Delaying childbirth increases the proportion of advanced maternal age pregnancies. This increases the number of pregnancies requiring invasive prenatal testing. Prenatal diagnosis of chromosomal aneuploidies and monogenic disorders requires fetal cells obtained through invasive procedures (i.e. chorionic villus sampling and amniocentesis). These procedures carry a risk of fetal loss, which causes anxiety to at-risk couples. Intact fetal cells entering maternal circulation have raised the possibility of non-invasive prenatal diagnosis. Rarity of fetal cells, however, has made it challenging. Fetal nucleated red blood cells are ideal candidate target cells because they have limited lifespan, contain true representation of fetal genotype, contain specific fetal cell identifiers (embryonic and fetal globins), and allow interrogation with chromosomal fluorescence in-situ hybridisation and possibly with array comparative genomic hybridisation. The utility of fetal nucleated red blood cells in non-invasive prenatal diagnosis has not reached clinical application because of the inconsistencies in enrichment strategies and rarity of cells.
Invasive prenatal diagnostic procedures
Current methods to detect fetal chromosomal and genetic abnormalities accurately are invasive procedures. Amniocentesis carried out from 15 weeks onwards, coupled with recent advances in technology, allows rapid, accurate detection of chromosomal aneuploidies and genetic disorders using uncultured amniocytes. The additional risk of miscarriage after amniocentesis, however, is up to 1%. Chorionic villus sampling (CVS) has a slightly higher miscarriage risk than that of amniocentesis carried out after 15 weeks gestation. Fetal blood sampling is carried out after 20 weeks’ gestation and has miscarriage rates of up to 2.3%.
Fetal nucleated cells in maternal blood
In 1893, Schmorl, a German pathologist, first documented the presence of fetal-derived trophoblast sprouts in the lungs of 17 women who died from complications of eclampsia. Definitive evidence that fetal cells circulate in maternal blood came in 1969 when lymphocytes carrying an X and a Y chromosome were detected in the peripheral blood of pregnant women carrying male fetuses. More compelling evidence of the existence of fetal cells in maternal blood came in the 1990s with the development of modern and sophisticated molecular techniques, such as polymerase chain reaction (PCR) and fluorescence in-situ hybridisation (FISH) to detect fetal DNA sequences from cellular components of maternal blood.
Fetal cells have been recovered and identified from maternal blood with the use of various enrichment and identification techniques. Despite considerable progress in detecting and isolating fetal cells from maternal blood, however, reproducibility and reliability remain poor. This is likely attributed to the rarity and variability of fetal cells among pregnancies.
Prevalence of fetal cells in maternal blood
Numerous studies have suggested that fetal cells are rare in maternal blood. Using methods such as PCR and chromosomal FISH (cFISH), it has been suggested that the prevalence of these cells ranged between 10 −4 and 10 −8 .
Studies have shown increased feto–maternal cellular trafficking in some abnormal pregnancies. In pregnancies affected by pre-eclampsia and before the onset of pre-eclampsia symptoms, increased numbers of fetal cells were found in the maternal circulation, which was confirmed by other studies. Furthermore, it was shown that, in pregnancies bearing a trisomy 21 fetus, a six-fold elevation of fetal cells occurred in the maternal blood, and between two to five times higher in other aneuploid pregnancies, including trisomy 18, 13, and triploidy (69,XXX).
Fetal cells detected in maternal blood can be used for non-invasive prenatal diagnosis, as shown in diagnosing fetal chromosomal aneuploidies and single-gene disorders, such as Mendelian disorders, human leukocyte antigen (HLA) polymorphisms and fetal Rhesus D genotype. A large clinical trial (The National Institute of Health Fetal Cell Study) studied fetal cells isolated from maternal blood, and revealed a 74.4% detection rate in the determination of common aneuploidies (13, 18, 21, X and Y), with a false–positive rate as low as 0.6%. This false–positive rate is slightly superior to current non-invasive methods, such as ultrasound or serum screening. The true prevalence of fetal cells in maternal blood will likely be determined only after the problems of enrichment and identification have been overcome.
