The Premature Newborn
Steven M. Peterec
Joseph B. Warshaw
Preterm delivery is probably the most important problem in obstetrics and neonatology today. Over 400,000 preterm births occur each year in the United States. It is the leading cause of perinatal, neonatal, and infant mortality. Approximately two-third of infant deaths in the United States occur in the 7% to 8% of infants born with a birth weight of less than 2,500 g. A higher rate of preterm delivery is a major reason that the United States has one of the highest infant mortality rates among developed nations. Preterm births also are responsible for a large proportion of long-term neurodevelopmental handicaps in children. It is estimated that preterm births account for approximately one-third of all health care spending for infants and a tenth of all health care spending for children in the United States.
DEFINITIONS AND TERMINOLOGY
The fetus or newborn infant is defined as term during the interval between 37 and 42 weeks after the onset of the mother’s last menstrual period. A preterm newborn is defined as an infant delivered before completion of 37 weeks of gestation. The word premature often is used synonymously with preterm, although for any given pregnancy, maturation may lag behind or forge ahead of gestational age. This chapter focuses on the problems of prematurity, which for the most part are those of preterm infants.
Other important terms are low birth weight (LBW), which describes infants with birth weights less than 2,500 g, very low birth weight (VLBW), which describes infants with birth weights less than 1,500 g, and extremely low birth weight (ELBW), which describes infants with birth weights less than 1,000 g. Just as the degree of maturation does not always parallel gestational age, birth weight may be small or large for gestational age. Thus, some preterm infants are not LBW, and some LBW infants are not preterm. Disorders of growth rate and particular problems associated with infants who are small for gestational age are discussed in Chapter 20, Growth and Metabolic Adaptation of the Fetus and Newborn and Chapter 27, Intrauterine Growth Retardation.
EPIDEMIOLOGY
Incidence
The preterm birth rate in the United States was 9.4% in 1981, and over the next two decades rose steadily to a rate of 12.0% in 2002 (Fig. 25.1). The increase in the preterm birth rate can be explained by a failure to combat medical and social conditions associated with preterm birth, as well as an increase in the frequency of multiple births (many due to infertility treatments), an increase in obstetrical interventions, and an increase in ascertainment. The rate of very preterm birth (less than 32 weeks’ gestation) is approximately 2%.
The rate of LBW rose in parallel with the preterm birth rate. The LBW and VLBW rates were 7.8% and 1.45%, respectively, in 2002 (see Fig. 25.1). Significant disparities in these rates exist between races. For example, in 2002, the preterm birth rate for non-Hispanic whites was 11.0%, for Hispanics, 11.6%, and for blacks, 17.5%.
TABLE 25.1. MATERNAL FACTORS PREDISPOSING TO SPONTANEOUS PRETERM LABOR AND DELIVERY | |
---|---|
|
Risk Factors
Most preterm births are spontaneous, due to preterm labor, premature rupture of membranes, or cervical incompetence. Approximately 20% to 30% of preterm births are elective deliveries for maternal or fetal indications. These include maternal preeclampsia, placental abruption, fetal growth restriction, and fetal distress.
Preterm labor is the major cause of preterm delivery. Risk factors for preterm labor include maternal demographic characteristics, maternal lifestyle factors, and medical conditions (Table 25.1). As noted, race is a strong demographic risk factor. Important maternal lifestyle factors include poor prenatal care, poor nutrition, smoking, cocaine use, and possibly psychologic stress. Maternal medical conditions include causes of uterine overdistension such as polyhydramnios and multiple gestation. The twin birth rate was 3.0% in the year 2001, and about half of all twins are born preterm. The rate of VLBW in twin gestations is approximately ten times that in singleton pregnancies. Triplets and higher-order multiple gestations account for approximately 0.2% of births. Ninety percent of triplets are born preterm, at a mean gestational age of 33.5 weeks. Between 1980 and 2000, the rate of twin births increased approximately 50% and that of higher-order multiples by 400%; fortunately, the rate of higher-order multiple births appears to have plateaued.
