Preeclampsia is a syndrome occurring only in pregnancy characterized by systemic maternal inflammation and associated with the presence of the placenta. How these 2 aspects of the disease are linked has been the subject of numerous theories and ideas. Recently, there has been increasing interest in DNA shed from the placenta into the maternal circulation as a potential agent initiating the inflammatory response. This review will discuss the current evidence and future directions for placental DNA as the linking factor in preeclampsia in the context of other hypotheses.
Preeclampsia is a syndrome occurring only in human pregnancy characterized clinically by persistent maternal hypertension (systolic blood pressure >140 mm Hg or diastolic >90 mm Hg) accompanied by ≥1 of a range of other symptoms and/or signs including severe proteinuria, decreased platelets, or decreased kidney or liver function. Preeclampsia causes considerable mortality and morbidity worldwide, affecting 2-8% of pregnancies and contributes, along with other “hypertensive disorders of pregnancy” (these include hypertension but no proteinuria and women with preceding chronic hypertension), to at least a quarter of maternal deaths. In addition, preeclampsia is associated with considerable fetal and neonatal mortality due to preterm birth and fetal growth restriction.
Despite the importance of this disease, the pathogenesis of preeclampsia is still somewhat mysterious. An important model postulates that preeclampsia should be considered as a 2-stage disorder. The first stage is reduced placental perfusion and the second stage the maternal systemic inflammatory syndrome, where endothelial cell decompensation is particularly important in triggering maternal hypertension. An extension of this model now includes a “stage 0”–the initial problem with placentation, the root of the majority of cases of preeclampsia–and a second “stage 2,” describing the effects on the fetus ( Figure 1 ). Although it may require some adaptation, this model is supported by many of the key observations in preeclampsia research, summarized in Table 1 . One of the challenges in understanding preeclampsia is explaining the link between a local effect generated at the level of the stressed placenta and the systemic maternal syndrome. The various hypotheses proposed are summarized in Table 2 . The focus of this review is on DNA shed from the placenta into the maternal circulation.

Aspect of model | Supporting evidence | Reference |
---|---|---|
Placenta is key initiator | Fetus and uterus are unnecessary–preeclampsia can occur in hydatidiform mole and abdominal pregnancy | |
Delivery of the placenta is the only effective treatment | ||
Role of placental hypoperfusion | Indirect measurements of uteroplacental blood flow | |
Doppler ultrasound studies | ||
Systemic inflammatory nature of maternal syndrome | Multiple measures including platelet aggregation, cytokine levels, endothelial activation | |
Effects on fetus | Multiple measures including birthweight, survival |
Hypothesis for linking factor | Summary of evidence | References |
---|---|---|
Direct products of placenta | ||
Cytokines |
|
|
s-Flt and other angiogenesis-regulating factors |
|
|
Leptin |
|
|
Direct effects of hypoxia | ||
Effects of oxidative stress on cells passing through placenta and release of metabolites such as uric acid |
|
|
Release of placental debris | ||
Syncytiotrophoblast microparticles |
|
|
Placental DNA |
|
Circumstantial evidence for placental DNA as the linking factor
Three pieces of circumstantial evidence suggest placental DNA could be one of the inflammatory triggers in preeclampsia:
- (1)
DNA itself is an immunostimulatory molecule when present outside the nucleus or mitochondria.
- (2)
In preeclamptic pregnancies the quantity of free DNA shed into the maternal circulation by the placenta is greatly increased.
- (3)
Placental DNA derived from syncytiotrophoblast may be in a more immunostimulatory state than from somatic cells.
Immune sensors for free DNA
The immunostimulatory properties of free DNA have been known for >50 years. Although DNA in the nucleus of cells is generally considered to be safe from detection, cytoplasmic or extracellular DNA can act as a pathogen or danger associated molecular pattern (PAMP or DAMP). PAMPs activate pattern recognition receptors (PRRs) of the innate immune system and initiate immune responses. There are many PRRs including Toll-like receptor 9 (TLR9), which senses unmethylated Cytosine-phosphate-Guanine (CpG) islands in endosomes, and various sensors of double-stranded DNA in the cytoplasm ( Figure 2 ).