Candidate target cells for non-invasive diagnosis
Since the observation of fetal cell trafficking into maternal circulation in 1893, a variety of other fetal cell types (trophoblast, leukocytes, haematopoietic stem cells and fetal nucleated red blood cell [FNRBC]) were discovered to be circulating in the maternal blood. We subsequently highlight the different types of fetal cells isolated from maternal blood, efforts of enrichment and the merit of these cells towards use in NIPD.
Trophoblasts
Trophoblasts are viewed as an attractive cell type for non-invasive prenatal diagnosis, being the first fetal cell type detected to have crossed into maternal circulation. A novel method of enrichment of trophoblast cells has been examined based on cell size using the isolation by size of epithelial tumour or trophoblastic cells coupled with micro-dissection. Here, it was possible to prenatally diagnose spinal muscular atrophy and cystic fibrosis successfully for non-invasive prenatal diagnosis. Many others have confirmed the presence of trophoblast in the maternal circulation.
Drawbacks to using trophoblast cells for NIPD include the following: rapid clearing by the pulmonary circulation in normal pregnancies, lack of highly specific antibodies for enrichment, about 1% incidence of chromosomal mosaicism, and are multinucleated cells, therefore making FISH analysis of aneuploidies difficult.
Leukocytes
The first reported feto–maternal lymphocyte transfer was described by the detection of Y chromosome in mitogen-stimulated lymphocytes in blood cells from pregnant women bearing male fetuses. Subsequently, leukocytes were enriched using antibodies against paternally derived human leukocyte antigens (HLA) from maternal blood. Clinical application of this cell type, however, was limited owing to engraftment of fetal lymphocytes and persistence in the maternal blood in the post-partum years. Bianchi et al. showed the presence of extremely scarce CD34 + cells with stem-cell like properties that persisted in the maternal circulation for as long as 27 years, thus restricting its use for the diagnosis of ongoing pregnancies. Usage of these cells for NIPD also required prior HLA testing of both parents before cell enrichment was carried out.
Stem cells and progenitors
If fetal cells could easily be expanded in vitro, the problem of the rarity of fetal cells in maternal blood could be solved. A solution would be to consider fetal stem cells or progenitors that are present in maternal circulation. Although successes of in-vitro expansion of fetal erythroid progenitors isolated from maternal blood have been reported, data were not reproducible. Owing to their higher proliferative potential, some studies focused on CD34 + fetal cells. Their potential for use in NIPD, however, was limited by the low number of CD34 + fetal cells and the inability to consistently recover CD34 + fetal cells from maternal samples. These fetal CD34 + cells are able to engraft and may persist in the maternal circulation for as long as 27 years postpartum.
Fetal mesenchymal stem cells (MSC) possess morphologic, immunophenotypic, and functional characteristics similar to adult-derived MSC. Enrichment of fetal MSC, however, from maternal blood obtained after termination were unsuccessful. The rarity of fetal MSC and unique ability to differentiate and proliferate similar to adult stem cells may cause fetal MSC from previous pregnancies to engraft in maternal tissue and persist in the maternal circulation, suggesting only a limited role in NIPD.
Fetal erythroblasts or fetal nucleated red blood cells
The choice of FNRBC as ideal target cells is based on the following parameters: it has a limited life span in the maternal circulation, it can be differentiated morphologically from maternal cells, possesses cell-surface markers for enrichment, bears complete complement of nuclear genes, and carries developmentally specific markers (e.g. embryonic globin). Fetal nucleated red blood cells are found in maternal blood consistently, and are the predominant fetal cell type in the first trimester fetal blood.
Bianchi et al. successfully enriched FNRBCs using anti-CD71 and fluorescence-activated cell sorting (FACS) in three-quarters of maternal blood samples. Wachtel et al. enriched FNRBCs and determined fetal gender accurately in 94% of pregnancies by PCR amplification of Y-chromosome-specific sequences. Bianchi et al. showed that gender determination could be achieved with the use of enriched FNRBCs from either anti-glycophorin A (GPA) alone or in combination with anti-CD71 or anti-CD36 enrichment in all maternal blood samples.