Preterm delivery has been associated with leukocytosis of the amniotic fluid or chorioamnion, and bacteria have been cultured from the amniotic fluid of women in preterm labor with intact membranes. Genital tract colonization or infection (with such organisms as Ureaplasma urealyticum, Mycoplasma hominis, Trichomonas vaginalis, Gardnerella vaginalis, peptostreptococci, and bacteroides species) thus may be important
causes of preterm labor and delivery. Bacterial vaginosis is present in 10% to 25% of patients in general gynecologic and obstetric clinics, occurs more frequently in black women than in white women, and appears to increase the risk of preterm delivery more than twofold.
causes of preterm labor and delivery. Bacterial vaginosis is present in 10% to 25% of patients in general gynecologic and obstetric clinics, occurs more frequently in black women than in white women, and appears to increase the risk of preterm delivery more than twofold.
TABLE 25.2. RISK OF RECURRENT PRETERM DELIVERY* | ||||||||||||||||||||||||||||||||||||||||||||||||||||||||
---|---|---|---|---|---|---|---|---|---|---|---|---|---|---|---|---|---|---|---|---|---|---|---|---|---|---|---|---|---|---|---|---|---|---|---|---|---|---|---|---|---|---|---|---|---|---|---|---|---|---|---|---|---|---|---|---|
|
Several population-based studies have demonstrated that one of the strongest risk factors for preterm delivery is a history of a previous preterm delivery. Women who deliver at earlier gestational ages are at a greater risk of subsequent preterm birth. For example, in one study, white women whose first birth occurred between 32 and 36 weeks’ gestation had a rate of preterm delivery of 17% in the subsequent pregnancy, whereas those whose first birth occurred before 32 weeks’ gestation had a rate of preterm delivery of 28% in the subsequent pregnancy. The rate of recurrent preterm delivery was higher in blacks than in whites (Table 25.2).
The tendency for preterm delivery to recur may be due to the persistence of medical or lifestyle factors associated with preterm birth. A genetic predisposition to preterm delivery also may be present. Preterm delivery and LBW both have been shown to have a strong familial aggregation in the United States. The risk of preterm delivery is higher in mothers who were born preterm than in mothers who were born at term. A specific genetic locus associated with preterm delivery has not been identified, however.
PATHOGENESIS
Although many risk factors for preterm labor have been identified, the pathophysiologic events leading to preterm delivery are largely unknown. Many findings associated with preterm labor, such as the presence of inflammatory cytokines in the amniotic fluid, fetal fibronectin expression in cervicovaginal mucus, and an elevation in maternal salivary estriol, occur weeks or months before preterm birth, suggesting a chronic pathophysiologic process. Decidual hemorrhage, hormonal changes, mechanical factors, and infection all may be important triggers to preterm labor. Mechanical factors and infection can lead to injury or inflammation at the decidua–chorioamnion interface. This may explain the presence of fetal fibronectin (a basement membrane protein produced by the fetal membranes that helps adhere the placenta and membranes to the decidua) in the cervicovaginal mucus of women who deliver preterm. Bacterial infections, in part through the release of endotoxins and exotoxins, stimulate the production of cytokines and lead to prostaglandin release, neutrophil activation, and the release of other bioactive substances, such as metalloproteases. Prostaglandins stimulate uterine contractions, and metalloproteases both attack the chorioamnionic membranes (causing rupture) and remodel collagen in the cervix (causing softening). The relative role of infection in initiating preterm labor, and the possibility of treating infection and inflammation to prevent preterm delivery, are areas of active investigation. Recent studies showing that progesterone treatment can prevent preterm delivery emphasize the possible role of hormonal imbalances in the pathogenesis of preterm labor.
DIAGNOSIS: GESTATIONAL AGE ASSESSMENT
As noted above, a preterm infant is defined as one delivered before completing 37 weeks of gestation. An accurate assessment of gestational age is not only necessary to diagnose preterm delivery but also influences several aspects of the care of preterm infants. The gestational age affects decisions regarding interventions at the limits of viability, influences caregivers’ expectations of problems related to organ system immaturity, influences pharmacokinetics of certain drugs, affects the anticipated schedule of resolution of a number of problems of prematurity, and influences the timing of hospital discharge.
