinal fluid production by inhibiting choroid plexus carbonic anhydrase. Furosemide decreases cerebrospinal fluid production by an additional unknown mechanism. Acetazolamide may be used singly or in combination with furosemide. Acetazolamide is administered orally or intravenously in a dose of 10 to 30 mg per kg given every 6 to 8 hours not to exceed 2 g per day. A randomized controlled trial did not show any benefit of acetazolamide–furosemide combination in posthemorrhagic hydrocephalus in infants (11).
Corticosteroids
There is no evidence that corticosteroids favorably impact the outcome in traumatic brain injury. However, high-dose steroid therapy has been shown to confer benefit in terms of improved function in traumatic spinal cord injury (12). For steroids to be effective, they should preferably be given within 8 hours of injury. If given within 3 hours of injury, the recommended dose of methylprednisolone is an IV bolus of 30 mg per kg followed within 1 hour by a continuous infusion of 5.4 mg per kg per hour for 23 hours. If given between 3 and 8 hours from injury, the IV bolus should be followed within 1 hour by a continuous infusion of 5.4 mg per kg per hour for 47 hours.
Status Epilepticus
Status epilepticus is defined as continuous or repeated convulsive or nonconvulsive seizures lasting 30 minutes or more. In children, causes include infections, trauma, toxic ingestions, and metabolic disturbances such as hyponatremia and hypocalcemia, brain tumors, epilepsy, and subtherapeutic levels of antiepileptic drugs. Status epilepticus is one of the most common disorders in children requiring ICU admission. Within the first 30 minutes of status epilepticus, sympathetic overactivity results in hypertension, tachycardia, and increased cardiac output. Increased catecholamine activity produces hyperglycemia. A combined respiratory and metabolic acidosis is a result of lactic acidosis and CO2 retention. Excessive muscle activity can lead to rhabdomyolysis and acute tubular necrosis. The increase in cerebral metabolism often outstrips cerebral blood flow and eventually this discrepancy leads to depletion of brain glucose and oxygen. Status epilepticus should be considered an emergency, and treatment and determination of the etiology should be instituted immediately (Fig. 23.1).
Initial Survey
Complications of status epilepticus may include vomiting and aspiration, apnea, and increased oxygen requirement. The initial assessment should ascertain that the airway is adequate. Supplemental oxygen should be started, and if there is any concern that airway and breathing are compromised, the patient should be intubated and mechanical ventilation started. Intravenous access is essential. If IV access cannot be established within the first 5 minutes, intraosseous access should be attempted, especially in children younger than 1 year. Blood pressure measurement is
important to determine the presence of hypertension as the cause of seizures. A lumbar puncture can be performed as long as the patient has a stable airway and hemodynamic status, and if there are no other contraindications such as an infection at the puncture site, coagulopathy, and increased ICP with evidence of focal neurologic findings. If imaging studies are needed, patients should first have their airway and hemodynamic status stabilized before studies such as computed tomography scans are obtained. Avoid the use of contrast material if renal failure is suspected.
important to determine the presence of hypertension as the cause of seizures. A lumbar puncture can be performed as long as the patient has a stable airway and hemodynamic status, and if there are no other contraindications such as an infection at the puncture site, coagulopathy, and increased ICP with evidence of focal neurologic findings. If imaging studies are needed, patients should first have their airway and hemodynamic status stabilized before studies such as computed tomography scans are obtained. Avoid the use of contrast material if renal failure is suspected.
Metabolic Abnormalities/Toxins
Hyponatremia resulting from excessive water supplementation or improper mixing of formula can be a common cause of seizures in infants and should be suspected in an infant presenting with status epilepticus who appears otherwise healthy. A proper history should be elicited immediately and once improper formula mixing or if excessive water administration is confirmed, 3% sodium chloride should be administered pending the results of serum electrolytes determination. Accidental ingestion of toxins should be suspected in toddlers, while intentional overdose should be suspected in older children and adolescents. In addition to removal of the toxin, an appropriate antidote should be administered if available.
Benzodiazepines
A group of drugs that bind to the benzodiazepine receptor on the neuronal #979;-aminobutyric acid (GABA) receptors, thereby facilitating the inhibitory action of GABA on neuronal transmission.
