INVOLUTION OF THE REPRODUCTIVE TRACT
Birth Canal
Return to the nonpregnant state begins soon after delivery. The vagina and its outlet gradually diminish in size but rarely regain their nulliparous dimensions. Rugae begin to reappear by the third week but are less prominent than before. The hymen is represented by several small tags of tissue, which scar to form the myrtiform caruncles. Vaginal epithelium begins to proliferate by 4 to 6 weeks, usually coincidental with resumed ovarian estrogen production. Lacerations or stretching of the perineum during delivery may result in vaginal outlet relaxation. Some damage to the pelvic floor may be inevitable, and parturition predisposes to urinary incontinence and pelvic organ prolapse. This is discussed in detail in Chapter 27 (p. 536).
Uterus
The massively increased uterine blood flow necessary to maintain pregnancy is made possible by significant hypertrophy and remodeling of pelvic vessels. After delivery, their caliber gradually diminishes to approximately that of of the prepregnant state. Within the puerperal uterus, larger blood vessels become obliterated by hyaline changes, are gradually resorbed, and are replaced by smaller ones. Minor vestiges of the larger vessels, however, may persist for years.
During labor, the margin of the dilated cervix, which corresponds to the external os, may be lacerated. The cervical opening contracts slowly and for a few days immediately after labor, readily admits two fingers. By the end of the first week, this opening narrows, the cervix thickens, and the endocervical canal reforms. The external os does not completely resume its pregravid appearance. It remains somewhat wider, and typically, ectocervical depressions at the site of lacerations become permanent. These changes are characteristic of a parous cervix (Fig. 36-1). The markedly attenuated lower uterine segment contracts and retracts, but not as forcefully as the uterine corpus. During the next few weeks, the lower segment is converted from a clearly distinct substructure large enough to accommodate the fetal head to a barely discernible uterine isthmus located between the corpus and internal os.
Cervical epithelium also undergoes considerable remodeling, and this actually may be salutary. Ahdoot and associates (1998) found that approximately half of women showed regression of high-grade dysplasia following vaginal delivery. Kaneshiro and coworkers (2005) found similar regression—about 60 percent overall—regardless of delivery mode.
Postpartum, the fundus of the contracted uterus lies slightly below the umbilicus. It consists mostly of myometrium covered by serosa and internally lined by basal decidua. The anterior and posterior walls, which lie in close apposition, are each 4 to 5 cm thick (Buhimschi, 2003). At this time, the uterus weighs approximately 1000 g. Because blood vessels are compressed by the contracted myometrium, the uterus on section appears ischemic compared with the reddish-purple hyperemic pregnant organ.
Myometrial involution is a truly remarkable tour de force of destruction or deconstruction that begins as soon as 2 days after delivery as shown in Figure 36-2. As emphasized by Hytten (1995), studies that describe the degree of decreasing uterine weight postpartum are poor quality. Best estimates are that by 1 week, the uterus weighs approximately 500 g; by 2 weeks, about 300 g; and at 4 weeks, involution is complete and the uterus weighs approximately 100 g. After each successive delivery, the uterus is usually slightly larger than before the most recent pregnancy. The total number of myocytes does not decrease appreciably—rather, their size decreases markedly.
FIGURE 36-2 Cross sections of uteri made at the level of the involuting placental site at varying times after delivery. p.p. = postpartum. (Redrawn from Williams, 1931.)
Sonographic Findings
Uterine size dissipates rapidly in the first week (Fig. 36-3). And the uterus and endometrium return to pregravid size by 8 weeks after delivery (Belachew, 2012; Steinkeler, 2012). In a study of 42 normal women postpartum, Tekay and Jouppila (1993) identified fluid in the endometrial cavity in 78 percent at 2 weeks, 52 percent at 3 weeks, 30 percent at 4 weeks, and 10 percent at 5 weeks. Demonstrable uterine cavity contents are seen for up to 2 months following delivery. Belachew and colleagues (2012) used 3-dimensional sonography and visualized intracavitary tissue matter in a third on day 1, 95 percent on day 7, 87 percent on day 14, and 28 percent on day 28. By day 56, the small cavity was empty. Sohn and associates (1988) described Doppler ultrasound results showing continuously increasing uterine artery vascular resistance during the first 5 days postpartum.
FIGURE 36-3 Sonographic measurements of uterine involution during the first 9 days postpartum. AP = anteroposterior. (Data from Hytten, 1995.)