Obstetric dating is determined by the timing of the last menstrual period and by measurements of fetal size by ultrasound before the twenty-sixth week. After delivery, examination of the newborn is used to confirm obstetric dates or to establish gestational age if obstetric dating is unavailable or unreliable. A number of physical characteristics correlate well with gestational age. For example, the disappearance of the anterior vascular capsule of the lens occurs consistently between 27 and 34 weeks’ gestation. The Dubowitz examination combines a number of neurologic and physical characteristics of newborns and was simplified by Ballard et al. to yield the most commonly used score of neuromuscular and physical maturity. A modified version of the Ballard score (Fig. 25.2) was published in 1991 to improve the accuracy of dating of very preterm neonates. Gestational age assessment by physical examination generally is considered accurate to within 2 weeks, but continues to be less accurate at gestational ages below 28 weeks. For that reason, some neonatologists prefer to use birth weight rather than gestational age when making decisions about the viability and prognosis of extremely preterm neonates.
CLINICAL MANIFESTATIONS AND TREATMENT
Transition from Intrauterine to Extrauterine Life
The resuscitation and stabilization of neonates immediately after delivery are discussed in Chapter 24, The Newborn
Intensive Care Unit. The transition from intrauterine to extrauterine life is more difficult for the preterm infant than the term infant because of the functional immaturity of many organ systems. For example, many preterm neonates have underdeveloped lungs and immature respiratory drive, and they develop respiratory insufficiency immediately after delivery. Transition also is made more difficult by impaired thermoregulation, immunoincompetence, fragility of the germinal matrix of the brain, and renal and hepatic immaturity of the preterm infant.
Intensive Care Unit. The transition from intrauterine to extrauterine life is more difficult for the preterm infant than the term infant because of the functional immaturity of many organ systems. For example, many preterm neonates have underdeveloped lungs and immature respiratory drive, and they develop respiratory insufficiency immediately after delivery. Transition also is made more difficult by impaired thermoregulation, immunoincompetence, fragility of the germinal matrix of the brain, and renal and hepatic immaturity of the preterm infant.
Prenatal Support
Many pregnancies that result in preterm delivery benefit from maternal referral to a high-risk obstetric service. It also is helpful for a neonatologist to meet with the parents before delivery. During this consultation, the neonatologist can become familiar with the details of the obstetric history, let the parents know what to expect after delivery, and answer questions. When delivery is anticipated at the limits of viability, they may discuss the choice between limited or aggressive intervention.
Antenatal interventions used for the treatment of preterm labor include monitoring of fetal well-being, attempts at tocolysis, and pharmacotherapy aimed at minimizing subsequent neonatal morbidity. Antibiotics are used after preterm rupture of membranes to prolong gestation and possibly decrease neonatal morbidity. Antibiotics also are used intrapartum for preterm neonates to try to prevent the vertical transmission of group B streptococcal disease. Antenatal corticosteroid therapy has been proven to improve survival, decrease the incidence and severity of respiratory distress syndrome, and decrease the incidence of intraventricular hemorrhage in preterm infants. Other benefits, and risks, of prenatal corticosteroid therapy (including possible adverse neurodevelopmental affects) continue to be evaluated.
The mode of delivery may affect the outcome of preterm deliveries. Neonates with birth weights between 600 and 1,250 g delivered by cesarean section have a lower incidence of early
intraventricular hemorrhage than those delivered vaginally. Others have noted lower mortality in preterm infants delivered by cesarean section. None of these studies prospectively, randomly assigned mothers to mode of delivery, however, and further evaluation is needed.
intraventricular hemorrhage than those delivered vaginally. Others have noted lower mortality in preterm infants delivered by cesarean section. None of these studies prospectively, randomly assigned mothers to mode of delivery, however, and further evaluation is needed.
Delivery Room Stabilization
The delivery room resuscitation and stabilization required by a preterm newborn depends on a number of factors, including gestational age. Delivery close to a special resuscitation room with cardiorespiratory monitors and pulse oximeters is desirable. Standard resuscitation procedures are followed, although several points should be noted. Infants born before 34 weeks’ gestation do not usually pass meconium, so suctioning of meconium from the trachea rarely is necessary. Because of anticipated functional immaturity, some clinicians opt to immediately intubate ELBW infants to provide respiratory support, without a trial of spontaneous respiration or bag mask ventilatory support. Others intubate preterm infants before the onset of respiratory effort to administer prophylactic surfactant therapy. Finally, the first steps in neonatal resuscitation—the provision of a heat source and drying of the infant to minimize evaporative heat loss—are of exceptional importance in preterm neonates and are discussed in the following section.