Lorazepam (Ativan) is the currently recommended first-line drug of choice for the treatment of status epilepticus because it can be injected rapidly and has a rapid onset of action of 2 to 3 minutes. Respiratory depression is observed in up to 9% of patients. Lorazepam is five times more potent than diazepam, is 90% protein bound, and has dependable absorption, with a serum half-life of up to 20 hours. It is metabolized by hepatic glucuronidation to inactive metabolites, and except in hepatic and renal failure, its metabolism is not significantly altered in critically ill children. Lorazepam is insoluble in water, needing the addition of polyethylene glycol as a solvent. With prolonged and high-dose infusions, lactic acidosis and hyperosmolar coma have been reported (13).
Midazolam (Versed) is an imidazobenzodiazepine that has been successfully used in the treatment of status epilepticus by both intravenous (14) and transmucosal (intranasal or buccal) route at a dose of 0.5 to 1 mg per kg (15,16,17,18). Baysun et al. determined that buccal midazolam was as effective as rectal diazepam in stopping seizures; and Harbord et al. found that seizures resolved in 89% of patients given intranasal midazolam. The use of continuous IV infusion
of midazolam at 1 to 5 μg per kg per minute has been used for the treatment of prolonged status epilepticus (19). The investigators found that the mean infusion rate required to control seizures was 2 μg per kg per minute. The onset of action is within 1 to 5 minutes, with a serum half-life of 1 to 2 hours. Up to 95% of the drug is protein bound. It is metabolized by hepatic microsomal oxidation, transforming it to active metabolites, α-hydroxymidazolam, and α-hydroxymidazolam-glucuronide. Drugs such as propofol and erythromycin can prolong the clearance of the active metabolites. This and other factors may prolong the effects of midazolam in critically ill children.
of midazolam at 1 to 5 μg per kg per minute has been used for the treatment of prolonged status epilepticus (19). The investigators found that the mean infusion rate required to control seizures was 2 μg per kg per minute. The onset of action is within 1 to 5 minutes, with a serum half-life of 1 to 2 hours. Up to 95% of the drug is protein bound. It is metabolized by hepatic microsomal oxidation, transforming it to active metabolites, α-hydroxymidazolam, and α-hydroxymidazolam-glucuronide. Drugs such as propofol and erythromycin can prolong the clearance of the active metabolites. This and other factors may prolong the effects of midazolam in critically ill children.
Diazepam (Valium) is highly lipophilic and is known to penetrate the BBB quickly. The onset of action is within 1 to 2 minutes, and the serum half-life as long as 60 hours. Although it has been replaced by lorazepam as the first-line drug of choice, 0.2 to 0.5 mg per kg rectal diazepam has its place in the treatment of status epilepticus in patients without IV access or as a drug that caregivers can use at home for children known to have frequent seizures.
Phenytoin: The solution for injection contains propylene glycol, ethanol, and sodium hydroxide resulting in an alkaline pH of about 12. When injected too rapidly, phenytoin has been known to precipitate cardiovascular collapse and arrhythmias. In addition, extravasation of phenytoin into the subcutaneous tissue causes tissue necrosis. Phenytoin follows both first-order and zero-order kinetics and is hydroxylated in the liver. The recommended bolus dose is 20 mg per kg, to be infused not more than 0.5 mg per kg per minute in children younger than 1 month, and not more than 1 mg per kg per minute in children older than 1 month. The onset of action is approximately 10 to 30 minutes, with a half-life of approximately 60 hours. Because of great variability in phenytoin metabolism, subsequent maintenance doses should be titrated to maintain free phenytoin serum levels at 1 to 2 μg per mL.
Fosphenytoin is a prodrug of phenytoin. Fosphenytoin sodium 1.5 mg is equivalent to 1 mg of phenytoin. Therapeutic concentrations can be achieved within 10 minutes following rapid IV injection and within 2 hours after intramuscular (IM) injection. Because fosphenytoin has a pH of 8.6 and is water soluble, it can be injected by IV injection more rapidly, and by IM injection when IV access cannot be obtained. However, in infants, there is extreme variability in therapeutic phenytoin levels (20) and, therefore, careful monitoring of serum levels is indicated.
Barbiturates
These drugs bind to the barbiturate receptor in the GABA receptor complex, producing sedation, hypnosis, anesthesia, and suppression of epileptiform activity. They are known to decrease cerebral metabolic oxygen demand and intracranial pressure. Adverse effects include suppression of myocardial contractility and peripheral vasodilation that can lead to hypotension. With prolonged infusion and use of high doses, patients often require inotropic drug support. With repeated doses, barbiturates can cause respiratory depression and apnea.