Decidua and Endometrial Regeneration
Because separation of the placenta and membranes involves the spongy layer, the decidua basalis is not sloughed. The remaining decidua has striking variations in thickness, it has an irregular jagged appearance, and it is infiltrated with blood, especially at the placental site (see Fig. 36-2).
Within 2 or 3 days after delivery, the remaining decidua becomes differentiated into two layers. The superficial layer becomes necrotic and is sloughed in the lochia. The basal layer adjacent to the myometrium remains intact and is the source of new endometrium. This arises from proliferation of the endometrial glandular remnants and the stroma of the interglandular connective tissue.
Endometrial regeneration is rapid, except at the placental site. Within a week or so, the free surface becomes covered by epithelium, and Sharman (1953) identified fully restored endometrium in all biopsy specimens obtained from the 16th day onward. Histological endometritis is part of the normal reparative process. Moreover, microscopic inflammatory changes characteristic of acute salpingitis are seen in almost half of women between 5 and 15 days, but these findings do not reflect infection (Andrews, 1951).
Clinical Aspects
Afterpains. In primiparous women, the uterus tends to remain tonically contracted following delivery. In multiparas, however, it often contracts vigorously at intervals and gives rise to afterpains, which are similar to but milder than labor contractions. These are more pronounced as parity increases and worsen when the infant suckles, likely because of oxytocin release (Holdcroft, 2003). Usually, afterpains decrease in intensity and become mild by the third day. We have encountered unusually severe and persistent afterpains in women with postpartum uterine infections.
Lochia. Early in the puerperium, sloughing of decidual tissue results in a vaginal discharge of variable quantity. The discharge is termed lochia and contains erythrocytes, shredded decidua, epithelial cells, and bacteria. For the first few days after delivery, there is blood sufficient to color it red—lochia rubra. After 3 or 4 days, lochia becomes progressively pale in color—lochia serosa. After approximately the 10th day, because of an admixture of leukocytes and reduced fluid content, lochia assumes a white or yellow-white color—lochia alba. The average duration of lochial discharge ranges from 24 to 36 days (Fletcher, 2012).
Placental Site Involution
Complete extrusion of the placental site takes up to 6 weeks (Williams, 1931). Immediately after delivery, the placental site is approximately palm-sized. Within hours of delivery, it normally consists of many thrombosed vessels that ultimately undergo organization (see Fig. 36-2). By the end of the second week, it is 3 to 4 cm in diameter.
Placental site involution is an exfoliation process, which is prompted in great part by undermining of the implantation site by new endometrial proliferation (Williams, 1931). Thus, involution is not simply absorption in situ. Exfoliation consists of both extension and “downgrowth” of endometrium from the margins of the placental site, as well as development of endometrial tissue from the glands and stroma left deep in the decidua basalis after placental separation. Anderson and Davis (1968) concluded that placental site exfoliation results from sloughing of infarcted and necrotic superficial tissues followed by a remodeling process.
Subinvolution
In some cases, uterine involution is hindered because of infection, retained placental fragments, or other causes. Such subinvolution is accompanied by varied intervals of prolonged lochia as well as irregular or excessive uterine bleeding. On bimanual examination, the uterus is larger and softer than would be expected. Ergonovine (Ergotrate) or methylergonovine (Methergine), 0.2 mg orally every 3 to 4 hours for 24 to 48 hours, is recommended by many, but its efficacy is questionable. Of these, only methylergonovine is currently available in the United States. If there is infection, antimicrobial therapy usually leads to a good response. In an earlier study, Wager and coworkers (1980) reported that a third of these late cases of postpartum metritis are caused by Chlamydia trachomatis. Empirical therapy with azithromycin or doxycycline usually prompts resolution regardless of bacterial etiology.
Another cause of subinvolution is incompletely remodeled uteroplacental arteries (Andrew, 1989; Kavalar, 2012). These noninvoluted vessels are filled with thromboses and lack an endothelial lining. Perivascular trophoblasts are also identified in the vessel walls, suggesting an aberrant interaction between uterine cells and trophoblasts.
Late Postpartum Hemorrhage
The American College of Obstetricians and Gynecologists (2013b) defines secondary postpartum hemorrhage as bleeding 24 hours to 12 weeks after delivery. Clinically worrisome uterine hemorrhage develops within 1 to 2 weeks in perhaps 1 percent of women. Such bleeding most often is the result of abnormal involution of the placental site. It occasionally is caused by retention of a placental fragment or by a uterine artery pseudoaneurysm. Usually, retained products undergo necrosis with fibrin deposition and may eventually form a so-called placental polyp. As the eschar of the polyp detaches from the myometrium, hemorrhage may be brisk. As discussed in Chapter 56 (p. 1118), delayed postpartum hemorrhage may also be caused by von Willebrand disease or other inherited coagulopathies (Lipe, 2011).