Thermoregulation
In utero, the fetus is warmer than the mother and has a body temperature of 37.6°C to 37.8°C. At delivery, the wet, naked infant is exposed to delivery room air temperatures of 22°C to 25°C. Significant heat loss can occur in the first few minutes after birth, through each of the four mechanisms of heat transfer—conduction, convection, radiation, and evaporation. Heat loss after delivery can result in a decrease in body temperature of 1°C to 3°C in just minutes.
Newborns behave as homeotherms and try to maintain body temperature within a narrow range. They respond to environmental cold stress by utilizing subcutaneous fat as an insulating medium, vasoconstricting peripheral vessels to shunt blood away from the body surface, assuming a posture of flexion to minimize skin surface area, and, most importantly, increasing heat production. Unlike adults who shiver to produce heat, neonates increase their metabolic rate to produce heat, an adaptation known as nonshivering thermogenesis. This increase in metabolic rate is achieved primarily through the breakdown of brown fat. Brown adipose tissue differentiates from reticular cells at approximately 26 weeks of gestation. At term, it constitutes 6% of the body weight and 40% of the body fat stores. The lipolysis of brown fat is induced by the release of norepinephrine and thyroid hormones, which are stimulated by cold stress.
Preterm neonates have an impaired response to cold stress. They have less insulating subcutaneous fat, have an impaired ability to peripherally vasoconstrict, have a relatively greater surface area from which to lose heat, and cannot assume a flexion posture to decrease surface area. Finally, their ability to generate heat by nonshivering thermogenesis is markedly impaired. In LBW preterm infants, brown adipose tissue may be markedly diminished or even functionally absent. In addition, VLBW infants may not exhibit a thermogenic response to lowered skin temperatures. Thus, they may be functionally poikilothermic for days after birth, dependent on the thermal environment for the maintenance of optimal body temperature. The ability to thermoregulate improves with advancing postnatal age, and VLBW infants have been demonstrated to have increased subcutaneous body fat deposits and increased thermogenic potential by 3 weeks of age.
Although some decrease in body temperature may facilitate the transition from intrauterine to extrauterine life, excessive heat loss is detrimental to the newborn. Prolonged and maximum heat production can use up substrate and increase metabolic by-products, leading to oxygen debt, metabolic acidosis, and hypoglycemia. Avoiding excessive heat loss has been shown to improve survival. The use of a preheated radiant warmer, immediate drying of the infant, removing wet linen, and increasing the ambient temperature in the delivery room all help to minimize heat loss and are essential components of the resuscitation of a preterm infant.
Close attention to thermoregulation remains exceedingly important during the subsequent hospital course. In the nursery, environmental temperatures are rigorously regulated to maintain a skin temperature of 36.5°C to 37.0°C. Preterm infants in incubators are maintained within the thermoneutral zone, which is defined as the environmental temperature at which the infant maintains a normal body temperature at the lowest level of energy expenditure. Energy expenditure increases at environmental temperatures higher or lower than the thermoneutral zone. Figure 25.3 shows that the thermoneutral zone is higher and narrower for smaller, younger infants.
Problems of Prematurity
Problems experienced by preterm infants are caused primarily by the functional immaturity of multiple organ systems. The degree of functional immaturity, and thus the frequency and severity of neonatal morbidities, is inversely related to gestational age and birth weight. Table 25.3 demonstrates this relationship for selected neonatal morbidities. Several points about the problems of prematurity can be made. First, virtually every organ system is involved to some degree. Second, many of the problems of preterm infants are partially iatrogenic. Almost all the therapies currently used in the neonatal intensive care unit have side effects that can add to the morbidity, and in some cases mortality, of preterm neonates. Perhaps the most obvious example is the role of ventilator-induced barotrauma, volutrauma, and oxygen toxicity in the development of chronic lung disease or bronchopulmonary dysplasia. Other examples are presented in Table 25.4. The care of the preterm neonate requires careful consideration and a balancing of the benefits and risks of every therapeutic intervention.