Phenobarbital is less lipophilic and does not enter the brain as rapidly. Onset of action can take as long as 60 minutes, and serum half-life as long as 3 days. A loading dose of 20 mg per kg is recommended, with a maintenance dose of 4 to 5 mg per kg per day. In the management of status epilepticus, serum levels should be monitored and maintained at 20 to 30 μg per mL. Higher therapeutic levels may be necessary for the treatment of prolonged status epilepticus resistant to lower levels and/or other antiepileptic drugs. Like lorazepam and thiopental, phenobarbital is formulated in propylene glycol.
Pentobarbital is formed by desulfurization of thiopental and has a short half-life. For the treatment of status epilepticus, a loading dose of 2 to 4 mg per kg can be administered. Because of a short half-life, a continuous infusion of 1 to 3 mg per kg per hour is often necessary. Some practitioners recommend electroencephalogram (EEG) monitoring, aiming for burst suppression. However, seizures have been known to continue despite burst suppression. All patients who require continuous pentobarbital therapy will need intubation and mechanical ventilation.
Isoflurane is a volatile anesthetic agent that is eliminated through the lungs. The difficulty in using this drug in the ICU is the lack of gas scavenging devices.
Etomidate is an anesthetic agent that has been used successfully in adults with status epilepticus (21). There are no studies evaluating the use of etomidate in children. The advantages of etomidate include its lack of myocardial depressant effects. However, it has been shown to result in significant adrenal suppression with only one dose (22); therefore, its long-term use requires supplementation with corticosteroids.
Propofol: Like etomidate, propofol is a short-acting intravenous anesthetic agent. It is formulated in an egg and soy emulsion and therefore should be avoided in children with egg or soy allergies. It has been found to be effective in stopping status epilepticus in adults but has not been studied in children. Long-term infusion has been reported to cause severe metabolic acidosis and the reports do support a cause and effect basis.
Paraldehyde is a cyclic polymer of acetaldehyde that is rapidly absorbed into the brain. It has been used successfully for over a century in the treatment of seizures. In the United States, however, paraldehyde USP is no longer available for human use. The manufacturers believe it is too expensive to make USP grade paraldehyde and with such limited use in humans, the decision was made to discontinue production. Paraldehyde is available in Canada and Europe.
Valproic acid by intravenous injection has been evaluated in adults with status epilepticus. Seizures were controlled in up to 75% of patients and no adverse cardiorespiratory effects were noted (23,24). However, intravenous valproic acid for the first-line treatment of status epilepticus has not been studied in children and its role is unclear at this time.
Levetiracetam is an anticonvulsant initially approved by the Food and Drug Administration (FDA) in oral form for adjunctive treatment of partial onset seizures in children aged 4 years or more, for myoclonic seizures in those older than 12 years, and for primary generalized tonic–clonic seizures. More recently, an intravenous form of the drug was approved for use as well. Levetiracetam has been shown to be efficacious, with a good safety profile. There are increasing reports that the intravenous administration of the drug may be effective in the treatment of status epilepticus in adults and children, with one report of
successful treatment of status epilepticus that was refractory to benzodiazepine (25,26,27). The mechanism of action of the drug is unclear but may be due to the prevention of hypersynchronization of epileptiform burst firing and propagation of seizure activity. Dosing regimen includes an intravenous loading dose of 15 mg per kg followed by 15 mg per kg per day divided in two doses. Others recommend no loading dose, with IV dose of 10 to 40 mg per kg per dose every 8 hours for infants and every 12 hours for older children. Approximately 66% of the drug is eliminated by the kidneys as unchanged drug. Therefore, dose adjustment is necessary with renal insufficiency.
successful treatment of status epilepticus that was refractory to benzodiazepine (25,26,27). The mechanism of action of the drug is unclear but may be due to the prevention of hypersynchronization of epileptiform burst firing and propagation of seizure activity. Dosing regimen includes an intravenous loading dose of 15 mg per kg followed by 15 mg per kg per day divided in two doses. Others recommend no loading dose, with IV dose of 10 to 40 mg per kg per dose every 8 hours for infants and every 12 hours for older children. Approximately 66% of the drug is eliminated by the kidneys as unchanged drug. Therefore, dose adjustment is necessary with renal insufficiency.
Myasthenia Gravis
Myasthenia gravis is an autoimmune disease caused by autoantibodies directed against the nicotinic receptors in the neuromuscular junction. Neonatal myasthenia gravis results from transplacental passage of maternal antibodies to the fetus. Medical therapy of myasthenia gravis consists of inhibition of acetyl cholinesterase inhibitors, immune suppression, and immune modulation. Acetyl cholinesterase inhibitors that are quaternary ammonium compounds (edrophonium, neostigmine, etc.) do not cross the BBB and are therefore appropriate for the management of myasthenia gravis. Tertiary amines such as physostigmine cross the BBB and are not appropriate in this setting.