Although erythroblast can be consistently enriched from maternal blood, it has been reported that only 30–50% are fetal in origin. Troeger et al. used single-cell PCR analysis, and showed that only one-half the circulating erythroblast were of fetal origin.
Price et al. had the earliest reported use of enriched FNRBCs for prenatal diagnosis, whereby trisomy 21 and trisomy 18 were correctly identified using FNRBCs enriched from maternal blood. Since then, FNRBCs enriched from maternal blood has led to the detection of other chromosomal abnormalities. The promising results led to the large scale clinical trial using FNRBCs in maternal blood for the detection of fetal aneuploidies and determination of fetal gender.
Enrichment of fetal-nucleated red blood cells for non-invasive prenatal diagnosis: current state of the art
The rarity of FNRBCs in maternal blood requires a simple, consistent and rapid protocol for their isolation to be developed before their clinical use for NIPD. Many approaches (e.g. centrifugation, filtration, lateral displacement, FACS, magnetic-activated cell sorting [MACS], magnetophoresis, lectin-binding method, dielectrophoresis [DEP], micromanipulation and laser capture and micro-dissection) were developed to recover FNRBCs from maternal blood in the past decade.
Centrifugation
Density-gradient centrifugation can be useful to deplete the overwhelming abundance of maternal red blood cells (density of FNRBCs is similar to that of mononuclear cells and slightly lower than red blood cells) as the initial enrichment step. With Percoll or Ficoll density solutions, single denser gradients are progressively preferred and, currently, many investigators prefer the use of Ficoll 1119 In a study comparing different density gradient solutions, Choolani et al. showed that Percoll 1118 yielded better recovery rates (64.1%) in the separation of first-trimester primitive FNRBCs in model mixtures compared with Ficoll 1119 (32%).
In a population of pregnant women at risk of beta-thalassaemia, a novel gradient using Percoll and high-speed centrifugation successfully enriched zeta-globin stained fetal erythroblast, which were micro-manipulated singly and amplified individually by PCR. Fetal beta-globin genotype was correctly identified in four of the six maternal blood tested.
Density-gradient centrifugation is by far the most widely used method; however, it is associated with an inevitably high loss of FNRBCs, usually between 30% and 70%. Furthermore, the resulting FNRBCs are dispersed in a large number of maternal white blood cells. Therefore, further enrichment is needed, usually by an antibody-dependent process such as FACS or MACS.
Fluorescence-activated cell sorting
Fluorescence-activated cell sorting is one of the most common techniques for isolation of fetal cells from maternal blood. Compared with immunomagnetic cell sorting, FACS cell sorting is based on differential antigen density, and generally gives higher purity of target cells. Furthermore, FACS allows multiparameter sorting: simultaneous analysis of several criteria on a single cell and can be adapted for use with intracytoplasmic antigens. After Herzenberg et al. showed enrichment of fetal cells by FACS, several others followed suit.
The presence of an antigen or marker that is highly specific for the FNRBCs would be ideal, as it could be used to isolate these cells from maternal blood and identify their fetal origin. DeMaria et al. capitalised on the multi-parameter sorting ability of FACS to combine an intracellular staining with anti-γ-globin monoclonal antibody and Hoechst 33342 (nuclear dye) to enrich FNRBCs from model mixtures of male umbilical cord cells and peripheral blood mononuclear cells from non-pregnant women. The recovery of FNRBCs varied between 39 and 100%, with purity between 59 and 73%. Fluorescence in-situ hybridisation analysis confirmed that almost all of γ-globin + enriched cells were male (98%) and of γ-globin − cells were female (92.5%). Targeting intracellular antigens, however, is subjected to cell loss as erythroid cells may lyse during permeabilisation steps.