TLR9 is preferentially expressed in plasmacytoid dendritic cells, traffics to endosomes and becomes mature following cleavage of its exodomain. Though much DNA binds TLR9 with little specificity, only unmethylated CpG DNA is able to cause a conformational change in TLR9 homodimers resulting in the close apposition of the TIR signaling domains and downstream activation of interferon regulatory factors (IRFs) and expression of inflammatory cytokines. Although initially discovered because of its ability to detect bacterial DNA, subsequent studies found TLR9 also detects self-DNA when present in endosomes in sufficient quantity. This raises the possibility of TLR9 stimulation by placental DNA, particularly because of the unique characteristics of DNA derived from trophoblast cells (see below).
The cytoplasmic DNA sensors belong to a broad range of protein families. Earlier discoveries focused on the PYHIN family, a group of proteins that contain DNA-binding HIN domains as well as signaling PYR domains. The PRR absent in melanoma protein (AIM) 2 that belongs to this class is the founding member of a group of AIM2-like receptors, including the PRR IFI16. AIM2 signals by assembling ASC and caspase-1 containing inflammasomes, thus resulting in the production of the inflammatory cytokine interleukin (IL)-1β whereas IFI16 activates IRFs and nuclear factor kappa-light-chain-enhancer of activated B cells (NF-κB) via stimulator of interferon genes (STING) (see below) inducing the production of a broad range of inflammatory mediators. Another group of DNA sensors are the DExD/H-box helicases, including RIG-I and DDX41. Counterintuitively, RIG-I actually directly detects RNA, but can also indirectly detect DNA through the converting action of cytoplasmic RNA polymerase III. Further STING-activating DNA sensors such as DNA-dependant protein kinase, cyclic GMP-AMP synthetase, and meiotic recombination 1 also exist in the cytoplasm. A common question is how the detection of DNA by these factors signals to produce transcriptional changes within a cell. One protein of importance is STING, which forms a common adaptor in many of these signaling pathways. STING is an endoplasmic reticulum-bound protein with a cytoplasmic C-terminal domain that upon activation by upstream elements forms a scaffold for assembly of the kinase TANK-binding kinase 1 and transcription factor IRF3, allowing phosphorylation of TANK-binding kinase 1 and IRF3 activation. This process occurs simultaneously with the relocation of STING from the endoplasmic reticulum to mysterious punctate foci closer to the cell membrane. How exactly STING becomes active remains an area of intensive research, but because STING is responsive to the signaling molecule cyclic guanosine monophosphate-adenosine monophosphate, formed by the DNA-sensing enzyme cyclic GMP-AMP synthetase, other DNA-sensing proteins may affect STING through alterations in the level of this second messenger.
Although DNA sensing has been shown to be essential in our defense against certain pathogens such as herpesviruses, it has also been linked to many autoimmune and autoinflammatory conditions, including systemic lupus erythematosus (SLE), Aicardi-Goutiere syndrome, and chronic heart failure. In these conditions, sterile inflammation often associated with genetic variations in DNA disposal enzymes such as the lysosomal deoxyribonuclease II and cytoplasmic TREX1 can drive pathology. Indeed, loss of TREX1 in mice is sufficient to cause in utero death from DNA-driven inflammation that depends on the cytoplasmic detection of DNA as shown by rescue upon STING knockout. Thus, there are precedents for the involvement of fetal DNA as the factor driving inflammation in preeclampsia.
Shedding of placental DNA in pregnancy
Fetal DNA was first detected in maternal plasma in 1997. This DNA originates from the placenta, and placental-specific messenger RNA molecules are also easily detected in maternal plasma. The source of the placental DNA is mainly from the syncytiotrophoblast covering the villous tree that is in contact with the maternal blood in the intervillous space. Fetal DNA in maternal plasma is detectable from the seventh week of gestation onwards and can reach high concentrations: 25.4 and 292.2 genome equivalents/mL in early and late pregnancy, respectively. This accounts for around 3.5% and 6.2% of the DNA present in maternal plasma in the 2 stages of pregnancy.
Importantly, in preeclampsia, fetal DNA reaches concentrations up to 5-fold higher than this and can be detected in early pregnancy before the onset of preeclampsia. There is also a graded response between the quantity of fetal DNA and the risk of developing preeclampsia, with levels highest in women with HELLP (hemolysis, elevated liver enzymes, and low platelet count) syndrome ( Figure 3 ).

