DEVELOPMENTAL ABNORMALITIES
The initial manifestation of eye development in the embryo occurs during the third week of gestation when grooves appear in the cranial aspect of the neural tube; these grooves are termed the optic sulci. As the neural folds fuse during the fourth week, the optic sulci bulge outward from the neural tube toward the surface ectoderm; at this point they are referred to as the optic vesicles. As the optic vesicles extend outward, they remain connected to the developing diencephalon by the hollow optic stalks, which continue to elongate until the optic vesicles contact the surface ectoderm. During this process, neural crest cells from the dorsolateral portion of the neural tube migrate ventrally to surround the developing eye. The neural crest cells differentiate into the skeletal and connective tissue components of the orbit, lids, and conjunctiva. The neural crest cells also give rise to the middle ear bones, teeth, meninges, and endocrine gland components. This common developmental pathway accounts for the association of some developmental ocular lesions with craniofacial, dental, spinal cord, and ear anomalies.
During the fifth week of gestation, the optic vesicles invaginate inferiorly to form the optic cups. Each optic cup is composed of inner and outer layers that are separated by a potential space. A groove termed the choroidal fissure forms along the inferonasal aspect of each optic cup and stalk. The choroidal fissure serves as the pathway for mesenchyme of neural crest cell origin to migrate into the optic cup and stalk. The hyaloid vessels that supply to the developing eye also course through the choroidal fissure. Closure of the choroidal fissure begins in the central aspect of the optic cup during the fifth gestational week and proceeds anteriorly and posteriorly until complete by the end of the seventh week. The optic cups contribute to formation of parts of the retinas, ciliary bodies, and irises.
The optic vesicles induce the overlying surface ectoderm to form the lens placodes. The lens placodes differentiate into the lens pits, which invaginate to form the lens cups and subsequently the lens vesicles. The lens vesicles are hollow spherical structures that are lined by cuboidal epithelium. The lens vesicles eventually detach from the surface ectoderm and come to lie anterior to the developing optic cups. The lens vesicles induce the overlying surface ectoderm to form the corneal epithelium.
Microphthalmos (microphthalmia) refers to an abnormally small globe, either due to congenital underdevelopment or acquired diminution in size. Simple congenital microphthalmos consists of a small globe without other orbital abnormalities. The deformity is usually unilateral. Congenital complex microphthalmos is most often bilateral and tends to be associated with colobomas and intracranial anomalies.
The most common causes of microphthalmos include posterior coloboma, microphthalmos with cyst, persistent hyperplastic primary vitreous, phthisis bulbi, retinopathy of prematurity, and congenital toxoplasmosis. Other potential mechanisms include trauma, congenital syphilis, viral infection, and irradiation. Associations with microphthalmos include Sturge-Weber syndrome, hypomelanosis of Ito, Goldenhar syndrome, Proteus syndrome, Aicardi syndrome, congenital glaucoma, fetal alcohol syndrome, epidermal nevus syndrome, CHARGE syndrome, Gorlin syndrome, Rubinstein-Taybi syndrome, Fanconi syndrome, Diamond-Blackfan syndrome, and various chromosomal anomalies. Microphthalmos occurs in approximately 1 in 2000 livebirths.
Anophthalmos is defined as the complete absence of the globe or the presence of a vestigial eye. There are primary, secondary, and degenerative types. Primary anophthalmos is often bilateral, and is due to lack of development of the optic primordia. Degenerative anophthalmos occurs when the vesicle forms but later degenerates. Secondary anophthalmos is due to lack of development of the anterior portion of the neural tube; this is a very rare, fatal anomaly. Anophthalmos occurs with an incidence of approximately 0.2 per 1000 births. Anophthalmos is associated with trisomy 13, congenital rubella, maternal vitamin A deficiency, maternal thalidomide ingestion, and a variety of syndromes.
Imaging studies of children with microphthalmia or anophthalmia serve to document the orbital anatomy and detect associated intracranial or craniofacial anomalies. The spectrum of ocular pathology ranges from an otherwise normal-appearing small globe to complete absence of the globe and optic nerve (Figure 27-1). In patients with bilateral anophthalmia, the optic chiasm is small or absent, the optic tracts are rudimentary, and there may be manifestations of gliosis in the lateral geniculate nuclei. Dystrophic calcification sometimes occurs in rudimentary ocular tissue. The extraocular muscles are present, even in patients with true anophthalmia. Underdevelopment of the bony orbit parallels the severity of the ocular anomaly. In patients with acquired microphthalmia, the globe often appears small and irregular and may contain dystrophic calcifications.
Cerebro-oculo-nasal syndrome refers to the association of anophthalmia with central nervous system (CNS) anomalies and anomalous nasal development. Hydrocephalus and dysgenesis of the corpus callosum are common in these children. Most have neurodevelopmental abnormalities. In addition to the nasal malformation, these children may have abnormal eyebrows and eyelashes, as well as anomalies of the external ears.1,2
Coloboma is a congenital ocular defect that is due to incomplete closure of the primitive embryonic choroidal fissure. The defect can occur anywhere from the iris to the posterior aspect of the optic nerve sheath, thereby involving the optic disc, retina, or uvea. The prevalence of coloboma is approximately 1 in 1000 births. Coloboma can be isolated (most common), inherited (bilateral), associated with microphthalmia, or associated with brain anomalies such as dysgenesis of the corpus callosum. Coloboma is part of the CHARGE association, which includes coloboma, heart defects, atresia of the choanae, retarded growth and development, genital anomalies, and ear anomalies. Coloboma can also occur in association with Meckel syndrome, Warburg syndrome, Aicardi syndrome, Goltz focal dermal dysplasia, basal encephalocele, and midline facial clefts. Visual acuity in patients with coloboma ranges from normal to total blindness, largely determined by the size and location of the lesion.
Colobomas range in size from a very small defect to a large lesion that involves the entire length of the choroidal fissure. A retinochoroidal coloboma arises from the wall of the globe, and is characterized by thinning and outward bulging of the sclera along the inferomedial quadrant. These patients are at risk for retinal detachment, which occurs in approximately 10% of affected children. Cross-sectional imaging with CT or MR shows a retinochoroidal coloboma as a fluid-filled outpouching of the globe, with thinning and eversion of the sclera. In some patients, a cyst projects into the retrobulbar space (Figure 27-2). A defect in the sclera should be visible at some point along the margin of the cyst. The eye is usually small in patients with a retinochoroidal coloboma, and the optic nerve may also be small.3,4
Pathology | Radiology |
---|---|
Defective closure of choroidal fissure | Extraocular cyst |
Communication with vitreous | |
± microphthalmos |
In some patients with coloboma, markedly ectatic sclera expands into the orbit and forms a large cyst that communicates with the vitreous of a small dysplastic globe; this is termed microphthalmos with cyst. Developmental abnormalities of the contralateral eye are common in children with microphthalmos with cyst. Imaging with CT or MR shows a very small dysplastic globe, with a large portion of the orbit occupied by a fluid-filled mass that is adjacent to, and causes distortion of, the globe (Figure 27-3). The contents of the cyst are homogeneous and have CT attenuation values and MR signal intensities similar to those of normal vitreous. In some patients, the cyst contents are slightly hyperintense relative to vitreous on T2-weighted MR sequences. Contrast enhancement does not occur. There is a peripheral capsule comprised of thin sclera. Images should be inspected for communication of the cyst with the vitreous cavity of the dysplastic globe. In some patients, however, the communication is too small for visualization on imaging studies.5
An additional subtype of coloboma is the morning glory disc anomaly, which refers to a small coloboma of the optic nerve head in association with peripapillary anomalous retinal vessels, pigmentary changes, and gliosis. Ophthalmoscopic examination shows the optic disc to be enlarged, excavated, and funnel-shaped, with an elevated rim. Morning glory anomaly is typically unilateral. Females are affected twice as often as males. Various associated congenital malformations occur with an increased incidence in these children, including periorbital hemangioma, midline craniofacial defects (e.g., cephalocele), midline brain anomalies (e.g., corpus callosum dysgenesis), and intracranial vascular anomalies (e.g., moyamoya). Concomitant colobomas of the iris, ciliary body, or retina are lacking in patients with morning glory disc anomaly.