In view of this, investigators used antibodies against surface antigens such as CD45 (lymphocyte specific) to deplete maternal nucleated lymphocytes, GPA (red blood cell specific), or both, to enrich FNRBCs from blood samples. Glycophorin A separation often causes agglutination of maternal anucleated red blood cells, thereby changing both light scatter and fluorescence properties of cells by FACS and complicating FISH analysis. This posed a difficulty in using anti-GPA for selecting FNRBCs for FACS. Bianchi et al. evaluated the effectiveness of three monoclonal antibodies: CD71, CD36 and GPA either used alone or in combination for the separation of FNRBCs from maternal blood by FACS. Used alone, CD71 and CD36 accurately predicted gender in 57% and 88%, respectively. The use of GPA alone or in combination with CD71 or CD36, however, accurately predicted gender in 100% of maternal blood tested. Therefore, the use of CD71 alone for FNRBC enrichment from maternal blood may be inadequate. Choolani et al. have reported that only a minority of FNRBCs in first-trimester healthy pregnancies express CD71, and those that were CD71 positive showed weak expression levels compared with that of definitive FNRBCs.
Use of FACS has many limitations, of main concern is its low recovery and low throughput. In addition, machines are expensive, therefore unavailable in many laboratories, and specially trained staff are needed to operate machine.
Magnetic-activated cell sorting
Magnetic-activated cell sorting was recommended for use in enrichment of FNRBCs from maternal blood for NIPD after successful isolation was reported. Magnetic-activated cell sorting is a simple, fast and economical bench-top technique with the capability to process large cell numbers. By exploiting the antigenic differences between cells, positive selection or depletion by MACS can be carried out. Disadvantages of MACS is that positive selection or negative depletion can only be carried out one antigen at a time, and that purity of selected population is poorer compared with FACS separation ; however, recovery is superior to FACS.
Most of the antibodies currently used for isolation of fetal cells from maternal blood are not specific to fetal cells, and may therefore recognise and co-purify adult cells leading to problems of maternal contamination. As no surface antigens are known to be specific for FNRBCs, it is likely that a combination of antibodies will be required to separate FNRBCs from the other cells in the maternal blood. Surface antigens that are potentially useful include GPA, CD36, CD35, CD45, CD47, CD71 and erythropoietin receptor (EPO-r). Glycophorin-A (GPA) is expressed on all erythroid lineage cells while CD45 is absent. Transferrin receptor (CD71) is expressed on the entire erythroid lineage, activated lymphocytes, monocytes, trophoblasts, any cell incorporating iron and definitive FNRBCs in maternal blood. Beyond the reticulocyte stage, CD36, EPO-r and CD71 expression are lost, whereas CD35 is expressed only on mature erythrocytes.
After density-gradient centrifugation, enrichment of FNRBCs could be further enhanced using surface markers such as CD71, EPO-r, CD36 alone or in combination with GPA. By combining CD45 with CD14 to deplete unwanted leukocytes followed by positive selection with CD71, Reading et al. were able to recover FNRBCs from 87% of maternal blood studied. Others have used a combination of CD32 to deplete maternal leukocytes cells and CD71 to select FNRBCs with limited success.
Filtration on chip
Mohamed et al. studied the native characteristics of FNRBCs, such as size and deformability, and capitalised upon them. Channels and pillars were etched into their device, which allowed cells to deform and reform. Initial trials with fetal cord blood proved successful with the confirmation of enriched cells to be of fetal origin. This device, however, has certain drawbacks: (1) it does not sort whole blood; (2) sample preparation involves density gradient centrifugation and only mononuclear layer is passed though the device; (3) cell adhesion to channel wall; (4) it has low flow rates (0.35 ml/h).
Lee et al. reported proof of concept experiments using a micro-fluidic device that showed promising enrichment of epsilon-positive FNRBCs (e + FNRBCs) from model mixtures containing e + FNRBCs and adult red blood cells. The device (using flow rate of 0.3 ml/min) gave the best recovery of e + FNRBCs (74%), with a depletion of about one-half the adult red blood cells from the model mixture. Optimal flow rate of 0.3 ml/min suggests that the device could theoretically sort 20 ml of maternal blood in just over an hour.