Edrophonium is used mainly to establish the diagnosis but may also be used to distinguish between myasthenic crisis and cholinergic crisis in children receiving anticholinesterase therapy. An IV dose of 0.04 mg per kg over 1 minute is followed by 0.16 mg per kg (maximum 10 mg) given within 45 seconds if no response is observed. Objective evidence of motor function such as muscle strength and forced vital capacity should be documented. The onset of action is within seconds and duration of action is a few minutes. As with other anticholinesterase agents, severe bradycardia, hypotension, and other cholinergic effects can occur with the use of edrophonium. Atropine (0.01 mg per kg IV, minimum 0.1 mg, maximum 2 mg) should be available at the patient’s bedside to counteract serious cholinergic effects if they should occur.
Neostigmine is used both as a diagnostic agent as well as for ongoing therapy. It can be given IM in a dose of 0.025 to 0.04 mg per kg as a diagnostic tool. For ongoing therapy, 0.04 mg per kg can be given IM every 3 to 4 hours. Neostigmine bromide given orally in a dose of 0.4 mg per kg every 4 to 6 hours is preferred for prolonged use. For patients with significant dysphagia, neostigmine should be administered about 30 minutes before meals. Pyridostigmine is slightly longer acting than neostigmine. It is administered orally in a dose of 5 mg per kg per day in 4 to 6 divided doses. The doses of both neostigmine and pyridostigmine may have to be adjusted every 48 hours.
Corticosteroids: Long-term therapy may be necessary for immune suppression. A single oral daily dose of prednisone (0.5 to 1 mg per kg per day) is recommended. In resistant cases, combined steroid and azathioprine therapy is utilized to minimize the side effects of steroids. Thymectomy should be considered for patients with high titers of antiacetylcholine-receptor antibodies.
Intravenous immunoglobulin (IVIG): For immune modulation, plasmapheresis and IVIG are sometimes beneficial. IVIG is administered as 2 g per kg as a single dose over 10 to 12 hours or 400 mg per kg daily for 4 days. Relative benefits of immune modulation are still unconfirmed by randomized controlled studies. Several case series report short-term benefit from plasmapheresis particularly in myasthenic crisis (28).
Neuromuscular Blocking Agents
In addition to sedative agents, critically ill children may require neuromuscular blocking agents (NMBAs) to facilitate various mechanical ventilatory modes such as high frequency oscillation, inverse ratio ventilation, and slow rates, all of which can induce significant discomfort and agitation. The addition of neuromuscular blockade may help improve respiratory system compliance and reduce barotrauma by preventing the patient from “fighting” the ventilator. Occasionally, neuromuscular blockade may also be necessary in children following laryngotracheoplasty, or those with extensive facial injuries or following facial reconstructive surgery where movement has to be kept at a minimum to maintain the artificial airway. NMBAs exert either depolarizing or nondepolarizing (competitive) effects on the neuromuscular junction.
Succinylcholine acts similarly to acetylcholine and induces membrane depolarization. Because it is resistant to degradation by acetylcholinesterase, administration of succinylcholine produces initial fasciculations resulting from repetitive excitation, followed by flaccid paralysis. The recommended dose in children is 1 mg per kg per dose. Paralysis occurs in 1 minute after IV injection. Effects dissipate quickly as the drug is metabolized by butyrylcholinesterase in the blood and liver. However, during depolarization induced by succinylcholine, significant amounts of potassium are released, and succinylcholine is therefore contraindicated in conditions that can potentiate hyperkalemia. These include crush injuries, burns, and rhabdomyolysis. It is also contraindicated in patients with ICP, spinal cord injuries, muscular dystrophies, and known or family history of malignant hyperthermia.
Nondepolarizing or competitive NMBAs include pancuronium, vecuronium, atracurium, and mivacurium. These drugs block acetylcholine from binding to receptors on the motor endplate, thereby inhibiting depolarization. Elimination of nondepolarizing NMBAs varies, and this characteristic should be taken into account when using these drugs in critically ill children (Table 23.1).