Morning glory disc anomaly is readily detected with ophthalmoscopic examinations. The primary role for imaging studies in these patients is to assess for an associated anomaly. The funnel-shaped or rounded coloboma of the optic nerve head is visible on high-resolution CT and MR images (Figure 27-4). Occasionally, there are small calcifications in the dysplastic retinochoroidal tissue at the margins of the defect. Dilation of the optic nerve sheath is sometimes present. The retrobulbar portions of the optic nerves usually are normal. The involved globe may be small or normal in size. Patients with morning glory anomaly are at risk for retinal detachment and subretinal exudate.6,7
Buphthalmos refers to enlargement of the globe due to elevated intraocular pressure. The pathogenesis usually involves obstruction of the canal of Schlemm. The canal of Schlemm is located between the cornea and the iris. Patency of this structure is essential for appropriate resorption of the aqueous humor. Buphthalmos may occur as an isolated lesion or in association with neurofibromatosis type 1 (NF-1), Sturge-Weber syndrome, homocystinuria, aniridia, Lowe syndrome, or ocular mesodermal dysplasia. In patients with NF-1, aberrant mesodermal tissue obstructs the canal of Schlemm. CT and MR of patients with buphthalmos show diffuse enlargement of the globe (Figure 27-5). The sclera is thin and the ventral surface of the lens may be flattened.8
Figure 27–5
Buphthalmos.
A contrast-enhanced T1-weighted MR image of a child with NF-1 shows an enlarged left globe. There is an enhancing infiltrative plexiform neurofibroma in the left orbit, periorbital region, and infratemporal fossa. The bony walls of the orbit are expanded. Exophthalmos is due to the enlarged globe and globe displacement by the neurofibroma.

A staphyloma is a type of focal buphthalmos, in which there is a localized bulge in the posterior sclera. This lesion sometimes occurs in association with axial myopia; in these patients, the lesion most often is located on the temporal side of the optic disc. Staphyloma can also occur due to trauma, infection, or glaucoma. Cross-sectional imaging studies show a focal bulge of the sclera. The sclera is abnormally thin at the site. Unless there is choroidal hemorrhage and retinal detachment, the lesion contains fluid that has identical imaging characteristics to those of normal vitreous. Table 27-1 lists considerations in the differential diagnosis of enlargement of the globe.9
Condition | Imaging features |
---|---|
Buphthalmos | Uniformly enlarged globe |
Axial myopia | Elongation of the globe; scleral thinning |
Staphyloma | Focal thinning and bulging of the sclera |
Connective tissue disorder | Irregular globe contour; scleral thinning |
Proteus syndrome | Enlarged globe, periorbital exostoses, epibulbar tumors |
Intraocular mass | Soft tissue in vitreous cavity |
Peters anomaly is a rare congenital malformation of the anterior segment of the eye. It is characterized by a central corneal opacity in conjunction with subjacent defects in the posterior corneal stroma, Descemet membrane, and corneal endothelium. Bilateral ocular involvement is present in approximately 80% of patients. There is considerable variation between patients with regard to the severity of the ocular deformities. Congenital glaucoma occurs in 50% to 70% of patients with Peters anomaly. The major importance of Peters anomaly from a diagnostic imaging standpoint is the association of this lesion with a wide array of congenital ocular and systemic abnormalities: cataracts, glaucoma, microcornea, buphthalmos, aniridia, coloboma ± microphthalmia, short stature, cleft lip/palate, and anomalies of the CNS, genitourinary tract, and cardiovascular system. Systemic malformations are most common in individuals with bilateral ocular involvement.
Peters-plus syndrome refers to Peters anomaly in association with cleft lip/palate, short limbs, cognitive dysfunction, and ear anomalies. Peters-plus syndrome is an autosomal recessive congenital disorder of glycosylation in which there is inactivation of a β-1,3-glucosyltransferase.10,11
Axial myopia refers to enlargement of the globe along the anteroposterior axis. Axial myopia is the most common cause of macrophthalmia. This lesion is usually idiopathic; it can also occur in association with Graves disease or orbital infection. Axial myopia can be unilateral or bilateral. Because there is convergence of light anterior to the retina, the affected eye has impaired visual acuity. Axial myopia is demonstrated on cross-sectional imaging studies as an abnormal oval configuration of the globe, with elongation in the anteroposterior dimension. Scleral thinning may be present.9,12
Persistent hyperplastic primary vitreous is a developmental anomaly caused by failure of involution of the primary vitreous and fetal hyaloid vasculature. Hyperplasia of embryonic connective tissue occurs within the globe. There is no gender predilection for this disorder. The sporadic form is usually unilateral. Persistent hyperplastic primary vitreous occasionally occurs in conjunction with multisystem diseases, such as Walker-Warburg syndrome, primary vitreoretinal dysplasia, and Norrie disease. The syndromic form is often bilateral.
Children with primary hyperplastic primary vitreous usually present with leukocoria. The globe is small. Symptoms related to vitreous hemorrhage or lens opacification may occur. Funduscopic examination shows a white or pink vascularized membrane behind the lens. Although the lens is clear at birth, it eventually becomes opaque. Persistent hyperplastic primary vitreous is the second most common cause of leukocoria in children, after retinoblastoma (Table 27-2). The natural course of the disorder is progression to glaucoma and eventual buphthalmos or phthisis. If the eye is not severely microphthalmic, surgical extraction of the retrolental membrane and vascularized tissues preserves limited vision in selected cases. Surgical intervention can also help prevent progression to glaucoma and spontaneous hemorrhage.