Finally, most of the problems of prematurity resolve with growth and maturation. (Exceptions, such as long-term growth restriction and neurodevelopmental and pulmonary sequelae, are discussed in subsequent chapters.) The rate of resolution of these problems is extremely variable. For example, indirect hyperbilirubinemia usually resolves within several days of birth, even in the most preterm neonate, whereas apnea of prematurity can persist for weeks or months. The duration of many problems is influenced by both the degree of initial immaturity and complicating factors, including iatrogenic injury. For example, the duration and severity of chronic lung disease is much greater in a 24-week-gestation neonate who, in addition to respiratory distress syndrome, develops pulmonary edema from a patent ductus arteriosus (PDA), pulmonary interstitial emphysema from ventilator-induced barotrauma, or nosocomial sepsis and pneumonia.
Respiratory Function
Impaired respiratory function is usually the most serious problem after the delivery of a preterm newborn. At the limits of viability (22 to 23 weeks’ gestation), the fetal lungs frequently cannot sustain extrauterine life because of inadequate surface area for gas exchange, inadequate thinning of the air–blood barrier, surfactant deficiency, and several other maturational deficiencies. As the lungs grow and mature in the third trimester, these deficiencies disappear. Maternal corticosteroid
treatment before delivery accelerates lung maturation and decreases the incidence of respiratory distress syndrome. Exogenous surfactant therapy corrects surfactant deficiency, but has no effect on the other problems of the premature lung. Surfactant deficiency and neonatal respiratory distress syndrome are discussed in depth in Chapter 42, Respiratory Distress Syndrome, and chronic lung disease or bronchopulmonary dysplasia is discussed in Chapter 50, Bronchopulmonary Dysplasia.
treatment before delivery accelerates lung maturation and decreases the incidence of respiratory distress syndrome. Exogenous surfactant therapy corrects surfactant deficiency, but has no effect on the other problems of the premature lung. Surfactant deficiency and neonatal respiratory distress syndrome are discussed in depth in Chapter 42, Respiratory Distress Syndrome, and chronic lung disease or bronchopulmonary dysplasia is discussed in Chapter 50, Bronchopulmonary Dysplasia.
TABLE 25.3. NEONATAL MORBIDITY BY BIRTH WEIGHT* | ||||||||||||||||||||||||||||||||||||||||
---|---|---|---|---|---|---|---|---|---|---|---|---|---|---|---|---|---|---|---|---|---|---|---|---|---|---|---|---|---|---|---|---|---|---|---|---|---|---|---|---|
|
Immaturity of other respiratory system components besides the lungs frequently causes problems for preterm infants. Preterm infants may have decreased central respiratory drive, particularly if born before 30 to 32 weeks of gestation. In
infants delivered at 24 to 28 weeks’ gestation, apnea frequently persists beyond 38 weeks’ postmenstrual age. Asphyxia, sepsis, intracranial hemorrhage, and metabolic disturbances may exacerbate this problem. (Apnea is discussed in Chapter 49, Apnea.) The preterm neonate’s airway also is less stable, leading to obstructive apnea. Finally, at earlier gestational ages, less ventilatory muscle mass and greater chest wall compliance is present. Thus, even with adequate central ventilatory drive and a patent airway, VLBW infants may not be able to perform the work necessary for effective ventilation. Diseases that decrease lung compliance, such as respiratory distress syndrome, compound this problem.
infants delivered at 24 to 28 weeks’ gestation, apnea frequently persists beyond 38 weeks’ postmenstrual age. Asphyxia, sepsis, intracranial hemorrhage, and metabolic disturbances may exacerbate this problem. (Apnea is discussed in Chapter 49, Apnea.) The preterm neonate’s airway also is less stable, leading to obstructive apnea. Finally, at earlier gestational ages, less ventilatory muscle mass and greater chest wall compliance is present. Thus, even with adequate central ventilatory drive and a patent airway, VLBW infants may not be able to perform the work necessary for effective ventilation. Diseases that decrease lung compliance, such as respiratory distress syndrome, compound this problem.
TABLE 25.4. EXAMPLES OF SECONDARY DISORDERS OF PRETERM INFANTS THAT RESULT FROM THE TREATMENT OF PRIMARY DISORDERS | ||||||||||||||||||||
---|---|---|---|---|---|---|---|---|---|---|---|---|---|---|---|---|---|---|---|---|
|
Cardiovascular Function
Unlike the lungs, which are not involved in gas exchange in utero, the fetal heart must function throughout most of gestation. Problems of the cardiovascular system in the preterm neonate are thus rarely caused by functional immaturity. Rather, cardiac insufficiency usually is caused by the persistence of a PDA, myocardial dysfunction secondary to other acute problems such as sepsis or asphyxia, or congenital heart disease.