Lateral displacement and magnetophoresis
Huang et al. combined a filtration device and deterministic lateral displacement with magnetophoresis, a magnetic device for haemoglobin-based cell isolation. Fetal-nucleated red blood cell were sorted on the basis of unique characteristics, namely cell size, presence of nucleus and haemoglobin content. Deterministic lateral displacement method was used as a preliminary step to de-bulk adult red blood cells by taking advantage of the of the hydrodynamic size differences between nucleated and non-nucleated cells. Next, cells containing haemoglobin become paramagnetic when deoxygenated and, in a magnetic field, these cells move in a different direction allowing separation from other cells. The investigators reported effective depletion of erythrocytes from whole maternal blood sample (over 99.9% efficacy) without the prior need to de-bulk maternal erythrocytes. They isolated an average of 38 cells/ml of maternal blood from 58 pregnant women. A question that remains to be addressed, however, is whether the recovered cells are truly fetal and not of maternal origin, as the cells were only identified by May-Giemsa stain.
Lectin-binding method
Wada and Kitagawa observed differences of the sugar moiety and protein glycosylation on the surfaces of FNRBCs and red blood cells, and developed a novel cell-separation strategy using differential lectin-binding selection. Soybean agglutinin (SBA), a lectin, recognises galactose and N-acetylgalactosamine moieties on the membrane surface of cells. Several reports on the successful use of SBA in enrichment of FNRBCs from maternal blood. Although SBA-lectin method recovered eight-fold more FNRBCs per millilitre of maternal blood compared with MACS, purity was significantly poorer in the SBA-lectin method.
Dielectrophoresis
Dielectrophoresis microfluidic device is an electrokinetic movement of neutral particles induced by polarisation in an asymmetric electric field, and manipulations can be applied onto cells based on the differences in their dielectrophoretic properties. Several dielectrophoresis-based devices have been developed and found suitable for application in isolation of single-cell population.
Micromanipulation
Takabayashi et al. were the first to report on the analysis of micro-manipulated single fetal erythroblast cells, and successfully predicted fetal gender in 10 out of the 11 maternal blood samples. Similar methods were used to diagnose Duchenne’s muscular dystrophy, Rhesus D and haemoglobinopathies from micromanipulated fetal cells obtained from maternal blood. Several studies using classical methods of micromanipulation reported feasibility of this method to obtain genetic complement of the fetus. The use of micromanipulation remains limited, however, owing to special equipment needed, expertise and by the significant loss of cells during transfer process when cells are not under direct vision.
Laser micro-dissection and pressure catapulting
Advances in PCR, FISH and whole-genome amplification has enabled amplification of minute quantities of DNA (even a single copy) greater than 1 billion-fold, which has been exploited for the prenatal diagnosis of monogenic disorders from maternal blood and pre-implantation genetic diagnosis. PALM ® MicroBeam system is one such device that would be suitable for retrieval of pure population of cells that fully automates identification, selection, and capture of any cell type of interest for downstream genetic analysis for non-invasive prenatal diagnosis.
More recently, Kroneis et al. used the PALM ® Zeiss system, and successfully combined automatic screening for rare target cells based on immunofluorescence staining with isolation of single target cell by laser micro-dissection and pressure catapulting. Target cells were confirmed by DNA fingerprinting carried out on low-volume on slide PCR. DNA profiling successfully identified isolated target cells from mixed cells of non-related individuals (100%) and in mixed cells from related individuals (86%). Further evaluation of laser micro-dissection and pressure catapulting is warranted.
Identification of enriched fetal nucleated red blood cell from maternal blood
The accuracy of using fetal cells from maternal blood for prenatal diagnosis depends largely on the specificity of their identification. Different methods are used to identify fetal cells, which include cell morphology, immune-labelling with embryonic (epsilon [Ɛ] and zeta [ζ]) and fetal (gamma [γ]) globin, molecular analyses and FISH.