Condition | Imaging features | Bilateral | Globe size | Ca++ |
---|---|---|---|---|
Retinoblastoma | Enhancing intraocular mass | No | Normal or ↑ | ++ |
Persistent hyperplastic primary vitreous | Subretinal fluid; intravitreal foci of enhancement | ± | ↓ | No |
Coats disease | Nonenhancing subretinal exudate, high attenuation on CT, high T1 signal on MR | 10% | Normal or↓ | No |
Retinopathy of prematurity | Early: subretinal fluid Late: fibrosis | Yes | Early: normal Late: ± ↓ | Early: No Late: ± |
Toxocara granuloma | Subretinal fluid, high attenuation on CT, high T1 signal on MR Granuloma | No | Early: normal Late: ± ↓ | ± |
Norrie disease | Early: intraocular mass, subretinal fluid Late: phthisis bulbi | Yes | Early: normal Late: ↓ | No |
Familial exudative vitreoretinopathy | ± Retinal detachment | Yes | Normal | No |
Persistent hyperplastic primary vitreous is characterized by the presence of remnants of the primary vitreous and lenticular branches of the primitive hyaloid artery along the course of the Cloquet canal. Histologically, there is a fibrovascular plaque of tissue that is adherent to the posterior surface of the lens. This tissue may undergo proliferation and can extend to the ciliary processes. Other tissue types can be present within the lesion, including smooth muscle, cartilage, and fat. The dysplastic vessels are friable, resulting in a propensity for intravitreal hemorrhage.
The imaging diagnosis of persistent hyperplastic primary vitreous can be achieved with CT, MR, or ultrasound. These studies show a small globe, which is an important characterizing feature. On CT, there is usually generalized increased attenuation of the vitreous chamber; however, the majority of the vitreous may have normal attenuation values in patients with mild disease. Calcification does not occur. In some cases, there are small intravitreal high attenuation masses that represent remnants of the embryonic hyaloid vascular system; these masses often enhance with IV contrast. The Cloquet canal may be demonstrated as a fine linear high attenuation structure extending from the retina to the posterior surface of the lens. A linear or triangular (apex posterior) band of enhancing tissue along the course of the Cloquet canal represents the hyaloid artery and embryonic fibrovascular tissue. The lens may be irregular and small, and the anterior chamber is often shallow. Retinal detachment is common; the associated subretinal fluid is often hyperintense on T1-weighted MR images and does not enhance with contrast (Figure 27-6). Layering within the subretinal fluid may occur.
Figure 27–6
Persistent hyperplastic primary vitreous.
A. Contrast-enhanced CT shows increased attenuation in a small right globe. There is triangular enhancing soft tissue extending through the central aspect of the vitreous chamber. B. Subretinal fluid is hyperintense on this T1-weighted MR image. Fibrovascular tissue between the displaced retinal leaves has a triangular shape. The anterior chamber is small.

Pathology | Radiology |
---|---|
Fibrovascular plaque posterior to lens |
Linear or triangular retrolental mass Enhancement; central vessel |
Dysplastic ocular development | Microphthalmos |
Hemorrhage | Subretinal fluid |
MR of persistent hyperplastic primary vitreous shows the serosanguineous fluid within the subretinal or subhyaloid spaces to have relatively high signal intensity on both T1-weighted and T2-weighted images, due to the high protein content. Fluid levels may be present. As with other imaging studies, characteristic MR features are microphthalmos and a linear or triangular band of tissue that extends from the retrolental region to the optic nerve head. The optic nerve within the involved orbit is normal or slightly small.
The typical sonographic appearance of persistent hyperplastic primary vitreous consists of a linear or triangular band of echogenic tissue at the midline of the globe, often sandwiched between bilobed hypoechoic subretinal fluid accumulations. Doppler examination may confirm the diagnosis by demonstrating flow within hyaloid vessels that course from the retrolental region toward the optic nerve head.
Norrie disease is an x-linked recessive genetic disorder caused by mutations in the NDP gene on Xp11.4. The encoded protein norrin is essential for appropriate specialization of retinal cells and for the establishment of blood supply to the retina and inner ear. Affected infants have leukocoria. Ophthalmoscopy demonstrates a fibrovascular mass within the vitreous. Progression over the next several years of childhood leads to evolving retinal detachment, glaucoma, cataracts, degeneration of the iris, and shrinkage of the globe. Eventually, phthisis bulbi occurs. Potential nonocular pathology in these patients includes developmental delay and slowly progressive hearing loss.13
In the early phase of Norrie disease, imaging studies may show retinal detachment, vitreoretinal hemorrhage, and a retrolental mass. Older children have bilateral microphthalmia. On CT, there is elevated attenuation within the vitreous cavities. The lenses are hyperattenuating. There are no ocular calcifications. MR may demonstrate hyperintense vitreous on T1-weighted sequences. The optic nerves are small.
Coats disease (congenital retinal telangiectasia) is a congenital idiopathic vascular anomaly of the retina. The characteristic features of this disorder are telangiectatic and aneurysmal retinal vessels, progressive accumulation of intraretinal and subretinal exudates, and the eventual development of massive exudative retinal detachment. Approximately 75% of individuals with Coats disease are male. Approximately 90% of cases are unilateral. True Coats disease is isolated and sporadic. Abnormalities of the retinal vasculature similar to those of Coats disease can occur in some children with multisystem abnormalities such as Turner syndrome, epidermal nevus syndrome, facio-scapulo-humeral muscular dystrophy, pericentric inversion of chromosome 3, and deletion mutations in chromosome 13.
Although a congenital lesion, the clinical presentation of Coats disease does not usually occur until there is retinal detachment. The peak age at diagnosis is 5 years. Most often, Coats disease is detected clinically due to leukocoria or strabismus; diminished visual acuity is also a common presenting complaint. Funduscopy shows retinal telangiectasis, microaneurysms, saccular outpouchings of retinal venules, and arteriovenous shunts. The temporal quadrants are most often affected in the early stages of the disease. With progression, yellow intraretinal and subretinal exudation is visible.14
The pathophysiology of Coats disease involves abnormal permeability of the vascular endothelial cells in the involved portion of the retina. This results in breakdown of the blood-retinal barrier and leakage of blood components into the retinal tissue and subretinal space. Among the materials that accumulate are fibrin, cholesterol, and lipid-laden macrophages. This exudate eventually causes retinal detachment and loss of vision. Coats disease nearly always follows a progressive clinical course. Coats disease can be classified into 5 stages based on the severity of retinal disease.15 With stage 1, there are focal exudates. Extensive intraretinal exudation is present in stage 2. With stage 3, there is partial exudative retinal detachment. Total retinal detachment is present in stage 4. Stage 5 is characterized by complications due to chronic retinal detachment.
The treatment of Coats disease consists of surgical photocoagulation or transscleral cryopexy of the abnormal retinal vessels. Effective stabilization is possible for some patients when photocoagulation is performed during the early phases of the disease. Retinal detachment surgery is sometimes useful for advanced disease.
Diagnostic imaging of children with suspected Coats disease helps to establish the severity of the disease and, more importantly, aids in the differentiation from potential mimicking conditions such as retinoblastoma and persistent hyperplastic primary vitreous. The clinical diagnosis of Coats disease may be difficult or impossible when there is retinal detachment at the time of presentation. In the early stages of Coats disease, diagnostic imaging studies of the orbits are usually normal. Retinal detachment is the major finding with progressive disease.