In our experiences, few women with delayed hemorrhage are found to have retained placental fragments. Thus, we and others do not routinely perform curettage (Lee, 1981). Another concern is that curettage may worsen bleeding by avulsing part of the implantation site. Thus, in a stable patient, if sonographic examination shows an empty cavity, then oxytocin, methylergonovine, or a prostaglandin analogue is given. Antimicrobials are added if uterine infection is suspected. If large clots are seen in the uterine cavity with sonography, then gentle suction curettage is considered. Otherwise curettage is carried out only if appreciable bleeding persists or recurs after medical management.
URINARY TRACT
Normal pregnancy-induced glomerular hyperfiltration persists on the first postpartum day but returns to prepregnancy baseline by 2 weeks (Hladunewich, 2004). Also, dilated ureters and renal pelves return to their prepregnant state during the course of 2 to 8 weeks postpartum. Because of this dilated collecting system, coupled with residual urine and bacteriuria in a traumatized bladder, urinary tract infection is a concern in the puerperium.
Bladder trauma is associated most closely with labor length and thus to some degree is a normal accompaniment of vaginal delivery. Funnell and colleagues (1954) used cystoscopy immediately postpartum and described varying degrees of submucosal hemorrhage and edema. Postpartum, the bladder has an increased capacity and a relative insensitivity to intravesical pressure. Thus, overdistention, incomplete emptying, and excessive residual urine are common. Their management is discussed on page 676.
It is unusual for urinary incontinence to manifest during the puerperium. That said, much attention has been given to the potential for subsequent development of urinary incontinence and other pelvic floor disorders in the years following delivery. A fuller discussion is found in Chapter 30 (p. 588).
PERITONEUM AND ABDOMINAL WALL
The broad and round ligaments require considerable time to recover from stretching and loosening during pregnancy. As a result of ruptured elastic fibers in the skin and prolonged distention by the pregnant uterus, the abdominal wall remains soft and flaccid. If the abdomen is unusually flabby or pendulous, an ordinary girdle is often satisfactory. An abdominal binder is at best a temporary measure. Several weeks are required for these structures to return to normal, and recovery is aided by exercise. These may be started anytime following vaginal delivery and as soon as abdominal soreness diminishes after cesarean delivery. Silvery abdominal striae commonly develop as striae gravidarum (Chap. 4, p. 51). Except for these, the abdominal wall usually resumes its prepregnancy appearance. When muscles remain atonic, however, the abdominal wall also remains lax. Marked separation of the rectus abdominis muscles—diastasis recti—may result.
HEMATOLOGICAL PARAMETERS AND PREGNANCY HYPERVOLEMIA
Hematological and Coagulation Changes
Marked leukocytosis and thrombocytosis may occur during and after labor. The white blood cell count sometimes reaches 30,000/μL, with the increase predominantly due to granulocytes. There is a relative lymphopenia and an absolute eosinopenia. Normally, during the first few postpartum days, hemoglobin concentration and hematocrit fluctuate moderately. If they fall much below the levels present just before labor, a considerable amount of blood has been lost (Chap. 41, p. 781).
By the end of pregnancy, there are many changes in laboratory findings that assess coagulation (Kenny, 2014). These are discussed in Chapter 4 (p. 57) and listed in the Appendix (p. 1288). Many persist in the puerperium. One example is that the markedly increased levels of plasma fibrinogen are maintained at least through the first week, and hence, so is the increase in sedimentation rate.
Pregnancy-Induced Hypervolemia
When the amount of blood attained by normal pregnancy hypervolemia is lost as postpartum hemorrhage, the woman almost immediately regains her nonpregnant blood volume (Chap. 41, p. 781). If less has been lost at delivery, it appears that in most women, blood volume has nearly returned to its nonpregnant level by 1 week after delivery. Cardiac output usually remains elevated for 24 to 48 hours postpartum and declines to nonpregnant values by 10 days (Robson, 1987). Heart rate changes follow this pattern. Systemic vascular resistance and blood pressure rise (Fig. 36-4). Systemic vascular resistance remains in the lower range characteristic of pregnancy for 2 days postpartum and then begins to steadily increase to normal nonpregnant values (Hibbard, 2014).