Fetal nucleated red blood cell were confirmed fetal in origin by MAb intracytoplasmic labelling, using developmentally specific fetal haemoglobin such as γ-, ζ- and Ɛ-globins. Currently, most researchers favour the use of γ-globin for FNRBC identification. The use of γ-globin, however, has its limitations, as a minority of maternal NRBCs express γ-globin, especially in an acute erythroid expansion such as in pregnancy, recovery from iron-deficiency anaemia and patients with beta-thalassaemia.
This led to the use of embryonic ζ-globin, which has limited use as the expression of ζ-globin is not completely absent in adults, such as the case of adults with alpha-thalassaemia trait. In addition, ζ-globin expression is not completely downregulated, as it is expressed in a fraction of definitive erythrocytes from second-trimester onwards.
Epsilon-globin has been shown to be fetal specific and gender independent. Comparison of specificity between the two embryonic globins (Ɛ and ζ) in the detection of FNRBCs in CVS supernatant fluid, ζ -globin was found to be more reliable and specific for the detection of FNRBCs.
Diagnosis of chromosomal and monogenic disorders by analysing fetal nucleated red blood cell from maternal blood
With the advent of important molecular techniques such as PCR, chromosomal FISH and array comparative genomic hybridisation the clinical utility of FNRBCs enriched from maternal blood for NIPD was explored.
Polymerase chain reaction
Investigators in this field of research use PCRs technological ability to amplify minute quantities of DNA over a billion-fold. Prenatal diagnosis of the haemoglobin (Hb) Lepore-Boston, X-linked ornithine transcarbamylase deficiency syndrome and Down’s syndrome was achieved by PCR.
Real time-polymerase chain reaction
Many more copies of the mRNA of an expressed gene exist than there are the genomic sequences within the genome, and therefore PCR of mRNA can be more sensitive than of genomic DNA. Reports, however, show that fetal mRNA is more vulnerable to enzymatic degradation in maternal blood compared with fetal DNA.
Chromosomal fluorescence in-situ hybridisation
The cFISH method is commonly used for NIPD to detect aneuploidies such as trisomies 13, 18 and 21 on FNRBCs enriched from maternal blood. Other chromosomal aberrancies can be diagnosed by FISH analysis of enriched fetal cells: Cacheux et al. showed detected sex chromosome aneuploidy, and Bischoff et al. could detect sex chromosome mosaicism in four XXY cells after thorough analysis of 500 metaphase spread which were missed by their routine cytogenetic protocol (only 15 metaphases examined). Fluorescence in-situ hybridisation, however, is not sufficiently specific with an error rate of 1 in 1000. In order to increase specificity two different Y probes can be used.
Array comparative genomic hybridisation
Array comparative genomic hybridisation is a technique that allows scanning of the entire genome for genomic copy number variation. This technique has been shown to be useful in preimplantation genetic diagnosis and prenatal diagnosis using fetal DNA obtained invasively (i.e. CVS and amniocentesis). The utility of array comparative genomic hybridisation, however, for non-invasive prenatal diagnosis remains to be seen.