CT of patients with advanced Coats disease shows diffuse high attenuation throughout the vitreous or a wing-shaped retinal detachment (Figure 27-7). When there is total retinal detachment, the boundaries of the retina may not be discernible on CT. The subretinal fluid is homogeneous and produces higher attenuation values than normal vitreous. The subretinal material does not enhance with contrast. Linear enhancement is typically visible at the anterior margin of the subretinal exudate, due to the presence of telangiectatic vessels within the thickened retina. The globe is usually normal in size or slightly small. Imaging with CT serves to exclude the presence of calcifications; this is important in the differentiation from retinoblastoma, which is often calcified. Rarely, there are submacular calcifications due to metaplastic changes in the retinal epithelium in patients with advanced Coats disease.16
Pathology | Radiology |
---|---|
Hyperpermeability of retinal vascular endothelial cells Subretinal exudate | Retinal detachment Subretinal fluid |
Telangiectatic vessels within thickened retina | ↑ Retinal enhancement |
On MR, the subretinal fluid in patients with Coats disease is hyperintense on both T1-weighted and T2-weighted images, due to the high lipid and protein content. The subretinal exudate tends to be relatively homogeneous early in the course of the disease, and becomes heterogeneous with chronicity. The heterogeneity can be ascribed to fibrosis and areas of organized hemorrhage. When present, a heterogeneous appearance aids in the distinction from the more homogeneous subretinal fluid that occurs in patients with retinoblastoma, persistent hyperplastic primary vitreous, and retinopathy of prematurity. As with CT, MR frequently shows enhancement in the thickened retina at the interface between the exudate and the vitreous. The lack of a focal enhancing mass serves to differentiate this lesion from retinoblastoma.16,17
Choroidal venous angiomas (small-vessel venous malformations of the choroid) are present in at least one-third of patients with Sturge-Weber syndrome. The ocular lesion is usually unilateral and ipsilateral to a facial port-wine stain (cutaneous capillary malformation). The choroidal venous lesions that occur in patients with Sturge-Weber syndrome are usually flat and diffuse. Many patients with choroidal venous angiomas are asymptomatic during childhood. Additional ocular pathology associated with Sturge-Weber syndrome includes glaucoma and buphthalmos. Glaucoma in these patients often develops during infancy. The pathophysiology may involve alteration of the anterior chamber drainage angle or elevation of episcleral venous pressure due to a conjunctival/episcleral capillary malformation.
Choroidal venous angiomas are often demonstrable with MRI. There is thickening of the choroid, which is the layer along the inner margin of the hypointense peripheral sclera. The thickened choroid often has a crescentic shape and enhances prominently with IV contrast. A subretinal effusion can develop in association with a choroidal angioma. This contains proteinaceous fluid that is moderately hyperintense on T1-weighted images (Figure 27-8). Imaging studies of Sturge-Weber syndrome patients with buphthalmos due to glaucoma show an enlarged globe, often with an elongated shape (Figure 27-9).
Figure 27–8
Choroidal venous angioma.
A. An axial fat-suppressed T1-weighed image of a 7-year-old child with a left facial port-wine stain shows high signal intensity subretinal fluid collections in the left globe. The choroidal angioma is a layer of moderate signal intensity tissue (arrow) between the fluid and the hypointense sclera. B. There is intense enhancement of the angioma (arrow) on this fat-suppressed T1-weighted image obtained after IV gadolinium administration. The subretinal fluid does not enhance.

Retinopathy of prematurity (retrolental fibroplasia) is a vascular proliferative disorder that affects premature infants with low birth weights. This disorder may lead to blindness from retinal detachment, macular folds, refractive amblyopia, or strabismic amblyopia. Approximately 80% of newborns with birth weights of less than 1 kg develop retinopathy of prematurity of varying severity, and nearly 10% of these infants go on to suffer vision-threatening disease. About half of infants with birth weights between 1 and 1.25 kg develop this disorder, but only 2% of these children suffer significant visual compromise. A 2004 study of retinopathy of prematurity in the state of New York showed an overall prevalence among all newborn infants over a 5-year period of 0.2%. The prevalence among infants with a birth weight less than 1200 g and an initial hospital length-of-stay more than 28 days was 27%.18
Retinopathy of prematurity results in some degree of permanent visual loss in approximately 1300 children each year in the United States and causes severe visual loss in approximately 500 of these children. Optimization of the clinical outcome of retinopathy of prematurity requires early detection and treatment. Therefore, the American Academy of Pediatrics, the American Academy of Ophthalmology, and the American Association for Pediatric Ophthalmology and Strabismus recommend a screening examination by an experienced ophthalmologist for all infants with a birth weight less than 1500 g or with a gestational age less than 28 weeks, as well as for selected infants between 1500-and 2000-g birth weight who are believed to be at high risk by their attending neonatologist.19–21
The pathophysiology of retinopathy of prematurity is incompletely elucidated. Potential factors include prolonged oxygen therapy, increased ambient light, and inadequate levels of vitamin E. Oxygen induces aberrant physiological responses in various tissues that can be damaging in premature infants. Vasoconstriction in the retina is an early response to oxygen that can lead to vasoobliteration, neovascularization, and retinal traction. The temporal portion of the retina is most susceptible to retinopathy of prematurity, due to the still-developing vasculature in this region in premature infants. The earliest ophthalmoscopic finding is the development of a demarcation line between the vascular and avascular portions of the retina. Abnormally branched and tortuous vessels are visible adjacent to the avascular portion of the retina. With progression, abnormal retinal vessels may extend into the vitreous, and migration of myoblasts into the vitreous eventually leads to formation of a fibrovascular mass. This can progress to partial retinal detachment or complete retinal detachment. In most affected children, progression of this process is incomplete.
The diagnostic imaging features of retinopathy of prematurity depend on the stage and severity of disease. In the chronic phase, a noncalcified, retrolental high attenuation mass may be visible on CT, often associated with retinal detachment. Approximately three-quarters of retinal detachments in these children resolve spontaneously. Scarring of the vitreous with advanced disease can lead to microphthalmia. Children with chronic retinopathy of prematurity sometimes have ocular calcifications that are visible on CT. During the early stages of retinopathy of prematurity, MRI may show subretinal fluid that is hyperintense on T2-weighted images and hypointense on contrast-enhanced T1-weighted images. Persistence of the embryonic hyaloid vascular system occurs in some children with retinopathy of prematurity.
The orbits are involved with various types of cephalocele (see Chapter 26). Hypertelorism is common in children with frontoethmoidal or sphenoidal (basal) cephaloceles. Defects in the orbital wall(s) sometimes occur. Rarely, a cephalocele bulges into the orbit via a defect in the roof. Children with sphenoidal cephalocele sometimes have dysplastic optic nerves. A rare type of orbital cephalocele can occur in children with NF-1, with expansion of middle cranial fossa structures into the posterior orbit via sphenoid wing dysplasia. Imaging with CT or MR allows demonstration of the osseous defect of a cephalocele, the size and location of the resultant herniated meningeal sac, the presence or absence of brain within the sac, and the effects of the cephalocele on the intraorbital structures.