FIGURE 36-4 During the early puerperium, blood pressure normally rises toward nonpregnant values. MAP = mean arterial pressure.
Normal pregnancy is associated with an appreciable increase in extracellular sodium and water, and postpartum diuresis is a physiological reversal of this process. Chesley and coworkers (1959) demonstrated a decrease in sodium space of approximately 2 L during the first week postpartum. This also corresponds with loss of residual pregnancy hypervolemia. In preeclampsia, pathological retention of fluid antepartum and its diuresis postpartum may be prodigious (Chap. 40, p. 768).
Postpartum diuresis results in relatively rapid weight loss of 2 to 3 kg, which is added to the 5 to 6 kg incurred by delivery and normal blood loss. Weight loss from pregnancy itself is likely to be maximal by the end of the second week postpartum. It follows that any residual increased weight compared with prepregnancy values probably represents fat stores that will persist. According to Schauberger and associates (1992), women approach their self-reported prepregnancy weight 6 months after delivery but still retain an average surplus of 1.4 kg (3 lb).
BREASTS AND LACTATION
Breast Anatomy and Products
Each mature mammary gland or breast is composed of 15 to 25 lobes. They are arranged radially and are separated from one another by varying amounts of fat. Each lobe consists of several lobules, which in turn are composed of numerous alveoli. Each alveolus is provided with a small duct that joins others to form a single larger duct for each lobe as shown in Figure 36-5. These lactiferous ducts open separately on the nipple, where they may be distinguished as minute but distinct orifices. The alveolar secretory epithelium synthesizes the various milk constituents, described next.
FIGURE 36-5 Schematic of the alveolar and ductal system during lactation. Note the myoepithelial fibers (M) that surround the outside of the uppermost alveolus. The secretions from the glandular elements are extruded into the lumen of the alveoli (A) and ejected by the myoepithelial cells into the ductal system (D), which empties through the nipple. Arterial blood supply to the alveolus is identified by the upper right arrow and venous drainage by the arrow beneath.
After delivery, the breasts begin to secrete colostrum, which is a deep lemon-yellow liquid. It usually can be expressed from the nipples by the second postpartum day. Compared with mature milk, colostrum is rich in immunological components and contains more minerals and amino acids (Ballard, 2013). It also has more protein, much of which is globulin, but less sugar and fat. Secretion persists for 5 days to 2 weeks, with gradual conversion to mature milk by 4 to 6 weeks. The colostrum content of immunoglobulin A (IgA) offers the newborn protection against enteric pathogens. Other host resistance factors found in colostrum and milk include complement, macrophages, lymphocytes, lactoferrin, lactoperoxidase, and lysozymes.
Mature milk is a complex and dynamic biological fluid that includes fat, proteins, carbohydrates, bioactive factors, minerals, vitamins, hormones, and many cellular products. The concentrations and contents of human milk change even during a single feed and are influenced by maternal diet, as well as infant age, health, and needs. A nursing mother easily produces 600 mL of milk daily, and maternal gestational weight gain has little impact on its quantity or quality (Institute of Medicine, 1990). Milk is isotonic with plasma, and lactose accounts for half of the osmotic pressure. Essential amino acids are derived from blood, and nonessential amino acids are derived in part from blood or synthesized in the mammary gland. Most milk proteins are unique and include α-lactalbumin, β-lactoglobulin, and casein. Fatty acids are synthesized in the alveoli from glucose and are secreted by an apocrine-like process. Most vitamins are found in human milk, but in variable amounts. Vitamin K is virtually absent, and thus, an intramuscular dose is given to the newborn (Chap. 33, p. 644). Vitamin D content is low—22 IU/mL. Thus, newborn supplementation is also recommended by the American Academy of Pediatrics (Wagner, 2008).
Whey is milk serum and has been shown to contain large amounts of interleukin-6 (Saito, 1991). Human milk has a whey-to-casein ratio of 60:40, considered ideal for absorption. Prolactin appears to be actively secreted into breast milk (Yuen, 1988). Epidermal growth factor (EGF) has been identified in human milk. And because it is not destroyed by gastric proteolytic enzymes, it may be absorbed to promote growth and maturation of newborn intestinal mucosa (McCleary, 1991). Other critical components in human milk include lactoferrin, melatonin, oligosaccharides, and essential fatty acids.