In utero, the ductus arteriosus is patent, under the influence of prostaglandins and the low oxygen tension of fetal blood. It carries 55% to 60% of the combined cardiac output and allows the majority of the right ventricular output to bypass the pulmonary circulation by shunting blood from the pulmonary artery to the descending aorta. After birth, the plasma oxygen tension increases sharply, effecting a reactive vasoconstriction of the ductus arteriosus. In addition, because the lung is a major site of prostaglandin catabolism, postnatal increases in pulmonary blood flow result in a higher rate of prostaglandin degradation.
The ductus arteriosus is functionally closed within 10 to 15 hours of birth in most term neonates, and by 2 days in almost all term neonates. Closure of the ductus arteriosus is delayed in preterm neonates, possibly because of an impaired vasoconstrictor response to the increase in oxygen tension. The incidence of a persistently PDA depends on gestational age and postnatal age. In a large national collaborative study, a symptomatic PDA was noted during hospitalization in 42%, 21%, and 7% of neonates with birth weights between 500 and 999 g, 1,000 and 1,499 g, and 1,500 and 1,750 g, respectively. Sepsis probably increases the risk of a PDA.
As pulmonary vascular resistance decreases, a PDA may result in significant shunting of blood from the aorta to the pulmonary artery, with subsequent myocardial stress, pulmonary congestion, and systemic underperfusion. A PDA may thus worsen the course of respiratory distress syndrome, increase the incidence of bronchopulmonary dysplasia and—through “steal” of systemic blood flow—increase the risk of necrotizing enterocolitis, cerebral ischemia, and intraventricular hemorrhage. Renal perfusion, glomerular filtration rate, and free water clearance also may be reduced. Progressive heart failure and diminished effective cardiac output can be life-threatening.
Neonates with a PDA typically have a systolic or continuous murmur, hyperactive precordium, increased pulse pressure, and bounding pulses. If heart failure ensues, tachycardia, hepatomegaly, decreased urinary output, tachypnea, and worsening respiratory distress may occur. Chest radiography may reveal cardiomegaly in addition to pulmonary plethora. A metabolic acidosis may develop.
Some patients respond to fluid restriction or diuretic therapy. Pharmacologic closure with indomethacin, and rarely ibuprofen (inhibitors of prostaglandin synthesis), is attempted in most cases. If pharmacologic closure fails or is contraindicated, a PDA may be closed surgically. The closure of a PDA in a 590-g neonate using a video-assisted thoracoscopic approach also has been reported.
In addition to acute processes such as sepsis, asphyxia, or PDA, a number of chronic disorders can impair myocardial function in the growing preterm neonate. Hyperinsulinism, chronic corticosteroid therapy, and secondary hypertension may lead to myocardial hypertrophy. Chronic hypoxemic episodes, particularly in patients with severe bronchopulmonary dysplasia, can lead to right-sided heart failure.
Hypotension and hypertension are relatively common problems in the preterm neonate, and hemodynamic instability may contribute to the development of intraventricular hemorrhage or periventricular leukomalacia.
Fluids and Electrolytes
After birth, neonates lose body weight because of a loss of body water, primarily from the extracellular compartment. This physiologic weight loss represents an isotonic contraction of body fluids, is associated with a diuretic phase, and is part of the normal adaptation to extrauterine life. VLBW infants have a higher body-water content and a higher ratio of extracellular to intracellular water. Because of their different body composition, neonates with lower birth weights lose a greater proportion of their body weight after birth. For example, the mean maximum postnatal weight loss is approximately 10% of birth weight for infants weighing 1,000 to 1,500 g compared to 5% to 7% for those weighing 2,500 g. The administration of excessive fluids to prevent this contraction has been associated with a higher incidence of symptomatic PDA, necrotizing enterocolitis, and bronchopulmonary dysplasia.