Optic nerve hypoplasia is a developmental abnormality that is among the more common causes of visual impairment in children. Brain anomalies are present in at least half of children with bilateral optic nerve hypoplasia. Potential CNS abnormalities in these patients include absence of the septum pellucidum, ectopic neurohypophysis, cerebral migration anomalies, schizencephaly, gray matter heterotopias, cephalocele, and manifestations of intrauterine brain injury. Children with absence of the septum pellucidum as the only associated intracranial abnormality typically have normal neurodevelopmental and endocrinological functions. Posterior pituitary ectopia and abnormalities of the cerebral hemispheres in children with optic nerve hypoplasia usually are accompanied by pituitary hormone deficiency and neurodevelopmental deficits. Clinical evidence of hypothalamic-pituitary dysfunction is present in approximately 60% of patients with bilateral optic nerve hypoplasia. Individuals with unilateral optic nerve hypoplasia sometimes have coloboma, microphthalmia, retinal vascular malformation, aniridia, blepharophimosis, or ocular motor nerve palsy. The severity of visual impairment with optic nerve hypoplasia varies substantially between patients.22–25
Septooptic dysplasia (de Morsier syndrome) refers to bilateral optic nerve hypoplasia in conjunction with agenesis or hypoplasia of the septum pellucidum. The optic nerves, optic chiasm, and hypothalamic infundibulum are hypoplastic in patients with septooptic dysplasia (see Figure 14-30 in Chapter 14). The pituitary gland is frequently hypoplastic, and about two-thirds of patients suffer hypothalamic-pituitary dysfunction (most often deficiencies of growth hormone and thyrotropin). Other midline intracranial anomalies can also occur, and some patients have cleft palate. Septooptic dysplasia has a multifactorial etiology; hypothesized mechanisms include an insult during pregnancy (e.g., viral infection, gestational diabetes), vascular disruption, and genetic alteration (HESX1 gene mutations). Potential clinical findings include strabismus, nystagmus, decreased visual acuity, endocrine dysfunction, mental retardation, cerebral palsy, developmental delay, and delayed growth.26,27
Although a variety of developmental mechanisms likely account for the findings of septooptic dysplasia, this disorder is often classified into 3 subsets according to the embryogenesis and the neuropathological findings. (1) When there is an associated malformation of cortical development, the lesion can be termed septooptic dysplasia-plus. The potential cortical lesions with this type of septooptic dysplasia include schizencephaly (most common) and gray matter heterotopias (see Figure 14-31 in Chapter 14). There is partial formation of the septum pellucidum in some of these patients. (2) The second type of septooptic dysplasia, which accounts for about half of the affected patients, may represent a mild form of holoprosencephaly. The septum pellucidum is usually completely absent, and there is often mild hypoplasia of the cerebral white matter that is associated with slight enlargement of the lateral ventricles. (3) A third type of septooptic dysplasia includes pituitary hypoplasia and an ectopic neurohypophysis.28–30
Optic nerve atrophy is an acquired abnormality that occurs in association with various insults. Potential mechanisms of injury include ischemia, trauma, radiation, surgery, infection, inflammatory disease, and neoplasm. Elevated cerebrospinal fluid pressure can cause optic nerve injury and subsequent atrophy. With most of these underlying causes, the atrophy can involve the entire optic nerve or a portion, and may be unilateral or bilateral.
Imaging of optic nerve hypoplasia and atrophy is best achieved with MR. This allows distinction between the optic nerve and the fluid within the optic sheath (Figure 27-10). In some patients, the optic sheath maintains a relatively normal diameter despite the presence of a small optic nerve, and the finding may not be demonstrable on CT. Coexistent intracranial abnormalities, such as absence of the septum pellucidum and hypoplasia of the pituitary gland, are also best evaluated with MR. CT is the imaging technique of choice for those patients who require evaluation of the optic canals.
Figure 27–10
Bilateral optic nerve hypoplasia.
This otherwise healthy 16-month-old boy presented with nystagmus. A, B. Hyperintense fluid in the optic nerve sheaths produces high signal intensity surrounding thin optic nerves on these fat-suppressed T2-weighted MR images. C. A T1-weighted coronal image shows the chiasm to be small as well. There were no associated intracranial anomalies in this child.



There is a broad range of osseous lesions that can affect the orbit. These include developmental bone lesions, acquired metabolic skeletal abnormalities, and neoplastic lesions of the bone. In general, the imaging evaluation of the bony components of the orbits is with a combination of standard radiographs and CT. 3D CT is often useful for characterizing complex lesions. Although MRI also provides information on osseous pathology, its most important function is for evaluating the soft tissue structures within and adjacent to the orbits.
Dysostoses with prominent orbital manifestations include craniofacial dysplasia, the acrocephalosyndactyly syndromes, some forms of craniosynostosis, and mandibulofacial dysplasia. Premature closure of the coronal suture results in a shallow orbit with an oval configuration when viewed on frontal radiographs or 3D CT (see Figure 26-6). Some degree of hypertelorism occurs with bilateral coronal craniosynostosis. Metopic craniosynostosis is associated with hypotelorism, oval configurations of the orbital margins, and upward medial angulation (“quizzical eyes”) (see Figure 26-8). In children with total craniosynostosis, or cloverleaf skull, the orbits are markedly shallow and deformed; there is severe proptosis (see Figure 26-9).
Abnormal thickening of the bony walls of the orbits can occur in patients with osteopetrosis congenita, idiopathic hypercalcemia, fibrous dysplasia (see Figure 28-4), and craniometaphyseal dysplasia. Osseous thickening in these children sometimes causes crowding of intraorbital structures and proptosis. Involvement of the lesser wing of the sphenoid bone can narrow the optic canal(s) and cause impingement of the optic nerve(s). Vascular structures and cranial nerves can also be involved.
Orbital osseous dysplasia is common in individuals with NF-1 (see Figure 27-34). There is often an accompanying orbital plexiform neurofibroma. Patterns of osseous dysplasia in NF-1 include: hypoplasia of the greater wing of the sphenoid (most common), hypoplasia and elevation of the lesser wing of the sphenoid, enlargement of the middle cranial fossa and protrusion into the posterior aspect of the orbit, hypoplasia and deformity of the ipsilateral ethmoid and maxillary sinuses, enlargement of the orbital rim, and enlargement of the superior and inferior orbital fissures. Children with sphenoid wing dysplasia sometimes have pulsatile exophthalmos.31
Orbital abnormalities are common in patients with developmental oblique facial clefts. When the cleft passes through the orbit, microphthalmia or anophthalmia can occur. The size and shape of the orbit are frequently affected. One or more portions of an orbital wall may be absent.