The increase in shed DNA in preeclampsia reflects the increased cell death due to hypoxia. Levels of DNA released into supernatants from placental explants are increased when exposed to reduced oxygenation. Thus, the magnitude of the placental stress (stage 1 of preeclampsia) can be correlated with the levels of DNA (the putative linking factor) and hence to initiation of the systemic inflammatory syndrome (stage 2). Moreover, although fetal DNA in women with preeclampsia is increased compared to controls as early as 17 weeks of gestation, there is a sharp rise 3 weeks before the appearance of signs of preeclampsia, also suggesting a role for DNA in the precipitation of the systemic syndrome ( Figure 4 ). However, 1 study found that increases in plasma C-reative protein levels did not mirror the increases in fetal DNA, arguing against a direct link between DNA levels and systemic inflammation. Nonetheless, these studies do provide circumstantial evidence that increased circulating fetal (trophoblast) DNA may play a role in driving the systemic symptoms and signs of preeclampsia.

Characteristics of shed placental DNA
As well as being shed in large quantities from preeclamptic placentas, one characteristic of fetal–and particularly trophoblast–DNA is that it is hypomethylated, related to epigenetic states important for early development. This distinct methylation pattern is present throughout gestation, though it may be influenced by fetal sex. Hypomethylation at CpG motifs could make trophoblast DNA a ligand for TLR9, increasing its immunostimulatory ability in preeclampsia. Furthermore, trophoblast DNA is hypomethylated to a greater extent in the preeclamptic placenta compared to controls, and to a greater extent in early- compared to late-onset disease. Indeed, methylome profiling of placental DNA in maternal blood has been proposed as a way of assessing the risk of early- or late-onset preeclampsia. This is attributed to a secondary effect of hypoxia altering gene expression. Increased hypomethylation characteristic of trophoblast could also have a primary role in the pathogenesis of preeclampsia through increased activation of innate DNA sensors.
Direct evidence for placental DNA as the linking factor
In addition to the above circumstantial evidence, placental DNA can act as an inflammatory agent with associated pregnancy disruption in mice. Human fetal DNA triggers in vitro activation of NF-κB (a transcription factor with a major role in the inflammatory response) with resultant IL-6 production in both a human B-cell line and peripheral blood mononuclear cells from both pregnant and nonpregnant donors. Injection of human fetal (but not adult) DNA into pregnant BALB/c mice causes fetal resorption with increased levels of tumor necrosis factor-α and IL-6 and infiltration by inflammatory cells in the placental bed. Fetal but not adult DNA is also susceptible to cleavage by HPAII, an enzyme that cleaves DNA at unmethylated CpG islands. Deletion or inhibition of TLR9 in pregnant mice blocked fetal loss and the accompanying inflammation. Thus, fetal DNA can initiate inflammation through TLR9 activation and this affects pregnancy outcome in mice, supporting the hypothesis that fetal DNA may contribute to inflammation in preeclampsia. This model is not without its problems however. Although injection of control CpG DNA into pregnant mice induced systemic production of a range of inflammatory cytokines (interferon-γ, IL-12p70, tumor necrosis factor-α, IL-10), histological evidence of inflammation was restricted to the placenta and endometrium. This argues against placental DNA as a creator of systemic inflammation. The fetal DNA in this study was derived from umbilical cord tissue (Dr Sinéad Corr, PhD, written personal communication received March 19, 2014), and not from trophoblast so is not representative of the placental DNA liberated into maternal blood. Moreover, mice do not naturally experience preeclampsia, limiting their usefulness as a representative model.