In using NMBAs, a number of potential complications should be taken into account. It is important to ascertain adequacy of sedation to prevent the frightening consequence of paralyzing a child who has total awareness. The paralysis induced by agents with steroid rings such as pancuronium and vecuronium can result in a myopathy and prolonged paralysis when the patient is on corticosteroids as well (29). There are a number of drugs frequently used in children in the ICU that can potentiate the effects of NMBAs resulting in prolonged paralysis. These include antibiotics such as aminoglycosides; antiarrhythmics such as
β-adrenergic blockers; and other drugs such as furosemide, magnesium, and cyclosporine. Abnormalities in renal function and electrolyte disorders such as hypocalcemia and treatment modalities such as hypothermia can all prolong neuromuscular blockade and weakness. Unlike vecuronium or rocuronium, 80% of cisatracurium is metabolized to laudanosine via Hoffman elimination, with less than 20% of unchanged drug excreted in the kidneys, making it the more appropriate NMBA to use in patients with renal insufficiency. In addition, a study comparing vecuronium and cisatracurium in infants after congenital heart surgery showed more rapid spontaneous recovery of neuromuscular function with cisatracurium (30). Occasionally, there may be a need for continuous or frequent neuromuscular blockade. In such patients, the depth of neuromuscular blockade should be monitored. Clinical parameters such as handgrip strength may be used but may not be applicable in infants or those children who are sedated. Stimulating the ulnar nerve and assessing the evoked response of the adductor pollicis can provide a more objective measurement. Monitoring of neuromuscular function with the train-of-four ratio is useful in judging the response to therapy. Train of four is a modality whereby peripheral motor nerve stimulation is applied followed by the observation of muscle movement in response to the stimuli. Nondepolarizing agents such as vecuronium decrease the size of single twitches and both the strength and duration of the response to repetitive stimulation. The train of four consists of four stimuli given at 2 per second and is commonly used to determine the degree of neuromuscular blockade and the number of receptors occupied. If three twitches are observed, approximately 75% to 80% of receptors are occupied; and if one twitch is observed, 90% to 95% of receptors are occupied. One to two twitches indicate approximately 75% to 90% receptor occupancy and are thought to provide the optimum level of neuromuscular relaxation. Monitoring of the train of four in patients who are receiving frequent or continuous dosing of NMBAs helps prevent overdosing and prolonged paralysis.
β-adrenergic blockers; and other drugs such as furosemide, magnesium, and cyclosporine. Abnormalities in renal function and electrolyte disorders such as hypocalcemia and treatment modalities such as hypothermia can all prolong neuromuscular blockade and weakness. Unlike vecuronium or rocuronium, 80% of cisatracurium is metabolized to laudanosine via Hoffman elimination, with less than 20% of unchanged drug excreted in the kidneys, making it the more appropriate NMBA to use in patients with renal insufficiency. In addition, a study comparing vecuronium and cisatracurium in infants after congenital heart surgery showed more rapid spontaneous recovery of neuromuscular function with cisatracurium (30). Occasionally, there may be a need for continuous or frequent neuromuscular blockade. In such patients, the depth of neuromuscular blockade should be monitored. Clinical parameters such as handgrip strength may be used but may not be applicable in infants or those children who are sedated. Stimulating the ulnar nerve and assessing the evoked response of the adductor pollicis can provide a more objective measurement. Monitoring of neuromuscular function with the train-of-four ratio is useful in judging the response to therapy. Train of four is a modality whereby peripheral motor nerve stimulation is applied followed by the observation of muscle movement in response to the stimuli. Nondepolarizing agents such as vecuronium decrease the size of single twitches and both the strength and duration of the response to repetitive stimulation. The train of four consists of four stimuli given at 2 per second and is commonly used to determine the degree of neuromuscular blockade and the number of receptors occupied. If three twitches are observed, approximately 75% to 80% of receptors are occupied; and if one twitch is observed, 90% to 95% of receptors are occupied. One to two twitches indicate approximately 75% to 90% receptor occupancy and are thought to provide the optimum level of neuromuscular relaxation. Monitoring of the train of four in patients who are receiving frequent or continuous dosing of NMBAs helps prevent overdosing and prolonged paralysis.
Table 23.1 Nondepolarizing Blocking Agents | ||||||||||||||||||||||||||||
---|---|---|---|---|---|---|---|---|---|---|---|---|---|---|---|---|---|---|---|---|---|---|---|---|---|---|---|---|
|
The injection of aminosteroidal nondepolarizing NMBAs such as rocuronium and vecuronium stimulates the C-nociceptors in the peripheral veins, causing severe pain (31,32). The pain, as indicated by localized withdrawal response, is significant even after loss of consciousness during anesthesia induction. Ahmad et al. assessed the efficacy of fentanyl or lidocaine in the prevention of rocuronium injection–associated pain and found that fentanyl was more effective than lidocaine (33).