The most common cause of nasolacrimal duct obstruction in the newborn is idiopathic failure of normal perforation of the distal aspect. The imperforate duct often opens spontaneously within a few weeks to months. Persistently obstructed ducts can be opened with probing. Rarely, ductal obstruction is due to an intranasal mass or congenital structural abnormality that compresses the duct orifice or narrows the canal.32,33
Nasolacrimal duct obstruction often results in distention of the lacrimal sac; this is termed a lacrimal sac mucocele or dacryocystocele. A lacrimal sac mucocele appears clinically as a nontender mass just inferior to the medial canthus. There is epiphora. The contents of a lacrimal sac mucocele are sterile at birth, and secondary infection (dacryocystitis or dacryopyocele) is uncommon. Imaging with CT or MR confirms that the clinically visible mass represents a distended nasolacrimal sac. With long-term or prenatal obstruction, the nasolacrimal sac fossa is often enlarged. If there is distal obstruction of the nasolacrimal duct, imaging studies show dilation of the duct and canal.34,35
The most common site of nasolacrimal duct obstruction in the newborn is at the distal orifice in the inferior aspect of the nasal cavity. In about one-quarter of infants with a lacrimal sac mucocele due to distal duct obstruction, the distal portion of the duct becomes distended and forms an endo-nasal cyst under the inferior turbinate; this is termed a nasolacrimal mucocele or endonasal dacryocystocele. This lesion partially obstructs the nasal airway; neonatal respiratory distress is an occasional consequence. Nasolacrimal mucocele is the second most common cause of symptomatic neonatal nasal obstruction, after choanal atresia. Symptoms are most prominent when there are bilateral cysts; this occurs in slightly less than half of patients. Affected infants may exhibit noisy breathing, dyspnea, and feeding difficulty. Cross-sectional imaging studies show a submucosal mass in the nasal cavity (see Figure 29-8 in Chapter 29). The ipsilateral nasolacrimal canal is enlarged and the lacrimal sac is usually dilated as well, resulting in a dacryocystocele (Figure 27-11). Occasionally, a large nasolacrimal mucocele causes distortion of the bony walls of the nasal cavity, and bowing of the nasal septum. The typical treatment of a symptomatic nasolacrimal mucocele is probing or endoscopic marsupialization.36–38
Figure 27–11
Lacrimal sac mucocele and nasolacrimal mucocele.
A. An axial contrast-enhanced CT image of a 4-day-old infant with a medial right orbital mass shows dilation of the lacrimal sac and nasolacrimal sac fossa. B. There is enlargement of the nasolacrimal canal (arrow). C. The dilated nasolacrimal duct (arrow) bulges into the nasal cavity.


Epiphora refers to an overflow of tears. The causes are divided into those associated with excessive lacrimation and those due to obstruction of the drainage apparatus. Congenital obstruction of the nasolacrimal duct is relatively common, as described in the above section on nasolacrimal mucocele. Acquired obstruction can occur due to an inflammatory process, trauma, foreign body, or tumor. The most common site of obstruction is at the junction of the lacrimal sac with the nasolacrimal duct; imaging studies in this situation show distention of the lacrimal sac and a normal caliber duct (Figure 27-12). The second most common site of obstruction is in the common canaliculus, in which case imaging studies are normal.39,40
Figure 27–12
Inflammatory nasolacrimal duct obstruction.
Contrast-enhanced CT of a 4-year-old child with fever and periorbital swelling shows marked distention of the lacrimal sac (arrow). There is also inflammatory thickening of the preseptal soft tissues. Images inferior to this level (not shown) demonstrated a normal nasolacrimal canal.

In children, evaluation of the integrity of the lacrimal drainage apparatus can be easily and painlessly performed with radionuclide dacryocystography. Drops of normal saline that contains radiopharmaceutical are placed in the eyes and continuous pinhole images are obtained. This allows observation of the dynamics of tear drainage through the nasolacrimal apparatus into the nasal cavity and determination of the level of an obstruction. CT and MR are the imaging techniques of choice for the evaluation of fractures, foreign bodies, and tumors that involve the drainage apparatus.41,42
INFECTION/INFLAMMATION
Orbital infection is most often due to extension from an adjacent infected paranasal sinus. Although any paranasal sinus can serve as the source of orbital infection, the ethmoid sinuses are the most common responsible sites in children. An additional important mechanism is periorbital soft tissue infection due to spread from an adjacent source on the face, such as cellulitis following an insect bite. Hematogenous inoculation of the orbital structures from a remote site of infection is uncommon; this mechanism tends to occur more frequently in infants than in older children. Neonates occasionally develop orbital infections due to transmission of organisms from the mother during birth; infection with Neisseria cinerea can be acquired in this manner.43 The most common infectious agents to involve the orbits in the pediatric population included staphylococci, streptococci, and pneumococci. Infections with fungi, viruses, and parasites can also occur.44–46
Soft tissue swelling in the periorbital soft tissues is clinically identifiable in most children with orbital infection. Other potential findings include proptosis, limitation of ocular movements, and ocular/orbital pain. With severe infections, visual compromise can occur. Systemic manifestations, such as fever and leukocytosis, are present in most patients with a bacterial infection. Children with bacterial orbital infection should be carefully evaluated for signs of intracranial spread.47–49
There are 3 pathological manifestations of orbital infection: inflammatory edema, soft tissue infection (cellulitis), and abscess. In addition, infections are characterized according to the specific location and the structures that are involved. The important anatomic orbital divisions are the preseptal soft tissues, the orbit proper, the lacrimal gland, and the bony walls of the orbit. The orbit is further divided into the ocular and retrobulbar spaces, with the latter consisting of the extraconal and intraconal spaces and the extraocular muscles.
Clinically and radiographically evident periorbital soft tissue swelling indicates the presence of either reactive inflammatory edema or true infectious cellulitis. Reactive inflammatory edema (orbital cellulitis in situ) is a secondary inflammatory response to an adjacent infection of the skin, paranasal sinuses, or other structure. The most common pathophysiologic mechanism of reactive inflammatory edema is local venous obstruction caused by elevated pressure within infected paranasal sinuses. Periorbital inflammatory edema can also be caused by an infection in the orbit proper.
Imaging studies and standard clinical investigations do not allow reliable differentiation of periorbital reactive inflammatory edema from infectious cellulitis. The primary utility of diagnostic imaging in these patients is to assess the deeper orbital structures for evidence of cellulitis or abscess, and to evaluate the paranasal sinuses and other potentially infected adjacent structures. Periorbital reactive inflammatory edema and cellulitis are demonstrated on CT and MR as nonspecific thickening and prominent enhancement of the lids and other periorbital soft tissues (Figure 27-13). An abscess at this site is rare.
Figure 27–13
Periorbital cellulitis and ipsilateral maxillary bacterial sinusitis.
A. A contrast-enhanced fat-suppressed MR image shows thickening and hyperenhancement of the right periorbital soft tissues. B. The right maxillary sinus contains fluid and thickened mucosa that are hyperintense on this T2-weighted image. There is expansion of the medial sinus wall. Periorbital edema extends along the lateral aspect of the face. There are no findings of intraorbital inflammation on either image.