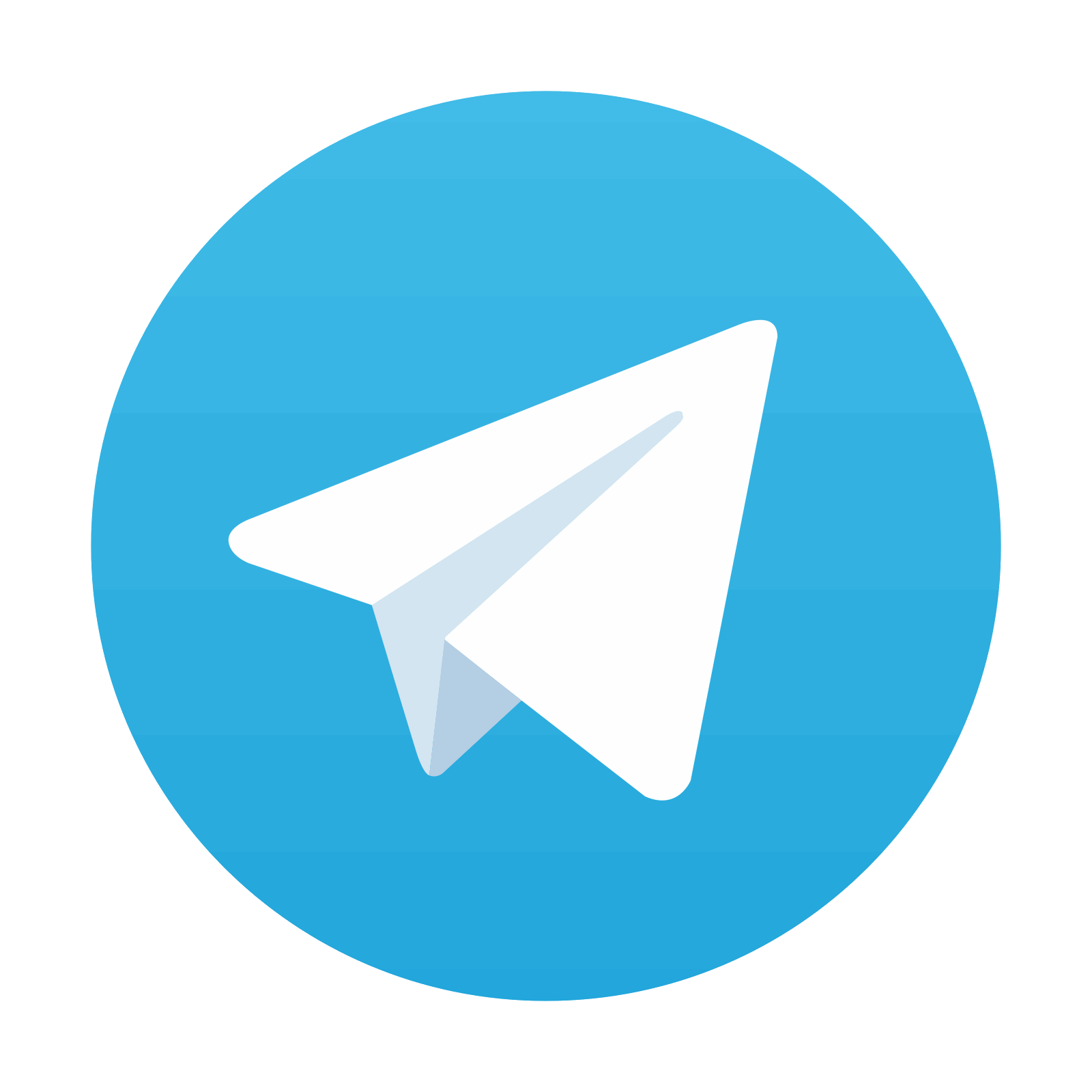
Stay updated, free articles. Join our Telegram channel

Full access? Get Clinical Tree
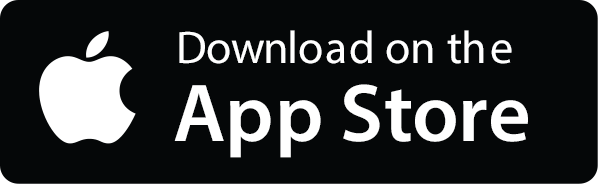
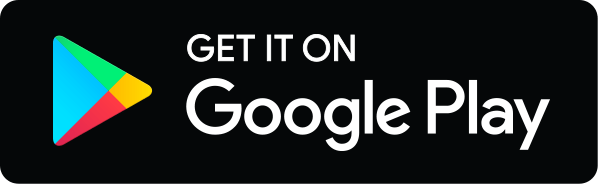