Rapid reversal of neuromuscular blockade is sometimes necessary in ICU patients who have received nondepolarizing agents. Neostigmine, 0.05 to 0.07 mg per kg IV (maximum of 5 mg), can be used for this purpose and has a peak action within 5 to 8 minutes. Atropine may have to be administered to counteract excessive muscarinic effects of bradycardia, increased secretions, and bronchospasm.
Sugammadex is a drug-specific modified #979;-cyclodextrin that is a selective relaxant binding agent. Unlike neostigmine, which increases cholinergic activity, the selective relaxant binding agent rapidly encapsulates steroidal NMBAs such as rocuronium, thereby directly preventing the pharmacologic actions of NMBAs. Studies have shown that sugammadex is effective and safe (34). The drug has been approved for use in Europe but not in the United States.
Sedation
Sedation is often necessary in critically ill children because of the need to maintain endotracheal tubes, facilitate mechanical ventilation, and to ensure that chest tubes, central venous, arterial, and bladder catheters are not pulled out by an agitated child. The ICU environment is often a stressful one. Endotracheal tubes prevent children from crying or communicating. Frequent monitor and ventilator alarms and harsh lighting prevent normal sleep. Pain from catheters and procedures may add to the discomfort. However, despite the routine use of sedative agents in ICUs, there are few sedation scales that are designed for, and validated for use in children. A number of scales have been designed to assess sedation, including the Ramsay scale, the Comfort scale, Children’s Hospital of Eastern Ontario Pain Scale (CHEOPS), visual analogue scale, and the Riker sedation–agitation scale. More commonly, caregivers use physiologic parameters such as heart rate and blood pressure, or movement to determine a child’s need for sedation. Children therefore can be subject to both undersedation and oversedation. The complications of oversedation include hemodynamic instability, severe withdrawal syndromes, and prolongation of mechanical ventilation and ICU stay.
The Ramsay Scale (35)
Awake Levels
Level 1: Patient anxious and agitated, restless, or both.
Level 2: Patient cooperative, oriented, and tranquil.
Asleep Levels
Level 3: Patient awake responds to commands only.
Level 4: Patient asleep, brisk response to a light glabellar tap or loud auditory stimulus.
Level 5: Sluggish response to a light glabellar tap or loud auditory stimulus.
Level 6: No response to a light glabellar tap or loud auditory stimulus.
Shortcomings of the Ramsay scale include overlap between the levels; each level is not described clearly and the scale has not been validated. There are difficulties in using this scale in children at the stage of development where they cannot understand commands or cooperate.
The Comfort Scale (36)
This scale was specifically designed for intubated children in the ICU and scores eight physiologic and behavioral parameters from 1 to 5, with a total range of 8 to 40. The optimal sedation range is 17 to 26. The COMFORT scale (Table 23.2) has advantages over all other scales because of its applicability to children of all ages and developmental status, and it does not require repeatedly awakening a patient to assess the adequacy of sedation.
Table 23.2 The COMFORT Scale | ||||||||||||||||||||||||||||||||||||||||||||||||||||||||||||||||||||||||||||||||||||||||||||||||||||||||
---|---|---|---|---|---|---|---|---|---|---|---|---|---|---|---|---|---|---|---|---|---|---|---|---|---|---|---|---|---|---|---|---|---|---|---|---|---|---|---|---|---|---|---|---|---|---|---|---|---|---|---|---|---|---|---|---|---|---|---|---|---|---|---|---|---|---|---|---|---|---|---|---|---|---|---|---|---|---|---|---|---|---|---|---|---|---|---|---|---|---|---|---|---|---|---|---|---|---|---|---|---|---|---|---|
|
The Penn State Children’s Hospital Sedation Algorithm (37)
Popernack et al. instituted the Penn State Children’s Hospital Algorithm to provide goal-directed sedation for mechanically ventilated children. Physicians write the order for the goal sedation level along with the appropriate medications that allow the nursing staff autonomy to provide individualized patient sedation regimens. Following implementation of the algorithm, the accidental extubation rates decreased significantly without increasing length of stay (37).
The Penn State Children’s Hospital Sedation Algorithm for ventilated children:
Level 1
Goal: Awake and interactive with environment, that is, watches television, communicates (generally for more mature children with neuromuscular cause for assisted ventilation).
Action: PRN anxiolytics/analgesics.
Level 2
Goal: Sleepy, arouses to light stimulation, becomes excited with nursing care/suctioning, moves spontaneously, turns head, consistently breathes above the ventilator.