The most common manifestation of infection of the orbit proper is edema within the soft tissues, typically in the extraconal fat. As with the periorbital soft tissues, edema within the extraconal or deeper fat can represent either reactive inflammatory edema or true infectious cellulitis. Inflammatory or infectious enlargement of one or more extraocular muscles can also occur, that is, inflammatory myositis. Inflammatory edema most often occurs in the inferomedial aspect of the orbit adjacent to an infected ethmoid or maxillary sinus. CT shows elevated attenuation values of the edematous orbital fat and MR demonstrates high signal intensity on T2-weighted images. Fat-suppressed T2-weighted MR images are most sensitive for detecting edema. Enlargement of the adjacent extraocular muscle(s) is common in children with inflammatory or infectious edema of orbital fat (Figure 27-14). T2-weighted images may demonstrate mildly elevated signal intensity in the involved muscle.50
Figure 27–14
Orbital cellulitis.
Contrast-enhanced coronal CT of a 14-month-old with acute periorbital swelling shows enlargement of the right inferior and medial rectus muscles, that is, inflammatory myositis. There is edema in the adjacent orbital fat, but no periosteal elevation. The paranasal sinuses are opacified.

Subperiosteal abscess is relatively common in pediatric orbital infections, typically occurring adjacent to an infected paranasal sinus. The medial aspect of the orbit adjacent to the ethmoid sinus is the typical site, particularly in young children.51 Superior and inferior subperiosteal abscesses also occur, especially in adolescents and older children with fully developed frontal sinuses. The pathophysiology of a subperiosteal abscess usually involves extension of paranasal sinus infection into the subperiosteal space by way of septic thrombophlebitis of emissary veins. Osseous destruction is not required for this process; in fact, imaging evidence of bone destruction in children with acute orbital infection is rare. The differentiation between inflammatory edema of the extraconal fat and a true subperiosteal fluid collection is usually not possible on unenhanced CT. The key diagnostic imaging feature is the visualization of the elevated periosteal membrane, which is clearly identifiable on technically adequate contrast-enhanced CT and MR images (Figure 27-15). A subperiosteal abscess is frequently best demonstrated on coronal images, particularly when the lesion is located along the superior or inferior wall of the orbit (Figure 27-16).52–54
Figure 27–15
Subperiosteal abscess.
A medial subperiosteal abscess causes displacement of the right medial rectus muscle of a 9-year-old child with a 3-day history of orbital pain and swelling. The elevated and thickened periosteal membrane (arrows) enhances on this CT examination performed with IV contrast.

An abscess within the intraconal space of the orbit is uncommon. Proptosis and visual impairment are often present in patients with an intraconal abscess. The lesion is effectively demonstrated with contrast-enhanced CT or MR. Other manifestations of orbital infection are usually present in addition to the abscess, including edema of the orbital fat and extraocular muscles.
Osteitis or osteomyelitis of the orbital wall can occur in association with orbital infection and/or paranasal sinus infection. Radiographic manifestations of bone involvement do not become apparent until the process is relatively advanced. Apparent bony destruction or thinning of the medial wall of the orbit should be interpreted with caution; this structure is normally quite thin, and visualization is compromised by paranasal sinus opacification. Although only clinically indicated in unusual situations, bone scintigraphy is a sensitive technique for the detection of bone infection in the orbital walls. Typically, bone infection causes abnormal increased tracer accumulation. Occasionally, interruption of the osseous blood supply leads to infarctive osteomyelitis; the infarcted bone fails to accumulate radiopharmaceutical, whereas adjacent inflamed viable bone has increased uptake. The edematous marrow of infected bone is hyperintense on fat-suppressed T2-weighted MR images and, unless infarcted, undergoes prominent contrast enhancement.
Intracranial extension of infection from the orbit or paranasal sinuses can occur by the same mechanism that sinus disease spreads into the orbit, that is, septic thrombophlebitis of emissary veins. Therefore, diagnostic imaging studies of children with orbital infection should be carefully inspected for signs of intracranial infectious involvement. The most common finding is an anterior epidural abscess adjacent to an infected frontal sinus. Other potential intracranial complications of orbital or paranasal sinus infection include subdural abscess, meningitis, cerebritis, brain abscess, and cavernous sinus thrombosis (see Chapter 28). These complications are effectively detected with CT or MR; the use of IV contrast is generally indicated in this situation. Bone destruction is an occasional finding, sometimes accompanied by pneumocephalus. Septic thrombophlebitis of the superior ophthalmic vein is a potential complication of orbital cellulitis that is demonstrable with MR or contrast-enhanced CT.55–57
Tuberculosis of the orbit is rare. The predominant route of orbital infection is dissemination from a pulmonary source or contiguous spread from an adjacent head and neck structure such as the sinonasal cavities. Orbital tuberculosis can present with proptosis, a “cold abscess,” periorbital soft tissue inflammation, keratitis, ectropion, or dacryoadenitis. Periostitis is a relatively common manifestation of orbital tuberculosis, particularly in children and young adults; this typically involves the outer margin of the orbit. The clinical manifestations of orbital tuberculosis tend to indicate a slowly progressive process. Typically, the infection is unilateral. Orbital tuberculosis occasionally occurs in the absence of a clinically or radiographically demonstrable systemic focus. Some patients have imaging and clinical findings of preseptal cellulitis, and the diagnosis of tuberculosis is first suggested by a failure of response to standard antibiotic therapy.58–60
Endophthalmitis is an infection of the globe. This occurs due to direct inoculation of the globe (e.g., penetrating trauma or surgery), spread from an adjacent structure, or hematogenous inoculation (uncommon). Endophthalmitis is a very serious ocular infection, and carries a poor visual prognoses. The clinical manifestations include blurred vision, redness, pain, conjunctival hyperemia, lid edema, chemosis, corneal edema, reduced red reflex, and an afferent pupillary defect.61,62
CT and MR of patients with endophthalmitis demonstrate scleral-uveal thickening and prominent contrast enhancement. The attenuation values and signal characteristics of the vitreous are frequently altered due to edema, pus, or hemorrhage. With penetrating trauma, imaging studies also serve to detect an intraocular foreign body or disruption of the posterior margin of the globe.63,64
Rarely, chronic granulomatous endophthalmitis due to tuberculosis can present with leukocoria and a vitreous mass that can simulate retinoblastoma. In some instances, the granuloma is calcified, further complicating the imaging differentiation from retinoblastoma.65
Infection with the dog roundworm Toxocara canis has 2 forms of clinical expression: visceral and ocular. Ocular infection is initiated by ingestion of Toxocara eggs. A larva subsequently migrates to the eye, where it dies. The resultant inflammatory granulomatous reaction (sclerosing endophthalmitis) causes opacification of the vitreous and retinal detachment. Hemorrhage is common in the retinal, subretinal, and vitreous spaces. The anterior segment is usually uninvolved. This infection most commonly occurs in young children who are in close contact with dogs, particularly puppies.