Endocrinology of Lactation
The precise humoral and neural mechanisms involved in lactation are complex. Progesterone, estrogen, and placental lactogen, as well as prolactin, cortisol, and insulin, appear to act in concert to stimulate the growth and development of the milk-secreting apparatus (Porter, 1974). With delivery, there is an abrupt and profound decrease in the levels of progesterone and estrogen. This decrease removes the inhibitory influence of progesterone on α-lactalbumin production and stimulates lactose synthase to increase milk lactose. Progesterone withdrawal also allows prolactin to act unopposed in its stimulation of α-lactalbumin production. Serotonin is produced in mammary epithelial cells and has a role in maintaining milk production. This may explain the decreased milk production in women taking selective serotonin-reuptake inhibitors—SSRIs (Collier, 2012).
The intensity and duration of subsequent lactation are controlled, in large part, by the repetitive stimulus of nursing and emptying of milk from the breast. Prolactin is essential for lactation, and women with extensive pituitary necrosis—Sheehan syndrome—do not lactate (Chap. 58, p. 1163). Although plasma prolactin levels fall after delivery to levels lower than during pregnancy, each act of suckling triggers a rise in levels (McNeilly, 1983). Presumably a stimulus from the breast curtails the release of dopamine, also known as prolactin-inhibiting factor, from the hypothalamus. This in turn transiently induces increased prolactin secretion.
The posterior pituitary secretes oxytocin in pulsatile fashion. This stimulates milk expression from a lactating breast by causing contraction of myoepithelial cells in the alveoli and small milk ducts (see Fig. 36-4). Milk ejection, or letting down, is a reflex initiated especially by suckling, which stimulates the posterior pituitary to liberate oxytocin. The reflex may even be provoked by an infant cry and can be inhibited by maternal fright or stress.
Immunological Consequences of Breast Feeding
Human milk contains several protective immunological substances, including secretory IgA and growth factors. The antibodies in human milk are specifically directed against maternal environmental antigens such as against Escherichia coli (Iyengar, 2012). As a result, breast-fed infants are less prone to enteric infections than bottle-fed ones (Cravioto, 1991). Human milk also provides protection against rotavirus infections, a major cause of infant gastroenteritis (Newburg, 1998). Moreover, the risks of atopic dermatitis and respiratory infections are reduced (Ip, 2009). Bartick and Reinhold (2010) calculated that significant economic burdens from pediatric disease could be lessened by improved breast-feeding rates.
Much attention has been directed to the role of maternal breast-milk lymphocytes in neonatal immunological processes. Milk contains both T and B lymphocytes, but the T lymphocytes appear to differ from those found in blood. Specifically, milk T lymphocytes are almost exclusively composed of cells that exhibit specific membrane antigens. These memory T cells appear to be an avenue for the neonate to benefit from the maternal immunological experience (Bertotto, 1990).
Nursing
Human milk is ideal food for neonates. It provides age-specific nutrients as well as immunological factors and antibacterial substances (American College of Obstetricians and Gynecologists, 2013a). Milk also contains factors that act as biological signals for promoting cellular growth and differentiation. The American Academy of Pediatrics (2012) has provided a list of dose-response benefits of nursing (Table 36-2). For both mother and infant, the benefits of breast feeding are long-term. For example, women who breast feed have a lower risk of breast and reproductive cancer, and their children have increased adult intelligence independent of a wide range of possible confounding factors (Jong, 2012; Kramer, 2008). Breast feeding is associated with decreased postpartum weight retention (Baker, 2008). In addition, rates of sudden-infant-death syndrome are significantly lower among breast-fed infants. Last, in the Nurses’ Health Study, women who reported breast feeding for at least 2 cumulative years had a 23-percent lower risk of coronary heart disease (Stuebe, 2009). For all these reasons, the American Academy of Pediatrics (2012) supports the World Health Organization (2011) recommendations of exclusive breast feeding for up to 6 months, with avoidance of exposure to cow milk proteins.