Action: PRN anxiolytics/analgesics, with or without continuous anxiolytics/analgesics; paralytics only if PRN sedatives fail.
Level 3
Goal: Asleep most of the time, arouses to pain, coughs with suctioning, breathes above ventilator, little spontaneous movement or head turning.
Action: PRN anxiolytics/analgesics with or without continuous anxiolytics/analgesics; paralytics only if PRN sedation fails.
Level 4
Goal: Asleep, arouses to pain, coughs with suctioning, returns to sleep immediately, does not consistently breathe above ventilator, little spontaneous movement, no head turning.
Action: Continuous anxiolytics/analgesics, PRN anxiolytics/analgesics; paralytics only if PRN sedatives fail.
Level 5
Goal: Asleep, minimal response to pain or suctioning, no respiratory effort, no sustained spontaneous movements.
Action: Continuous anxiolytics/analgesics; liberal use of paralytics if PRN sedatives fail.
Level 6
Goal: Asleep, continuous paralysis, level of paralysis assessed by nerve stimulator or by observing minor movements between supplemental doses.
Action: Continuous anxiolytics/analgesics, continuous paralytics; PRN anxiolytics/analgesics titrated to vital signs. Utilize train-of-four nerve stimulator for serial assessments, or observe motor movements between supplemental doses.
Protocol for using the levels of sedation algorithm:
After intubation, the level is established by the team to create an individualized patient behavior goal. The level is written as a physician order.
With appropriate medications prescribed, the nurse uses clinical assessment skills to administer pharmacological and age-appropriate psychological support to achieve the established goal.
The staff explains the desired level and the plans for implementation to the patient’s family. Nonverbal communication is used for levels 1 through 3 based on the patient’s cognition and age.
Respiratory therapists are responsible to help identify changes in patient status that deviate from the set goal.
An evaluation and adjustments are made daily and when dynamic changes occur.
Documentation:
Physician order on the plan of care.
Medications administered.
Patient data indicating therapeutic efficacy or required adjustments.
Assessment in the progress notes (37).
The BIS Monitor (Aspect Medical Systems, Natick, MA): BIS is a processed EEG variable obtained by computing EEG signals through Fast Fourier transformation, bispectral analysis, and artifact filtering and suppression detection, resulting in a single number—the BIS. BIS allows monitoring of depth of anesthesia or sedation, ranging from 100 (awake) to 0 (isoelectric EEG). Its use has been validated in children (38,39). Crain et al. compared the BIS score to the COMFORT scale and found that BIS may be better than the COMFORT scale in identifying and preventing oversedation of children in the pediatric ICU (PICU) (39). Furthermore, Lamas et al. compared the BIS, middle latency auditory evoked potentials and the modified Ramsay scale, and the Comfort scale to detect the responsiveness of 86 children to painful stimuli and determined that the BIS provided the most sensitive method of assessing responses (40). More importantly, these investigators determined that hemodynamic variables such as heart rate and blood pressure did not change after the application of the stimulus. This confirms the findings of other studies that using such clinical parameters to determine the occurrence of pain is of little use (41,42).
Benzodiazepines
Benzodiazepines offer anxiolysis, hypnosis, and amnesia, characteristics which are desirable in many ICU patients. Midazolam and lorazepam are the most commonly used benzodiazepines. The ideal amount of sedation is that which makes the patient sleepy and quiet but arousable and able to follow commands. More frequently, however, deeper sedation is needed, especially in those children on high-frequency oscillation, those with intracranial hypertension, those who have undergone surgical procedures where minimal movement is required for healing, and those requiring frequent neuromuscular blockade. Benzodiazepines exert their action by binding to GABA receptors. The binding results in increased cellular chloride entry inducing hyperpolarization of neuronal cells, rendering them resistant to excitation.
Lorazepam penetrates the BBB more slowly than other benzodiazepines such as diazepam. This characteristic results in a longer duration of amnestic effect. It is metabolized by hepatic glucuronidation into harmless metabolites and is therefore void of drug interactions, making it an ideal drug to use in the PICU. Lorazepam can be given by continuous infusion (0.06 mg per kg per hour) or intermittent injection (0.1 mg per kg per dose). It is also available in oral form. Lorazepam is the sedative agent most frequently used in both adult and pediatric ICUs.