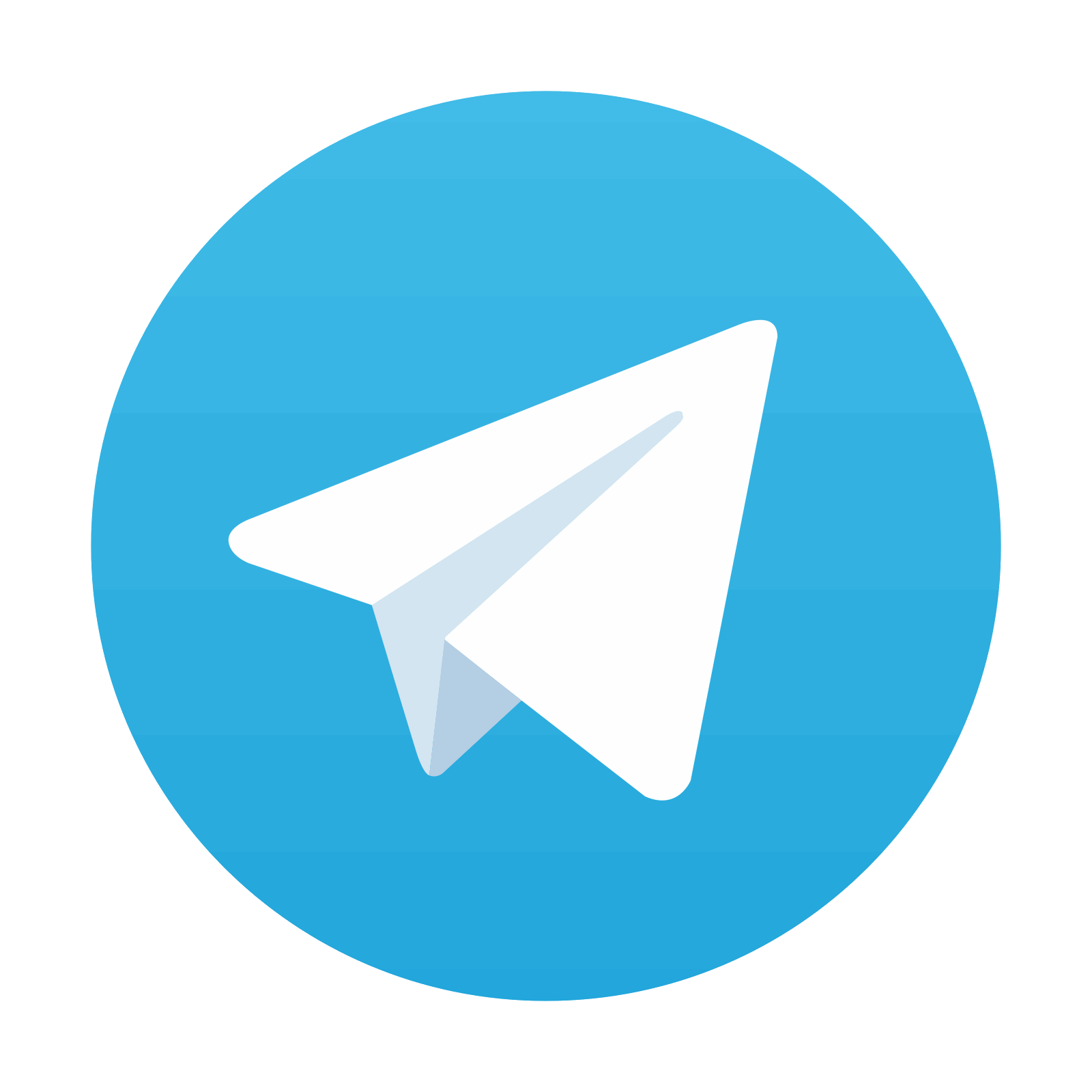
Stay updated, free articles. Join our Telegram channel

Full access? Get Clinical Tree
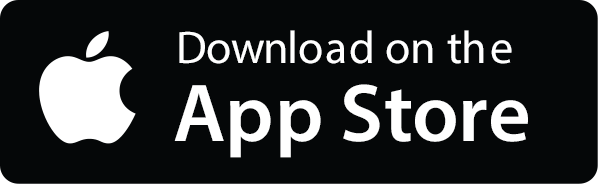
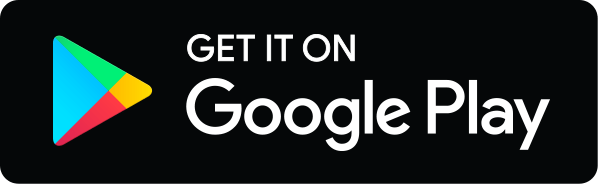