The diagnostic imaging findings of T canis endophthalmitis are variable. CT may show diffuse homogeneous increased attenuation within the vitreous due to subretinal exudates and organized vitreous. A discrete soft tissue attenuation mass with slight contrast enhancement can also be present, representing a granuloma. The proteinaceous exudate of T canis endophthalmitis has high signal intensity on both T1-weighted and T2-weighted MR images. With long-standing disease, there may be microphthalmos. A lack of ocular calcification in patients with T canis endophthalmitis aids in the differentiation from retinoblastoma; however, small irregular calcifications sometimes eventually develop with this entity.66–68
The eye is an important potential site of infection with Toxoplasma gondii. Eye infection can occur due to prenatal exposure. In older patients, ocular toxoplasmosis is nearly always associated with systemic immunocompromise, such as HIV infection. Single or multifocal retinal lesions occur, sometimes associated with large areas of retinal necrosis. The lesions are detectable with ophthalmoscopy. Cross-sectional imaging studies sometimes show manifestations of inflammation in the globe or retrobulbar soft tissues. The most common findings in infants with congenital toxoplasmosis of the eye are chorioretinitis and microphthalmia. Concomitant CNS lesions are present in most patients with congenital or acquired toxoplasmosis. Toxoplasmosis is the most frequent cause of posterior uveitis in childhood.69,70
Cytomegalovirus (CMV) chorioretinitis can occur in infants as a congenital infection, usually concomitant to encephalitis. Chorioretinitis is an exudative inflammatory process that involves the retinal vessels. Toxoplasmosis and CMV are the most common causes of neonatal chorioretinitis. CMV can lead to a rapidly progressive necrotic retinitis in immunocompromised patients of all ages, such as those with HIV infection or medical immunosuppression for transplantation. The imaging findings of CMV chorioretinitis are identical to those of toxoplasmosis: microphthalmia, uveal thickening and edema, and prominent uveal enhancement.
Orbital hydatid cyst is a rare complication of echinococcosis. However, this infection is an important cause of orbital pathology in highly endemic regions of the world. Orbital hydatid cysts are much more common in children than in adults. Common clinical findings include proptosis, visual disturbance, papilledema, and eyelid edema. Imaging studies usually show a unilocular intraorbital cyst. Multiple cysts are present in less than 5% of patients. Most often, the cyst is in the orbit proper. An intraocular location can also occur, sometimes subretinal.71–73
Dacryoadenitis refers to inflammation of the lacrimal gland. This can occur as an isolated process, as part of a larger inflammatory condition of the orbit, or as a component of a systemic disease. In the pediatric age group, lacrimal gland inflammation most often occurs as part of a postviral syndrome. Infectious etiologies of acute dacryoadenitis include mumps, infectious mononucleosis, bacterial infection, and fungal infection. Clinically, there is fullness and soft tissue swelling along the lateral upper margin of the orbit. The globe may be displaced.74,75
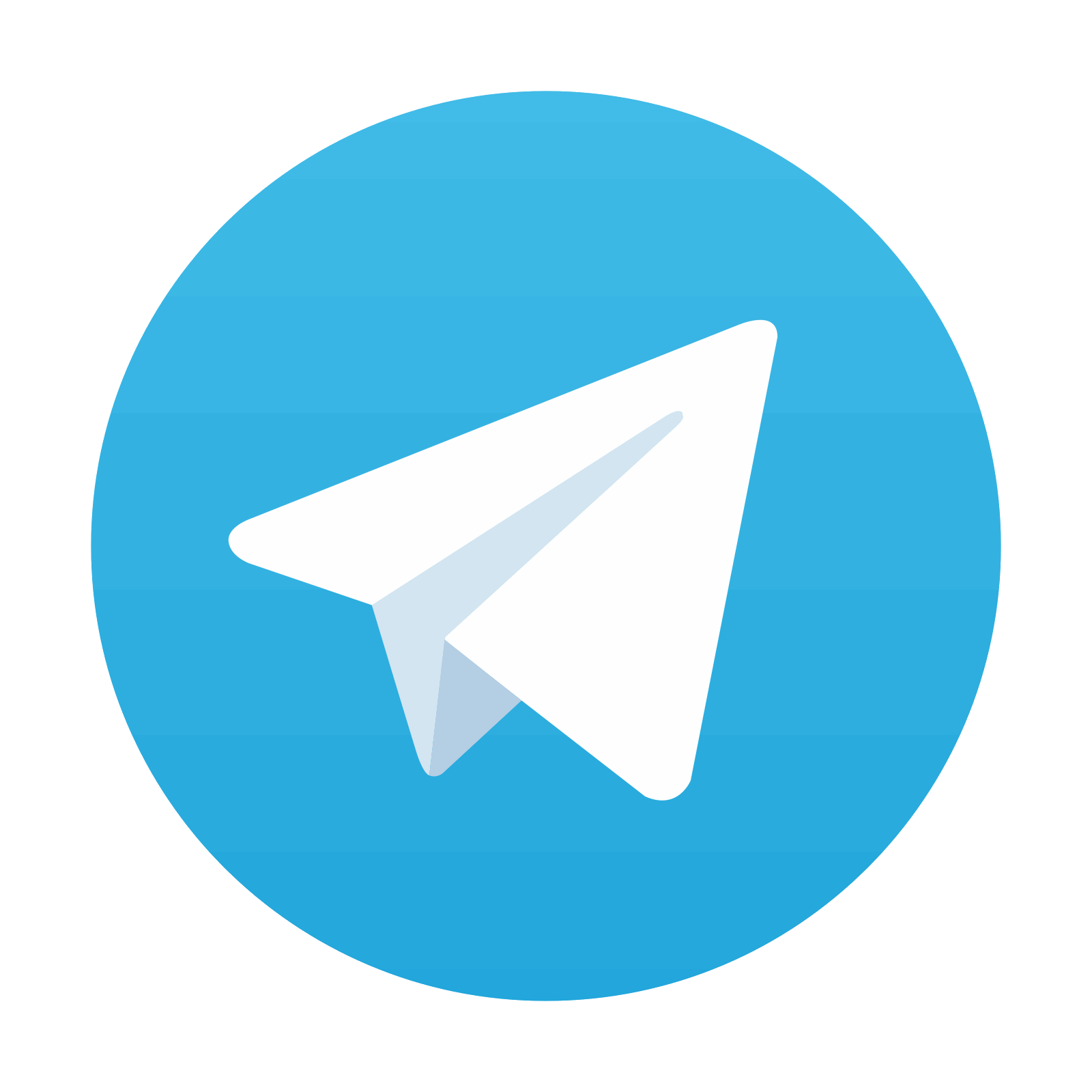
Stay updated, free articles. Join our Telegram channel

Full access? Get Clinical Tree
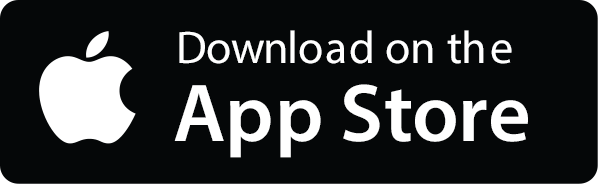
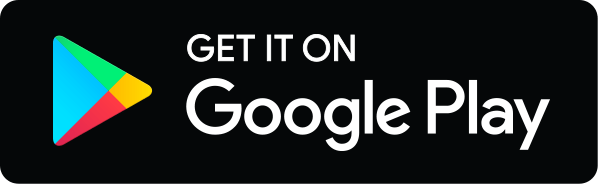