TABLE 36-2. Advantages of Breast Feeding
Nutritional
Immunological
Developmental
Psychological
Social
Economical
Environmental
Optimal growth and development
Decrease risks for acute and chronic diseases
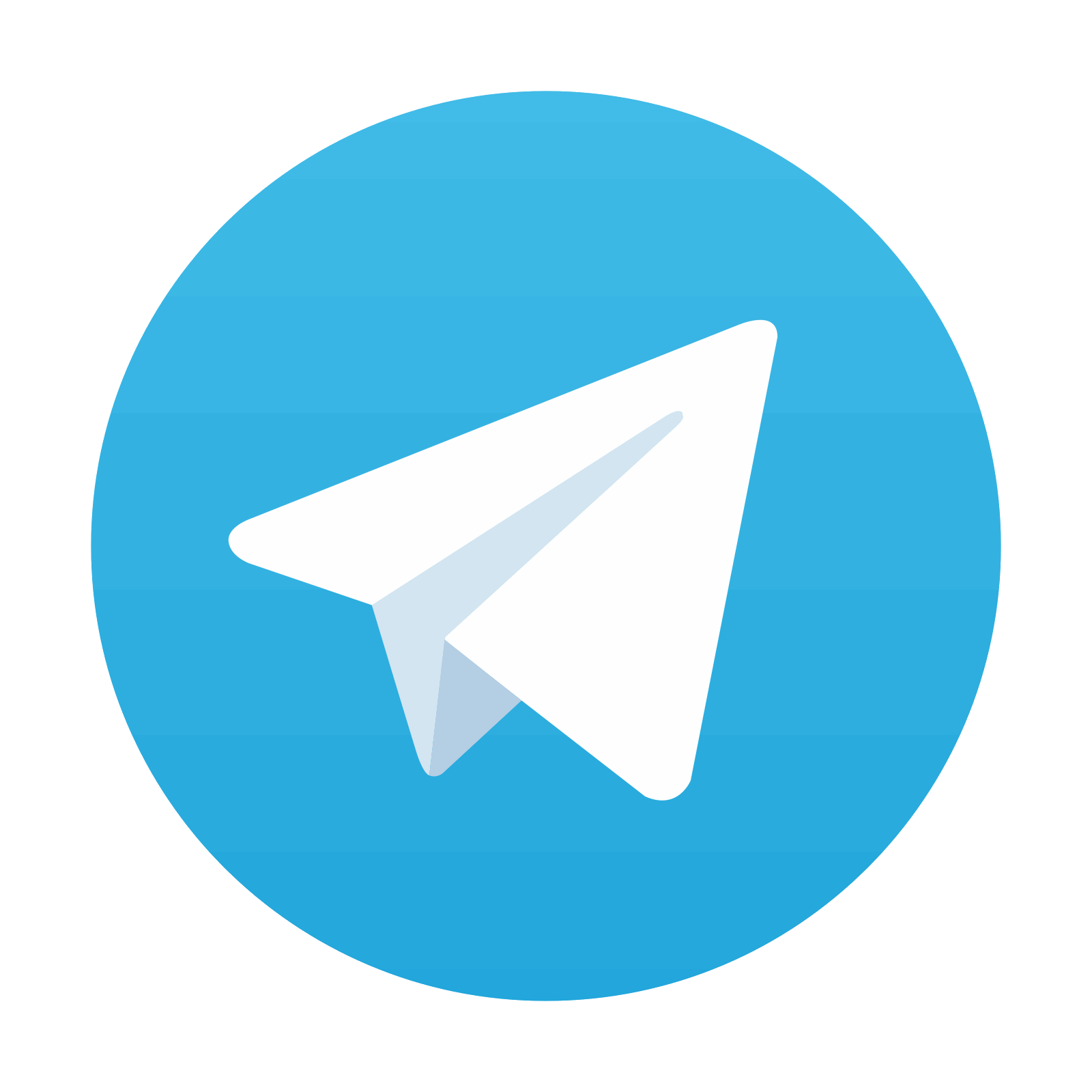
Stay updated, free articles. Join our Telegram channel

Full access? Get Clinical Tree
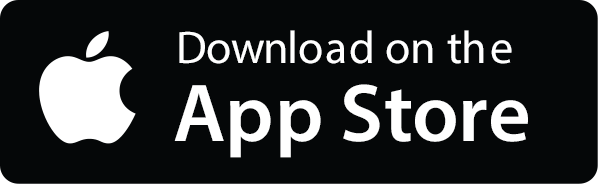
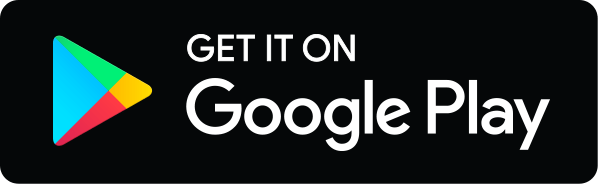