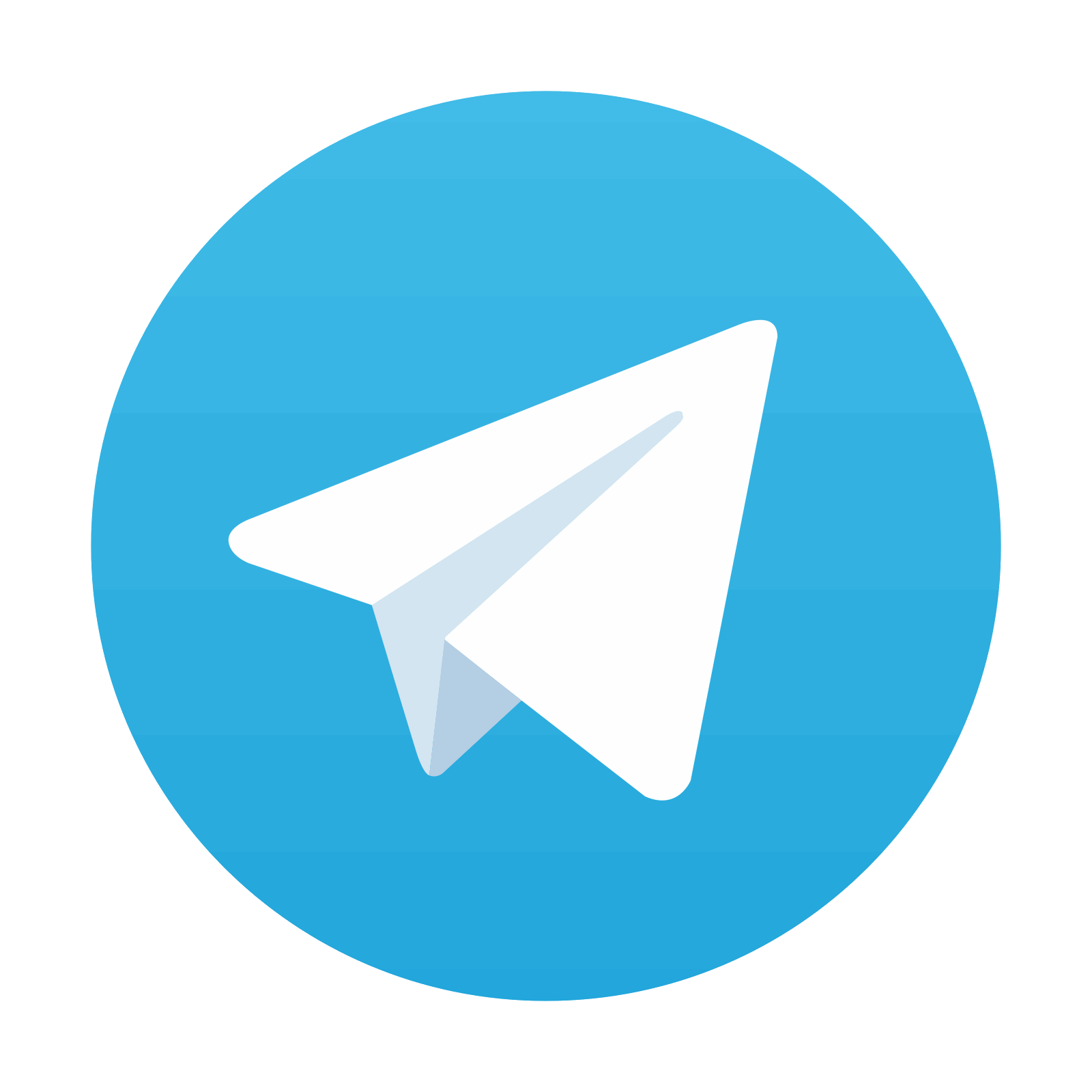
Stay updated, free articles. Join our Telegram channel

Full access? Get Clinical Tree
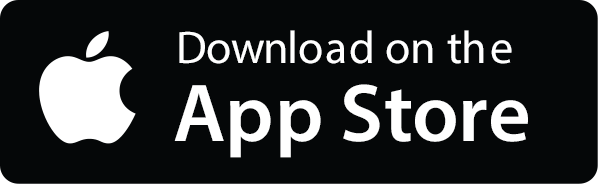
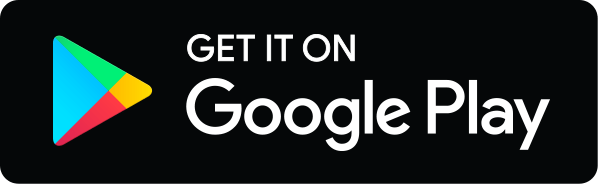