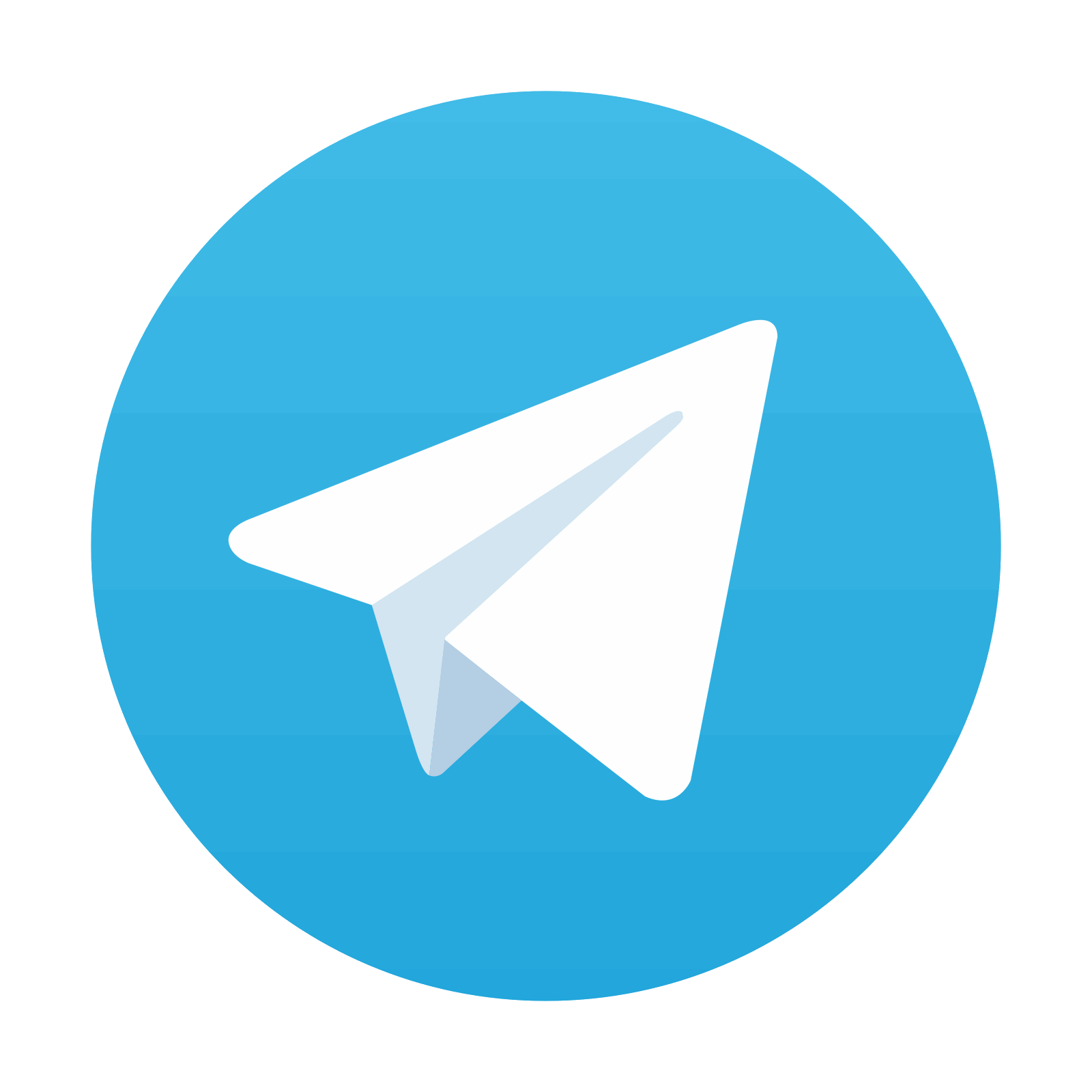
Stay updated, free articles. Join our Telegram channel

Full access? Get Clinical Tree
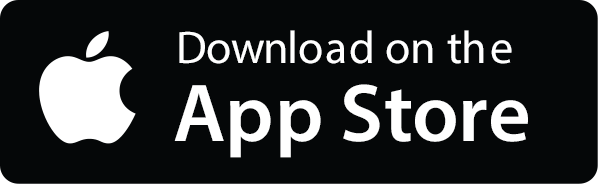
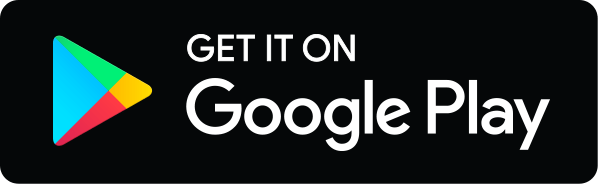